- 1Nearshore Ecology, Hakai Institute, Heriot Bay, BC, Canada
- 2Fisheries Ecology and Conservation Lab, Department of Biology, University of Victoria, Victoria, BC, Canada
- 3Institute for the Oceans and Fisheries, University of British Columbia, Vancouver, BC, Canada
In seagrass food webs, small invertebrate mesograzers often exert top-down control on algal epiphytes growing on seagrass blades, which in turn releases the seagrass from competition for light and nutrients. Yet, nearshore habitat boundaries are permeable, and allochthonous subsidies can provide alternative food sources to in-situ production in seagrass meadows, which may in turn alter mesograzer-epiphyte interactions. We examined the contribution of allochthonous kelp (Nereocystis luetkeana), autochthonous epiphytic macroalgal (Smithora naiadum), Ulva lactuca, and seagrass production to mesograzer diets in a subtidal Zostera marina (eelgrass) meadow. In both choice feeding experiments and isotopic analysis, mesograzer diets revealed a preference for allochthonous N. luetkeana over Z. marina, S. naiadum, and U. lactuca. Notably, Idotea resecata showed an ~20x greater consumption rate for N. luetkeana in feeding experiments over other macrophytes. In the meadow, we found a positive relationship between epiphytic S. naiadum and gammarid amphipod biomass suggesting weak top-down control on the S. naiadum biomass. Epiphyte biomass may be driven by bottom-up factors such as environmental conditions, or the availability and preference of allochthonous kelp, though further work is needed to disentangle these interactions. Additionally, we found that gammarid and caprellid amphipod biomass were positively influenced by adjacency to kelp at seagrass meadow edges. Our findings suggest that N. luetkeana kelp subsidies are important to the diets of mesograzers in Z. marina meadows. Spatial planning and management of marine areas should consider trophic linkages between kelp and eelgrass habitats as a critical seascape feature if the goal is to conserve nearshore food web structure and function.
1 Introduction
The recognition that ecosystems are connected across boundaries by nutrient and organism flow has broadened our understanding of trophic interactions within and among ecosystems (Loreau et al., 2003; Marczak et al., 2007). Energy that cross ecosystem boundaries - known as allochthonous subsidies - can play key roles in population and community structure of recipient habitats, and in turn, the function and stability of these ecosystems (Polis and Hurd, 1996; Huxel et al., 2002). The importance of allochthonous subsidies is now recognized in nearly all aquatic and terrestrial ecosystems (Lafage et al., 2019), from tropical and temperate rainforest insect communities (Recalde et al., 2016; Recalde et al., 2020; Nakano and Murakami, 2001) to freshwater planktonic communities (Vargas et al., 2011, Adamczuk et al., 2019), subtropical island ecosystems (Spiller et al., 2010) and coastal marine ecosystems (Savage, 2019; Zuercher and Galloway, 2019). While allochthonous subsidies are often overlooked when assessing ecosystem interactions (Buckner et al., 2018; Smale et al., 2018), they are increasingly recognized as having important influences on the composition of food web producers, as well as trophic transfer through food webs.
The availability of allochthonous foods can alter interactions between primary producers and consumers in the recipient ecosystem (Huxel and McCann, 1998; Huxel et al., 2002). For instance, if allochthonous inputs weaken specific autochthonous producer - consumer interactions, then expected trophic cascades could be dampened (Polis and Hurd, 1996; Huxel and McCann, 1998; Rodewald et al., 2011). The influence of an allochthonous subsidy may depend on the characteristics of the trophic subsidy itself (e.g. its duration, timing, spatial extent, palatability) as well as characteristics regulating consumers in the recipient habitat, such as availability of other food sources, consumer size, feeding mode, trophic level and life history stage (Zuercher and Galloway, 2019). Previous work has largely focused on the effect of cross-ecosystem subsidies on nutrient-poor recipient ecosystems such as desert islands (Anderson and Polis, 1999) and sandy beaches (Lastra et al., 2008; Liebowitz et al., 2016), as well as aquatic subsidies into riparian ecosystems (Hocking and Reimchen, 2009; Lafage et al., 2019). Growing evidence suggests cross-ecosystem energy transfer may be important to highly productive recipient ecosystems, such as mangroves (Slim et al., 1996), coral reefs (Carreón-Palau et al., 2013) and seagrass meadows (Hyndes et al., 2012, Cartraud et al., 2021).
In seagrass ecosystems, a central tenet of food web structuring is the top-down role of small invertebrate herbivores (herein ‘mesograzers’) in consuming algal epiphytes, which releases seagrass from negative impacts of algal shading and/or nutrient competition and maintains a seagrass-dominated ecosystem (Orth and Van Montfrans, 1984; Valentine and Duffy, 2006; Cook et al., 2011). Empirical evidence for this indirect positive effect of mesograzers on seagrass productivity has been demonstrated thoroughly (Orth and Van Montfrans, 1984; Hughes et al., 2004; Moksnes et al., 2008; Whalen et al., 2013) and is increasingly important to our understanding of bottom-up and top-down human disturbances to seagrass meadows (e.g., eutrophication and overfishing, respectively). Although negative relationships between mesograzers and epiphytes are widely generalized, their strength and direction can depend on seasonality (Whalen et al., 2013), the source of nutrient inputs (Hessing-Lewis and Hacker, 2013), mesograzer species composition (Duffy and Harvilicz, 2001; Jaschinski and Sommer, 2008), and predation rates on mesograzers (Moksnes et al., 2008; Hughes et al., 2013).
Seagrass habitats have high in-situ productivity and are known to contribute significant amounts of biomass to adjacent ecosystems such as the deep sea and sandy beaches (Heck et al., 2008; Liebowitz et al., 2016; Duarte and Krause-Jensen, 2017). Yet, their role as recipients of allochthonous materials has received relatively less attention. Seagrass meadows often occur in sheltered coastal environments, where their canopies facilitate the deposition of materials from the water column, leading to enhanced accumulation of allochthonous materials (Peterson et al., 2004; Hendriks et al., 2008). Among many potential allochthonous inputs, the role of kelp is emerging as an important food source for organisms in temperate seagrass meadows (Smit et al., 2006; Hyndes et al., 2012; Hyndes et al., 2014; Olson et al., 2019). For example, along the Atlantic coast of Canada, an estimated 82% of annual kelp productivity enters detrital pathways, which can enhance secondary production in recipient food webs (Krumhansl and Scheibling, 2012). Accumulation of kelp biomass in seagrass meadows can be substantial with transportation to meadows occurring from considerable distances away (Wernberg et al., 2006). However, the extent to which kelp may be incorporated into recipient seagrass food webs, and if they can alter mesograzer-producer interactions, remains unclear.
In this study, we examined the contribution of allochthonous bull kelp (Nereocystis luetkeana) to the diets of mesograzers in a temperate seagrass Zostera marina (common name ‘eelgrass’) relative to other ubiquitous macrophytes. We quantified the preference for allochthonous vs. autochthonous sources to mesograzers using choice feeding experiments. Next, we assessed the contributions of these same macrophytes to in-situ mesograzer diets using natural isotopic tracers. Because of the widespread presence of kelp forests in this seascape, and their high nutrient quality, we expected kelp subsidies to make up a significant proportion of mesograzer diets. Finally, kelp subsidies may mediate mesograzer – macrophyte interactions. As a first step in assessing the trophic influence of kelp on the Z. marina food web (see Hessing-Lewis et al., 2018), we examined relationships between common mesograzers and a dominant Z. marina epiphyte (Smithora naiadum), as well as the effect of meadow location on mesograzer biomass.
2 Methods
British Columbia’s (B.C.) coastline is characterized by high complexity, including exposed outer-coast islands, sheltered bays, estuaries, and steep fjords. On the central coast of B.C., the nearshore environment is a heterogenous seascape consisting of kelp forests, seagrass meadows, benthic algae, rocky reefs, and sandy habitats. Our study was conducted in a large subtidal Z. marina meadow located in Choked Passage on the northern shore of Calvert Island in the summer of 2015 (Figure 1). This Z. marina meadow is predominantly surrounded by shallow rocky reefs, bare sand habitats, annual N. luetkeana kelp forests, and to a lesser extent perennial Macrocystis pyrifera kelp forests.
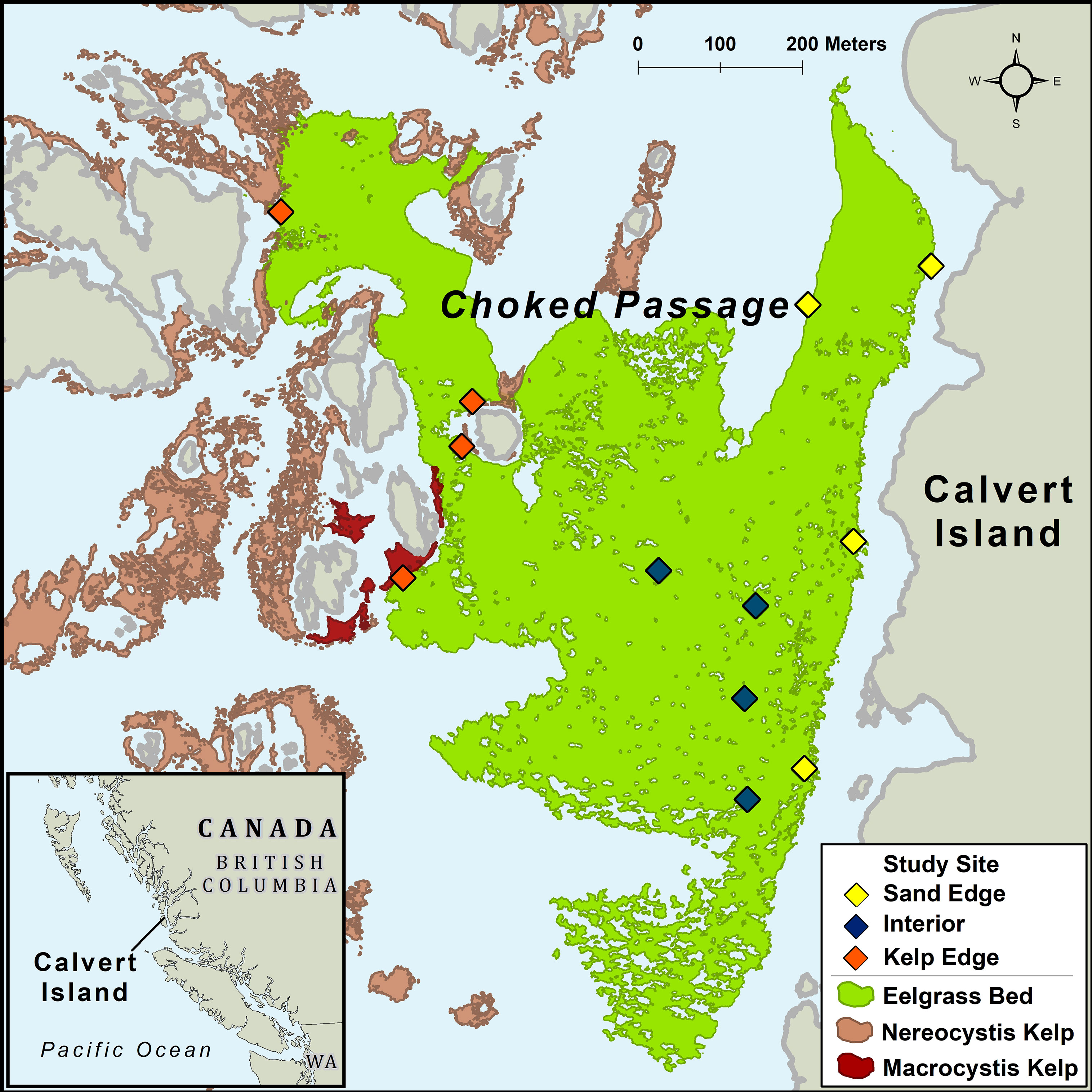
Figure 1 Study area off Calvert Island, British Columbia, Canada. Sites were established in a Zostera marina meadow in Choked Passage (green) which is located in a nearshore seascape surrounded by canopy forming kelp, primarily Nereocystis luetkeana (light red) in proximity to some Macrocystis pyrifera (dark red).
2.1 Choice feeding experiments
In August 2015, we conducted a multiple-choice feeding experiment with mesograzers collected from the Choked Passage meadow to determine their dietary preference among allochthonous (N. luetkeana) and autochthonous (Z. marina, S. naiadum) macrophytes, as well as Ulva lactuca (both allochthonous and autochthonous). We focused on mesograzers with larger body size because of their ubiquity in local Z. marina meadows and adjacent kelp forests and ease to work with. Mesograzers and macrophytes were collected haphazardly from the meadow, focusing on isopods, I. resecata, (mean 23 ± 4 mm length (SD) and 116.3 ± 33.6 mg biomass in our study) and cryptic kelp crabs, Pugettia richii, a larger body size grazer (max. size 44 mm, Lamb and Hanby, 2005) averaging 5962.9 ± 4421.9 mg in our study.
The experiment took place in a natural flow-through seawater system. Mesograzers were starved for 35 hours prior to the feeding experiments, weighed, and placed in separate small containers with fine mesh windows open to seawater off the Hakai Institute Observatory dock in Pruth Bay directly adjacent to another Z. marina meadow. For the experiment, mesograzers were placed in an experimental container (946 mL volume) which included four macrophytes of equal surface area (2 x 2 cm square): Z. marina, N. luetkeana, S. naiadum and U. lactuca. The containers were suspended off the dock and subject to natural daylight patterns and temperatures (Supplementary Figure 1). Replicate trials were conducted for each mesograzer type along with simultaneous control trials with no mesograzers present (n = 7). Experimental containers were otherwise bare (e.g., no sand or habitat substitutes). Macrophytes were blotted dry and weighed on a microbalance (mg) before and after the duration of the feeding trials, which were run for 18 hours. Macrophyte consumption rates by each mesograzer were calculated using Equation 1 (Taylor and Brown, 2006; Sampaio et al., 2017), which accounted for consumer size (i.e., biomass in mg) and the change in the weight of the macrophyte relative to a grazer-free control:
where Ti is the initial producer blotted wet weight (bww), Tf is the final bww, Ci is the initial control bww, Cf is the final control bww, nbio is the grazer biomass (i.e., size) at the end of the experiment (g), and t = duration of the experiment in days. Consumption rate is thus expressed in mg of macrophyte consumed per mesograzer biomass per day (mg PP/mg grazer/day). A one-way analysis of variance (ANOVA) was used to test for differences in the consumption rates for both I. resecata and P. richii on the four macrophytes. If significant differences were detected (P< 0.05), a Tukey’s post-hoc test was used to examine pairwise comparisons amongst all macrophytes used in the feeding trials.
2.2 Stable isotopes and mixing models
We collected mesograzers from Z. marina shoots during a 2-week period in late July- early August 2015 from the kelp edge, sand edge, and interior sites. Mesograzers were frozen until laboratory processing for isotope analysis. Due to their small body sizes, numerous individuals of gammarid amphipods (multiple species in the family Gammaridae) and Lacuna snails (multiple species of the Lacuna genus,) were pooled within a single sample (n = 10 individuals/sample, 5 samples total). Other mesograzers were large enough that an individual’s biomass filled a sample: Caprellid amphipods (multiple species of the Caprellidae family, n = 13), I. resecata (n = 13), and P. richii (n = 3). Isotope signatures of most macrophytes were obtained from a concurrent study (see Olson et al., 2019), which included Z. marina and S. naiadum as in-situ meadow production and N. luetkeana from surrounding kelp forests. For this study, U. lactuca (n = 20) was also collected adrift in the Z. marina meadow. Small amounts of U. lactuca were found growing in the seagrass meadow and also was commonly found growing in the surrounding reef or sand habitats rather than within the meadow (authors’ personal obs.), and thus could be considered as both autochthonous and/or allochthonous production.
Samples were prepared for isotope analysis by defrosting and removing surface debris. Lacuna snail bodies were pulled out of their shells for processing. Whole bodies of the other mesograzers (including stomachs) were rinsed in two baths of deionized water and dried at 60°C. δ13C and δ15N were analyzed at the Mazumder Lab at the University of Victoria on a Delta IV Isotope Ratio Mass Spectrometer, as the ratio of heavy to light isotope with values denoted in δ:
where R represents the ratios 13C/12C and 15N/14N of the sample or laboratory standard. Mass ratios of carbon to nitrogen (C:N) were also determined for macrophytes, and were used to assess their relative palatability, where lower values of C:N represent relatively higher nutritional content. An ANOVA test was used to assess differences in the C:N among macrophytes, with a Tukey’s post-hoc test to further contrast between each combination.
We used a Bayesian isotopic mixing model mixSIAR (Moore and Semmens, 2008, Stock et al., 2018) to assess the relative contribution of the macrophytes to mesograzer diets. When predators consume prey energy, the heavy isotope is favoured over the light isotope due to discrimination from metabolic processes, which causes an enrichment of isotope values with trophic level. Thus diet–tissue discrimination factors (DTDFs) are used when estimating prey contribution to a predator’s diet. Because mixing model results are highly sensitive to DTDFs, we assessed two options from the literature: 0.4 ± 0.12‰ for δ13C and 2.0 ± 0.20‰ for δ15N (McCutchan et al., 2003) and 0.4 ± 1.14‰ for δ13C and 3.4 ± 1.0‰ for δ15N (Post, 2002). After visual assessment of the consumer isotopic values alongside macrophyte values post correction (Phillips et al., 2014), DTDFs from Post et al. (2002) were chosen because of their better fit (i.e., consumer values fell within the range of macrophyte mean and standard deviation values). During this analysis, one caprellid amphipod outlier with depleted δ13C values outside the macrophyte isotope ranges was removed.
2.3 Establishing mesograzer-epiphyte relationships
To assess biomass and abundance of macrophytes and mesograzers, twelve sites were established in the Z. marina meadow (total area ~367,000m2; Figure 1). 40 m transects were set in the Z. marina meadow adjacent to N. luetkeana kelp forests (n=4 transects), adjacent to sand habitats (n=4), and in the interior of the meadow (n=4) at depths that ranged from 1.52m – 4.99m. We collected Z. marina shoots at 10 m intervals (n = 5 shoots per transect) by scuba. Divers carefully covered shoots with a plastic bag, detached the shoot from the rhizome, and sealed the bag before moving to the next collection point. Sampling occurred in May, July, and August 2015. Shoot samples were subsequently processed in the laboratory. Bag contents were sieved through a 500um filter, capturing mesograzers > 500um. Z. marina shoots were gently scraped of epiphytes and mesograzers. All components were oven dried at 60°C for biomass measurements.
We used a binomial - gamma hurdle generalized linear model (GLM) to assess the relationship between mesograzer biomass and S. naiadum biomass in R (R Core Team, 2021). Mesograzers and S. naiadum dry biomass (g) were standardized by dry Z. marina blade biomass (g) from which they were collected. We first modeled the probability of presence or absence of each mesograzer’s biomass related to S. naiadum biomass with a binomial distribution. A gamma model was then used on non-zero grazer biomass to estimate the relationship between grazers and S. naiadum biomass. If residual plots indicated high leverage points in preliminary model analysis, these data were removed by a Cook’s Distance cut off.
3 Results
3.1 Feeding experiment results: Mesograzer preferences
I. resecata and P. richii consumed all four macrophytes provided to them (N. luetkeana, U. lactuca, Z. marina and S. naiadum) to some extent. Control replicates (no grazers present) showed little loss of biomass and any changes that did occur were accounted for in consumption rate calculations (Equation 1). Preferences for macroalgae over Z. marina were demonstrated by both mesograzers, as well as a notably high consumption rate of N. luetkeana kelp by I. resecata isopods - 0.8 mg kelp/mg grazer/day (Figure 2A).
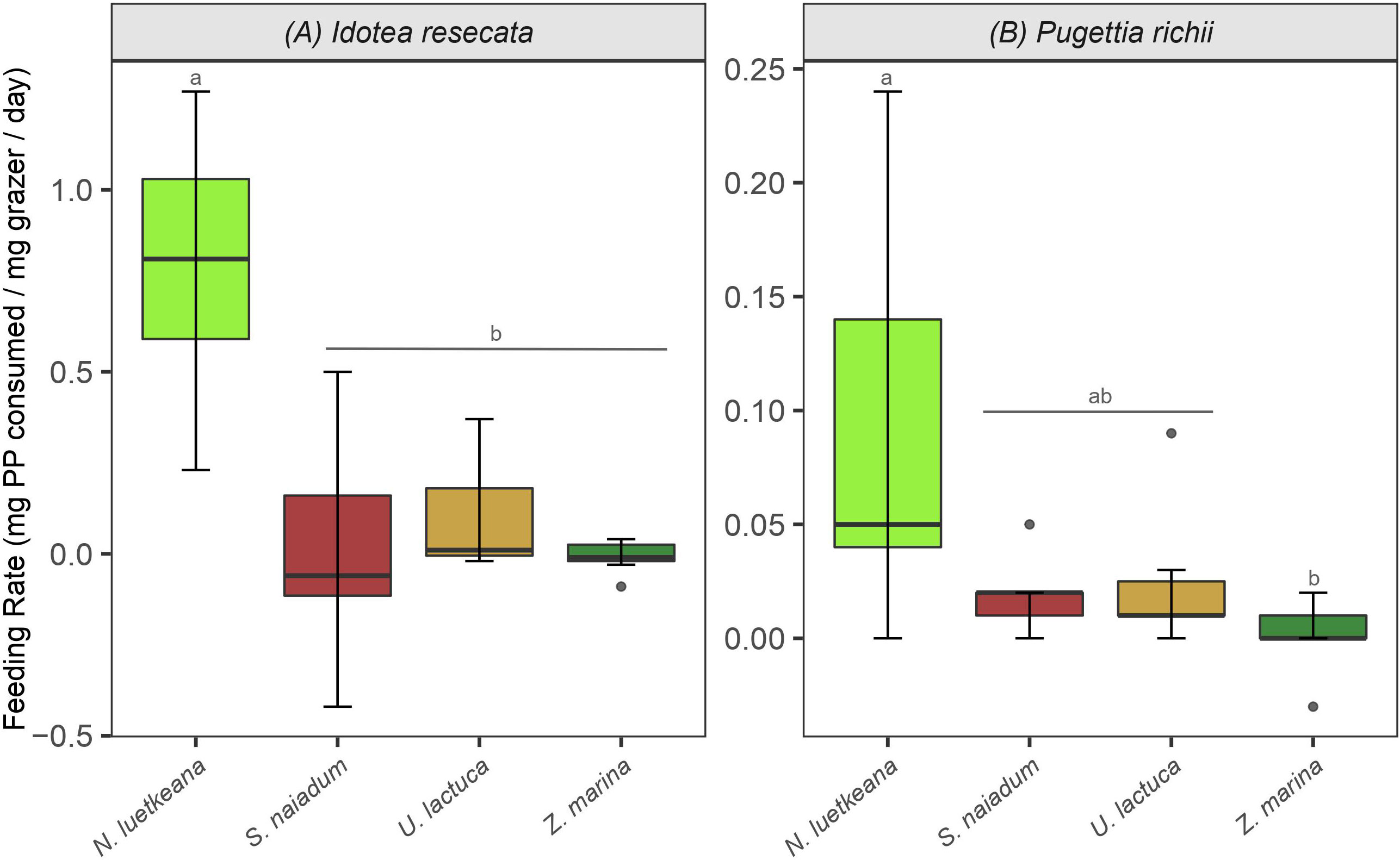
Figure 2 Consumption rates (mean and standard error) by (A) I. resecata isopods and (B) P. richii crabs on macrophytes from the feeding trials - N. luetkeana, S. naiadum, U. lactuca and Z. marina. Note the differing scales for each mesograzer. Letters indicate treatments that are significantly different from one another. The figure represents the full and raw dataset, and negative values derived from the consumption equation were retained for completeness.
I. resecata consumed the four macrophytes at different rates (Figure 2A, ANOVA: F(3, 24) = 17.21, P< 0.001). Specifically, they consumed N. luetkeana at a greater rate than U. lactuca, S. naiadum and Z. marina (P< 0.001 for all pairwise comparisons); consumption rates were ~20x higher for N. luetkeana relative to the other three options. Further, there was no difference in their consumption rate of each combination of S. naiadum, U. lactuca and Z. marina (Figure 2A). Similar to I. resecata, P. richii consumed macrophytes at different rates (Figure 2B, ANOVA: F(3, 24) = 4.271, P = 0.015). The largest difference in consumption rate by P. richii was observed between N. luetkeana and Z. marina (P = 0.014). No other pairwise comparisons of consumption rates for P. richii were significantly different (Figure 2B).
3.2 Isotopic results and feeding observations from the field
The isotopic composition of mesograzer in the Z. marina meadow was variable particularly in δ13C (Figure 3). Caprellid amphipods had the most depleted δ13C signatures, while Lacuna snails were most enriched. δ15N signatures of the mesograzers were much closer in range, where gammarid amphipods and P. richii were more enriched relative to I. resecata, caprellid amphipods, and Lacuna snails (Figure 3). δ13C and δ15N values of U. lactuca fell in between Z. marina (most enriched) and S. naiadum (most depleted). N. luetkeana, S. naiadum, and U. lactuca macroalgae had lower C:N ratios than Z. marina (pairwise comparisons, P<0.001). Macrophytes differed in palatibility as measured by C:N ratios (Table 1, ANOVA: F(3,63) = 158.1, P< 0.001). S. naiadum had the lowest C:N ratios of all macrophytes examined (P<0.05), specifically ~ 3x lower than the other autochthonous macrophyte, Z. marina. We found no difference between N. luetkeana and U. lactuca C:N ratios (P = 0.29).
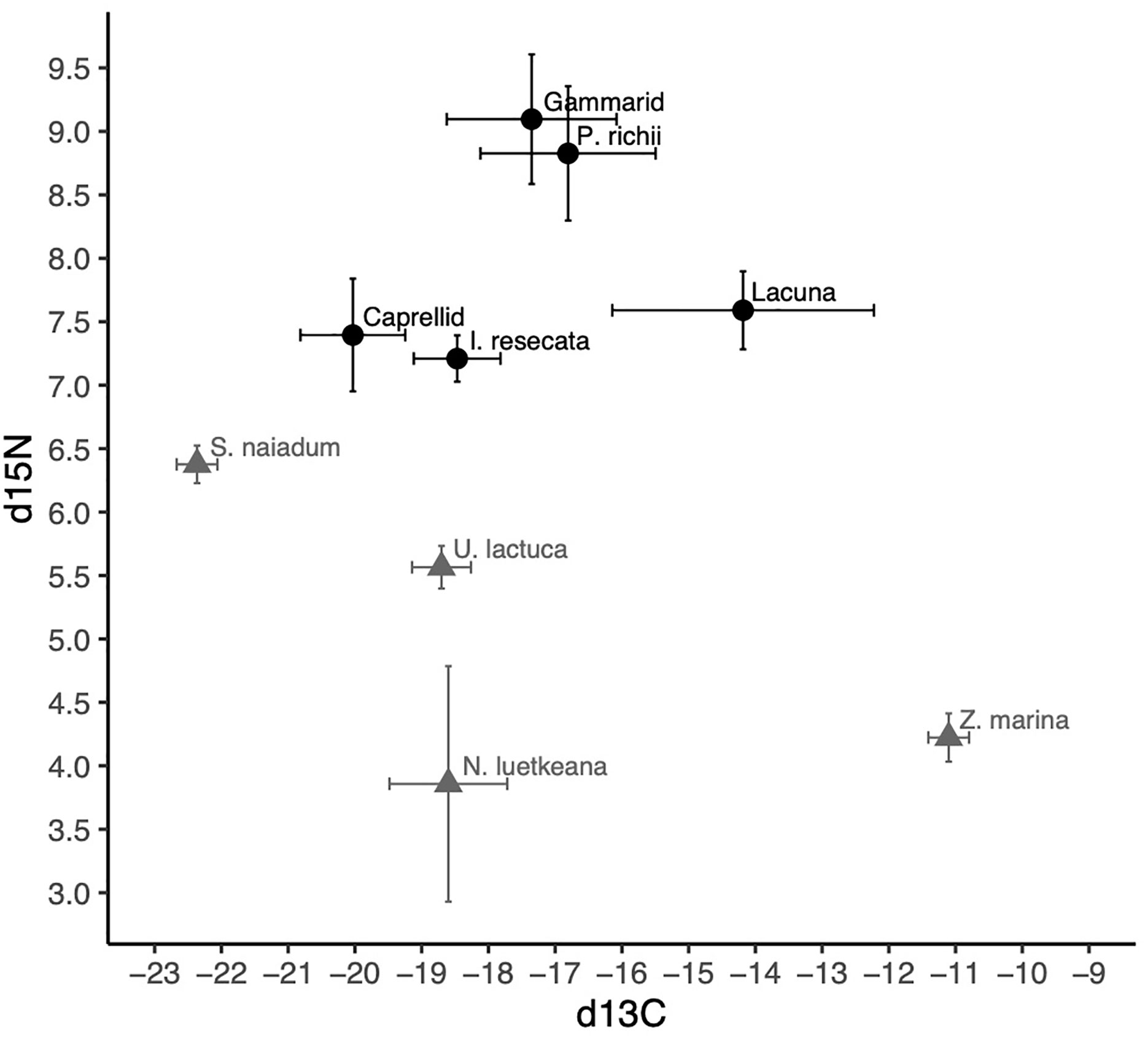
Figure 3 δ13C and δ15N signatures (mean and standard deviation) of invertebrate mesograzers (black) and macrophytes (grey) in the Choked Pass Z. marina meadow.

Table 1 Macrophyte carbon to nitrogen ratios (C:N) in the Z. marina meadow indicating their relative palatability.
Contributions of allochthonous vs. autochthonous macrophytes to diets varied by mesograzer (Figure 4). Allochthonous sources were high in I. resecata and caprellid amphipods, whereas autochthonous sources contributed more to P. richii, gammarid amphipod, and Lacuna snail diets. In I. resecata diets, N. luetkeana had the highest contribution (54.3%, Figure 4A), followed by U. lactuca (17.6%) and S. naiadum (16.3%). Z. marina had the lowest contribution to I. resecata diets (12%). For caprellid amphipod diets (Figure 4B), S. naiadum (35.2%) and N. luetkeana (33.6%) showed the highest contributions, whereas U. lactuca (21.3%) and Z. marina (10.4%) had low dietary contributions.
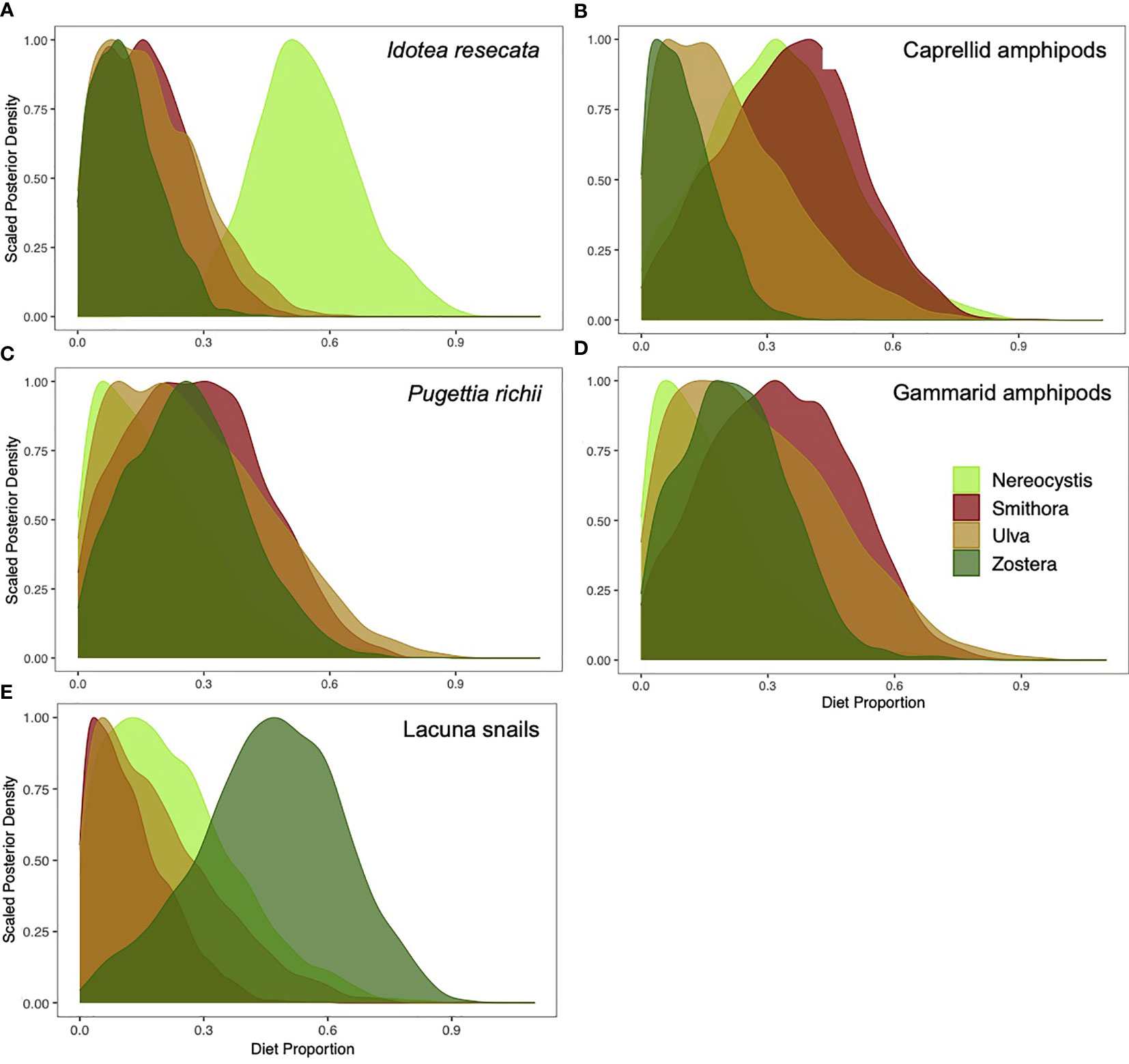
Figure 4 Proportion of N. luetkeana, S. naiadum, U. lactuca, and Z. marina that contributed to mesograzers diets: (A) I. resecata, (B) caprellid amphipods (C) P. richii crabs, (D) gammarid amphipods, and (E) Lacuna snails.
Autochthonous meadow sources dominated P. richii diets: S. naiadum (28.1%) and Z. marina (26.1%) (Figure 4C). Contributions from U. lactuca (27.4%) were also high, while contributions from N. luetkeana were lowest (18.4%). Similarly, gammarid amphipods diets (Figure 4D) had highest contributions from S. naiadum (32.7%) followed by U. lactuca (28%), Z. marina (22.5%) and the lowest by N. luetkeana (16.7%). Lacuna snail diets consisted primarily of Z. marina (46%), with lower contributions of N. luetkeana (22.6%), U. lactuca (19.1%), and S. naiadum (12.4%).
The relative uptake of primary production by mesograzers did not consistently mirror their relative palatability as assessed by C:N ratio (Table 1, Figure 4). Caprellid amphipods were the only mesograzer that closely matched their diets to palatability via C:N ratio. S. naiadum was favoured by most mesograzers (Figures 4B–D). N. luetkeana contributed more than expected (based on C:N) to I. resecata and Caprellids amphipods. Z. marina also had higher than expected contributions, as seen in Lacuna snails and P. richii crabs (Figures 4E, C, respectively).
3.3 Field observations of the grazer-producer biomass relationship
The sub-tidal Z. marina shoots weighed on average 2.3 ± 0.95 g (SD, dry weight, n = 178), and were characterized as long (146.5 ± 36.0 cm) and wide (0.85 ± 0.15 cm) from n = 115 intact longest blades. The dominant epiphyte across the meadow was the red alga S. naiadum. Lobed blades of S. naiadum growing from encrusted basal cushions were extensive across the meadow: present on 66% of the blades with an average biomass of 0.82 ± 1.1 g shoot-1 up to a maximum value of 5.1 g shoot-1. Punctaria spp. and Ulva spp. epiphytes were present but less abundant - when present, they had minimal biomass on blades (mean of 0.20 ± 0.16 g shoot-1 and 0.07 ± 0.05 g shoot-1, respectively).
Results from the gamma hurdle model indicate that gammarid amphipods and S. naiadum biomass had a positive relationship (GLM intercept = -6.30 ± 0.25; b = 2.21 ± 0.28, P<0.001) in the eelgrass meadow (Figure 5A). The other mesograzers examined did not demonstrate a significant relationship with S. naiadum (Supplementary Figure 2). The biomass distribution of mesograzers was uneven across the meadow (Figures 5B–D). Gammarid and caprellid amphipods had significantly higher biomass at the kelp edge relative to the sand edges and interior sites (GLM b = 1.63 ± 0.35, P<0.001, b = 3.35 ± 1.11, P =0.004, respectively). In contrast, I. resecata biomass was significantly lower at kelp edges than the interior and sand sites (P = 0.020). Lacuna snail biomass was consistent throughout the meadow (Supplementary Figure 2D).
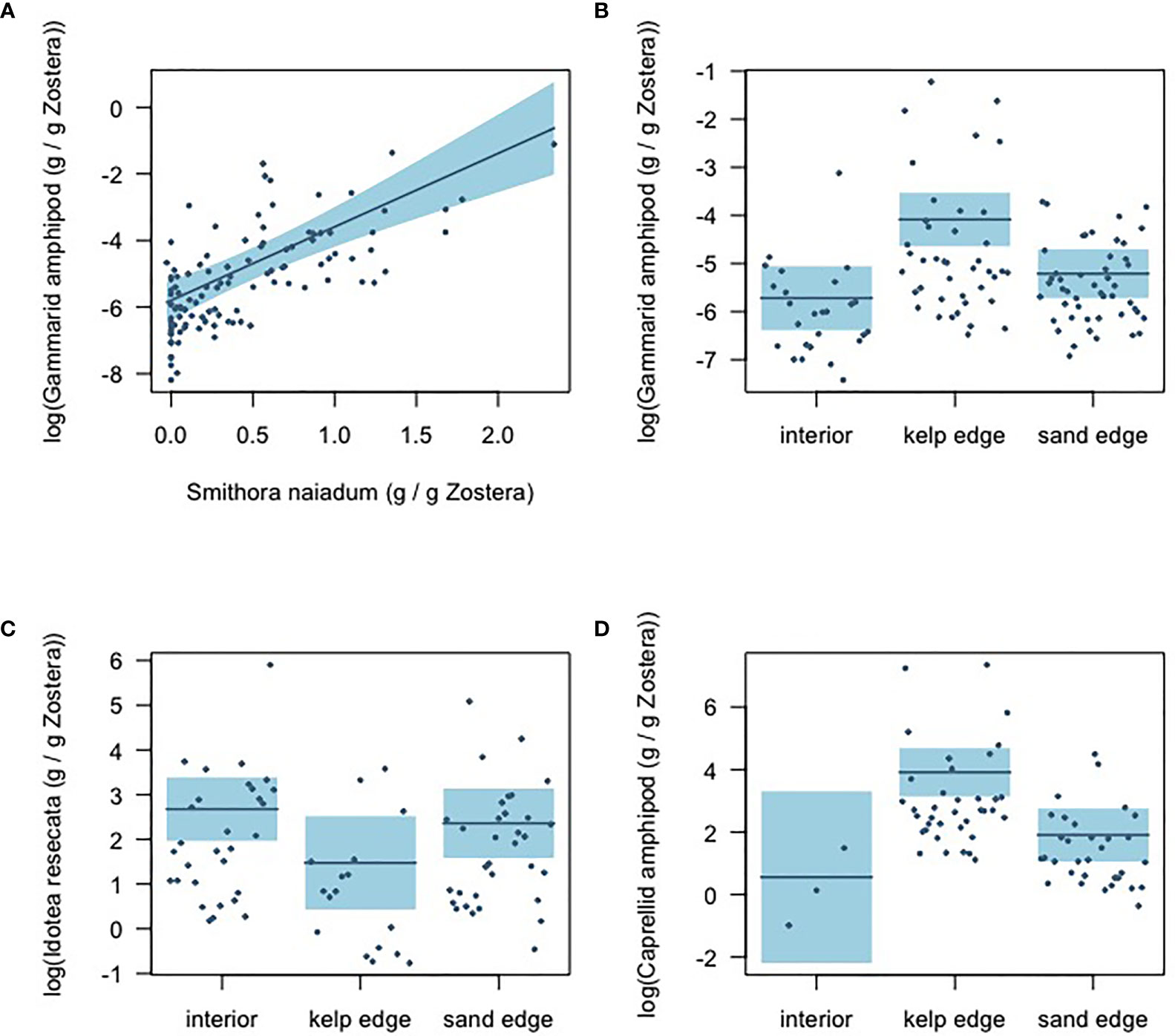
Figure 5 Gamma-hurdle model results showing the (A) relationship between S. naiadum epiphyte biomass and gammarid amphipod biomass (standardized by Z. marina biomass); and the mesograzer biomass distributions across the meadow sites: (B) gammarid amphipods (C) I. resecata, and (D) caprellid amphipods. Shaded blue indicates 95% confidence intervals.
4 Discussion
4.1 Overall findings
Using choice feeding experiments and stable isotopes, we found that mesograzers in a Z. marina meadow were consuming allochthonous kelp. Kelp was the preferred food in experiments involving I. resecata and P. richii mesograzers relative to other primary producers (S. naiadum, Z. marina, U. lactuca). Yet, stable isotope results revealed a more varied uptake of allochthonous vs. autochthonous food depending on the mesograzer. N. luetkeana was an important contribution to the diets of I. resecata, Caprellid amphipods, and Lacuna snails, but less so to P. richii and gammarid amphipod diets. Epiphytic S. naiadum was the most consistent in-situ meadow macrophyte source isotopically integrated into mesograzer diets, highlighting the importance of this epiphyte to the Z. marina food web. S. naiadum also had the highest palatability via C:N ratio which may explain its favourability. We observed a positive relationship between gammarid amphipods and S. naiadum epiphytes, suggesting that there may be weak top-down control on epiphyte abundance by grazers in this system (see food web structure in Hessing-Lewis et al., 2018). We hypothesize that bottom-up drivers may play a key role in structuring this Z. marina food web, however experiments controlling for potential bottom-up (e.g., currents, allochthonous subsidies, edge effects) and top-down factors (e.g., grazing pressures, species composition) are needed to disentangle those complex interactions in this ecosystem.
4.2 Mesograzer feeding preferences and why kelp may be preferentially consumed
While our results suggest the incorporation of kelp into mesograzer diets for some species, we also observed high variability in diets among mesograzer species, as has been found elsewhere (Duffy and Harvilicz, 2001; Douglass et al., 2011). Mesograzers often have the choice of a variety of food sources (e.g., eelgrass, periphyton, bladed epiphytes, detritus/drift algae) that vary in availability and palatability through time and space. Different food sources may be more or less available to mesograzers depending on their mode of feeding (e.g., filter feeders vs. grazing invertebrates). For I. resecata, a common eelgrass-dwelling mesograzer, the feeding trial and isotopic data aligned well and suggested a strong preference for N. luetkeana over autochthonous Z. marina and S. naiadum (Figures 2, 4A). This result makes sense as I. resecata are highly mobile and able to consume detritus and larger plant material, and are likely to feed on kelp when available. I. resecata is known to consume Z. marina and microalgae (Best and Stachowicz, 2012; Lewis and Boyer, 2014). Less is known about I. resecata feeding preferences for N. luetkeana, however they are a well-acknowledged grazer in M. pyrifera kelp forests (Ng and Micheli, 2020). Feeding trial results for P. richii suggest a strong preference for N. luetkeana (Figure 2). However, the isotope results suggested a different longer-term trend, with larger contributions of S. naiadum, U. lactuca and Z. marina than N. luetkeana (Figure 4C).
Mesograzer preference for N. luetkeana over autochthonous primary production in Z. marina meadows may be explained by its bioavailability and palatability. Although both mesograzer species used for the feeding trials (I. resecata and P. richii) showed a strong preference for kelp in the lab, N. luetkeana subsidies may be temporally and spatially limited in the Z. marina meadow. P. richii crabs are often in the eelgrass canopy, where access to Z. marina, S. naiadum, and U. lactuca is plentiful. N. luetkeana may be less common in their preferred habitat, which may explain why it was less dominant in the isotope results. Additionally, because isotopic analysis captures a longer time-integrated window of feeding activity (e.g., days) (Zanden et al., 2015) relative to the feeding trials which represent a snapshot in time, it is reasonable to expect the isotope results to show a more equal distribution among macrophytes (Figure 4).
Kelp can bioaccumulate in large quantities within seagrass meadows at certain times of the year (Wernberg et al., 2006; Krumhansl and Scheibling, 2012), and mesograzers may be able to respond to these pulses for their nutritional benefit, as demonstrated in the lab experiments. The Z. marina meadow examined here is surrounded by both N. luetkeana and M. pyrifera kelp forests (Figure 1) which culminate to large quantities of sea wrack biomass in the area (Wickham et al., 2020) and is thus likely available via detrital and POM pathways to mesograzers. Sediment isotopic analysis in this region has found kelp in sediment carbon pools (Prentice et al., 2019), indicating its accumulation in the not necessary meadow. N. luetkeana is an annual species that exhibits higher rates of productivity in the summer months (Maxell and Miller, 1996), when breakage and sloughing can produce the drift material found in seagrass beds. Major exports of kelp in the fall occur past the peak S. naiadum growing season, and may fill an important part of mesograzers fall and winter diets.
Examining the C:N ratios of macrophytes, macroalgae (S. naiadum, U. lactuca, and N. luetkeana) had lower C:N ratios, suggesting relatively higher palatability compared to Z. marina (Table 1). Given that S. naiadum epiphyte loads can be high (up to 5.1g dry weight per eelgrass shoot), our results indicate that S. naiadum is an important autochthonous contributor to mesograzer diets. Other nutritional properties are not captured in the C:N ratio that may make N. luetkeana a desirable food source, such as low levels of polyphenolic defense compounds (Steinberg, 1985; Pennings et al., 2000) or increased fatty acids or polysaccharides. More generally, N. luetkeana appears to be a preferred food choice for a variety of nearshore mesograzers, as seen in not necessary Tegula funebralis (Steinberg, 1985) (Steinberg, 1985), Pugettia producta (Dobkowski et al., 2017), Idotea wosnesenskii (Dethier et al., 2014), and Strongylocentrotus droebachiensis larvae (Feehan et al., 2018).
Although we did not look at it explicitly due to its relatively low biomass in the area, the giant kelp M. pyrifera, integrate into the food web at similar isotopic values to N. luetkeana (e.g, Monteray Bay δ13C ranging from -14.93 ± 0.52 ‰ to -20.54 ± 0.81‰, Drobnitch et al., 2018). Given the dominance of N. luetkeana in the study area (Figure 1) it is likely we captured a representative take on mesograzer diets, however examining temporal feed preferences of M. pyrifera alongside N. luetkeana would be a worthwhile, particularly because M. pyrifera the biomass available year-round.
While we did not examine periphyton (e.g., diatoms) as a potential food source it represents another ubiquitous and sometimes abundant food source that should be considered. Epiphytic microalgae may be a more important food source for gastropod mesograzers than to arthropod mesograzers, due to their low mobility (Doropoulos et al., 2009). This also may explain why Z. marina was found to be the dominant contribution to Lacuna snail diets (Figure 4E), as they may ingest surface layers of Z. marina tissue while scraping the blades for periphyton. Studies elsewhere have shown that these epiphytes can have similar δ13C values to seagrass, examples ranging from -11.3 ± 0.81‰ (Jaschinski et al., 2008) to −15 ± 1.5 ‰ (Mittermayr et al., 2014), whereas Z. marina can range from -9.64 ± 0.65‰ (Jaschinski et al., 2008) to -13.4 ± 3.3 ‰ (Mittermayr et al., 2014).
In other nearshore regions, allochthonous kelp subsidies has been found in the diets of seagrass meadow mesograzers. In Australia, the kelp Ecklonia radiata made a notable contribution to the diets of two seagrass-dwelling gastropods (Doropoulos et al., 2009), and gastropod species were found to assimilate isotopically-labeled δ15N kelp under both field and laboratory conditions (Hyndes et al., 2012). Further, in-situ addition of kelp to Posidonia sinuosa seagrass plots increased the densities and biomass of the gastropod Strigosella lepidus and shrimp (Cartraud et al., 2021). Finally, the trophic incorporation of kelp subsidies by seagrass dwelling species has been demonstrated at higher levels of the food web such as fish (Wernberg et al., 2006; Olson et al., 2019).
4.3 Trophic implications of kelp subsidies to eelgrass meadows
When allochthonous inputs are high, there is potential for the recipient ecosystem’s food web structure to be altered (Zuercher and Galloway, 2019). In seagrass ecosystems, the relationship between mesograzers and epiphytes is important for maintaining meadow health, as mesograzers prevent epiphytes from outcompeting seagrass for light and nutrients. Based on this typical seagrass trophic structure, if mesograzers were primarily consuming epiphytes, we would expect to observe an inverse relationship between mesograzer abundance and epiphyte biomass. A preliminary glance at the trophic structure in Choked Passage revealed a positive relationship between gammarid amphipods and S. naiadum (Figure 5A).
In addition to being a food source, S. naiadum may be providing habitat for some mesograzer species, supporting the positive relationship observed with gammarid amphipods. Epiphytes are known to add structural complexity to meadows which can increase mesograzer abundance and diversity (Viejo, 1999). We anecdotally observed amphipods exhibiting tube-building behavior within the S. naiadum epiphytes, and unpublished gammarid amphipod-S. naiadum feeding trials revealed very little consumption of S. naiadum and only a minor increase in mass lost with an increase in amphipod number. Thus, there may be a number of biotic and abiotic factors, including the availability of allochthonous food sources, that may be driving this positive mesograzer-epiphyte relationship. Moreover, gammarid and caprellid amphipod biomass was highest at transects next to kelp, suggesting potential edge effects from kelp - increasing food for mesograzers; or adding habitat from increased structural complexity (Olson et al., 2019). These results support bottom-up structuring in this system with allochthonous subsidies playing some role in shaping this ecosystem, however it may be minor compared to the environmental drivers.
Matched seasonal dynamics and bottom-up control of epiphytes and mesograzers may be also at play (Fong et al., 2000; O’Connor et al., 2022). High currents in the study area likely contribute to the persistence of S. naiadum, as the constant replenishment of water can supply plentiful nutrients to both seagrass and epiphytes, and maintains a low turbidity water column which reduces competition for light (O’Connor et al., 2022). Our inference of kelp's role in structuring the food web is also limited by the observational nature of our study data. In situ experimental addition or exclusion of kelp subsidies would add more insight on the causal effects of kelp on mesograzer-epiphyte relationships (e.g., Cartraud et al., 2021). The expectation of a negative relationship may be more applicable to interactions with smaller epiphytes or diatoms where a reduction in biomass could be reduced expeditiously by a similar abundance of mesograzers. A subsidy effect may further vary based on characteristics of the focal epiphyte (e.g., habitat-forming, nutritional quality, availability) and mesograzers (e.g., size, mobility, feeding behavior). Further research to decipher mechanisms of bottom-up drivers is needed to understand the full effect of kelp to seagrass food webs.
5 Conclusion
Seagrass meadows are highly productive ecosystems that not only export large quantities of biomass, but can also receive energy via allochthonous kelp from neighboring habitats. Our results suggest that inputs of allochthonous kelp are important to recipient Z. marina food webs through mesograzer consumption. Seagrass and kelp forests face concurrent challenges across their ranges; thus gaining a better understanding of the prevalence and magnitude of linkages among marine ecosystems is timely. Further characterizing the flows of allochthonous energy into and out of seagrass habitats can help us better understand their roles in climate change mitigation and habitat provisioning.
Data availability statement
The original contributions presented in the study are included in the article/Supplementary Material, further inquiries can be directed to the corresponding author/s.
Author contributions
AO, CP, MHL, FJ participated in sampling design. AO, CP, DV collected the data, processed samples. AO, CP, ZM analyzed data. AO, CP, and MHL wrote the manuscript, and all authors provided valuable feedback on the manuscript. All authors contributed to the article and approved the submitted version.
Funding
Funding for this study was provided by the Tula Foundation and the University of Victoria.
Acknowledgments
We thank the Tula Foundation for research support for the Hakai Institute’s Nearshore Ecology Program. Research support was given by the University of Victoria to AO and FJ. We thank Hakai Staff (Tristan Blaine, and Natasha Salter) and the UVic Fisheries Ecology and Conservation Lab for their role in data collection, the Hakai Oceanography Program for the temperature data from Pruth Bay, and Hakai Geospatial for providing a map of the study area.
Conflict of interest
The authors declare that the research was conducted in the absence of any commercial or financial relationships that could be construed as a potential conflict of interest.
Publisher’s note
All claims expressed in this article are solely those of the authors and do not necessarily represent those of their affiliated organizations, or those of the publisher, the editors and the reviewers. Any product that may be evaluated in this article, or claim that may be made by its manufacturer, is not guaranteed or endorsed by the publisher.
Supplementary material
The Supplementary Material for this article can be found online at: https://www.frontiersin.org/articles/10.3389/fpls.2022.991744/full#supplementary-material
References
Adamczuk, M., Ferencz, B., Mieczan, T., Dawidek, J. (2019). Allochthonous subsidies as driving forces for development of plankton in an autotrophic, temperate, and small lake. Hydrobiologia 846, 59–73. doi: 10.1007/s10750-019-04052-9
Anderson, W. B., Polis, G. A. (1999). Nutrient fluxes from water to land: seabirds affect plant nutrient status on gulf of California islands. Oecologia 118, 324–332. doi: 10.1007/s004420050733
Best, R., Stachowicz, J. (2012). Trophic cascades in seagrass meadows depend on mesograzer variation in feeding rates, predation susceptibility, and abundance. Mar. Ecol. Prog. Ser. 456, 29–42. doi: 10.3354/meps09678
Buckner, E. V., Hernández, D. L., Samhouri, J. F. (2018). Conserving connectivity: Human influence on subsidy transfer and relevant restoration efforts. Ambio 47, 493–503. doi: 10.1007/s13280-017-0989-4
Carreón-Palau, L., Parrish, C. C., del Angel-Rodríguez, J. A., Pérez-España, H., Aguiñiga-García, S. (2013). Revealing organic carbon sources fueling a coral reef food web in the gulf of Mexico using stable isotopes and fatty acids. Limnol. Oceanogr. 58, 593–612. doi: 10.4319/lo.2013.58.2.0593
Cartraud, A.E., Lavery, P.S., Rae, C.M., Hyndes, G.A. (2021). Pathways to Spatial Subsidies by Kelp in Seagrass Meadows. Estuaries and Coasts 44, 468–80. doi: 10.1007/s12237-020-00860-8
Cook, K., Vanderklift, M. A., Poore, A. G. B. (2011). Strong effects of herbivorous amphipods on epiphyte biomass in a temperate seagrass meadow. Mar. Ecol. Prog. Ser. 442, 263–269. doi: 10.3354/meps09446
Dethier, M. N., Brown, A. S., Burgess, S., Eisenlord, M. E., Galloway, A. W. E., Kimber, J., et al. (2014). Degrading detritus: Changes in food quality of aging kelp tissue varies with species. J. Exp. Mar. Biol. Ecol. 460, 72–79. doi: 10.1016/j.jembe.2014.06.010
Dobkowski, K. A., Kobelt, J., Brentin, S., Van Alstyne, K. L., Dethier, M. N. (2017). Picky pugettia: a tale of two kelps. Mar. Biol. 164, 210. doi: 10.1007/s00227-017-3244-4
Douglass, J.G., Emmett Duffy, J., Canuel, E.A. (2011). Food Web Structure in a Chesapeake Bay Eelgrass Bed as Determined through Gut Contents and 13C and 15N Isotope Analysis. Estuaries and Coasts 34, 701–11. doi: 10.1007/s12237-010-9356-4
Doropoulos, C., Hyndes, G. A., Lavery, P. S., Tuya, F. (2009). Dietary preferences of two seagrass inhabiting gastropods: Allochthonous vs autochthonous resources. Estuar. Coast. Shelf Sci. 83, 13–18. doi: 10.1016/j.ecss.2009.03.011
Drobnitch, S. T., Pochron, T., Miranda, C. (2018). Patterns and drivers of δ13C variation in the giant kelp, Macrocystis pyrifera. Limnology Oceanography 63, 871–885. doi: 10.1002/lno.10675
Duarte, C. M., Krause-Jensen, D. (2017). Export from seagrass meadows contributes to marine carbon sequestration. Front. Mar. Sci. 4. doi: 10.3389/fmars.2017.00013
Duffy, J. E., Harvilicz, A. M. (2001). Species-specific impacts of grazing amphipods in an eelgrass-bed community. Mar. Ecol. Prog. Ser. 223, 201–211. doi: 10.3354/meps223201
Feehan, C. J., Grauman-Boss, B. C., Strathmann, R. R., Dethier, M. N., Duggins, D. O. (2018). Kelp detritus provides high-quality food for sea urchin larvae. Limnol. Oceanogr. 63, S299–S306. doi: 10.1002/lno.10740
Fong, C. W., Lee, S. Y., Wu, R. S. S. (2000). The effects of epiphytic algae and their grazers on the intertidal seagrass Zostera japonica. Aquat. Bot. 67, 251–261. doi: 10.1016/S0304-3770(00)00101-7
Heck, K. L., Carruthers, T. J. B., Duarte, C. M., Hughes, A. R., Kendrick, G., Orth, R. J., et al. (2008). Trophic transfers from seagrass meadows subsidize diverse marine and terrestrial consumers. Ecosystems 11, 1198–1210. doi: 10.1007/s10021-008-9155-y
Hendriks, I. E., Sintes, T., Bouma, T. J., Duarte, C. M. (2008). Experimental assessment and modeling evaluation of the effects of the seagrass Posidonia oceanica on flow and particle trapping. Mar. Ecol. Prog. Ser. 356, 163–173. doi: 10.3354/meps07316
Hessing-Lewis, M. L., Hacker, S. D. (2013). Upwelling-influence, macroalgal blooms, and seagrass production; temporal trends from latitudinal and local scales in northeast pacific estuaries. Limnol. Oceanogr. 58, 1103–1112. doi: 10.4319/lo.2013.58.3.1103
Hessing-Lewis, M., Rechsteiner, E. U., Hughes, B. B., Tim Tinker, M., Monteith, Z. L., Olson, A. M., et al. (2018). Ecosystem features determine seagrass community response to sea otter foraging. Mar. pollut. Bull. 134, 134–144. doi: 10.1016/j.marpolbul.2017.09.047
Hocking, M. D., Reimchen, T. E. (2009). Salmon species, density and watershed size predict magnitude of marine enrichment in riparian food webs. Oikos 118, 1307–1318. doi: 10.1111/j.1600-0706.2009.17302.x
Hughes, A. R., Bando, K. J., Rodriguez, L. F., Williams, S. L. (2004). Relative effects of grazers and nutrients on seagrasses: a meta-analysis approach. Mar. Ecol. Prog. Ser. 282, 87–99. doi: 10.3354/meps282087
Hughes, B. B., Eby, R., Van Dyke, E., Tinker, M. T., Marks, C. I., Johnson, K. S., et al. (2013). Recovery of a top predator mediates negative eutrophic effects on seagrass. Proc. Natl. Acad. Sci. 110, 15313–15318. doi: 10.1073/pnas.1302805110
Huxel, G. R., McCann, K. (1998). Food web stability: The influence of trophic flows across habitats. Am. Nat. 152, 460–469. doi: 10.1086/286182
Huxel, G. R., McCann, K., Polis, G. A. (2002). Effects of partitioning allochthonous and autochthonous resources on food web stability. Ecol. Res. 17, 419–432. doi: 10.1046/j.1440-1703.2002.00501.x
Hyndes, G. A., Lavery, P. S., Doropoulos, C. (2012). Dual processes for cross-boundary subsidies: incorporation of nutrients from reef-derived kelp into a seagrass ecosystem. Mar. Ecol. Prog. Ser. 445, 97–107. doi: 10.3354/meps09367
Hyndes, G. A., Nagelkerken, I., McLeod, R. J., Connolly, R. M., Lavery, P. S., Vanderklift, M. A. (2014). Mechanisms and ecological role of carbon transfer within coastal seascapes. Biol. Rev. 89, 232–254. doi: 10.1111/brv.12055
Jaschinski, S., Brepohl, D. C., Sommer, U. (2008). Carbon sources and trophic structure in an eelgrass zostera marina bed, based on stable isotope and fatty acid analyses. Mar. Ecol. Prog. Ser. 358, 103–114. doi: 10.3354/meps07327
Jaschinski, S., Sommer, U. (2008). Functional diversity of mesograzers in an eelgrass–epiphyte system. Mar. Biol. 154, 475–482. doi: 10.1007/s00227-008-0942-y
Krumhansl, K. A., Scheibling, R. E. (2012). Production and fate of kelp detritus. Mar. Ecol. Prog. Ser. 467, 281–302. doi: 10.3354/meps09940
Lafage, D., Bergman, E., Eckstein, R. L., Österling, E. M., Sadler, J. P., Piccolo, J. J. (2019). Local and landscape drivers of aquatic-to-terrestrial subsidies in riparian ecosystems: a worldwide meta-analysis. Ecosphere 10, e02697. doi: 10.1002/ecs2.2697
Lamb, A., Hanby, B. P. (2005). Marine life of the pacific Northwest: a photographic encyclopedia of invertebrates, seaweeds and selected fishes (Madeira Park: Harbour Publishing).
Lastra, M., Page, H. M., Dugan, J. E., Hubbard, D. M., Rodil, I. F. (2008). Processing of allochthonous macrophyte subsidies by sandy beach consumers: estimates of feeding rates and impacts on food resources. Mar. Biol. 154, 163–174. doi: 10.1007/s00227-008-0913-3
Lewis, J. T., Boyer, K. E. (2014). Grazer functional roles, induced defenses, and indirect interactions: Implications for eelgrass restoration in San Francisco bay. Diversity 6, 751–770. doi: 10.3390/d6040751
Liebowitz, D. M., Nielsen, K. J., Dugan, J. E., Morgan, S. G., Malone, D. P., Largier, J. L., et al. (2016). Ecosystem connectivity and trophic subsidies of sandy beaches. Ecosphere 7, e01503. doi: 10.1002/ecs2.1503
Loreau, M., Mouquet, N., Holt, R. D. (2003). Meta-ecosystems: a theoretical framework for a spatial ecosystem ecology. Ecol. Lett. 6, 673–679. doi: 10.1046/j.1461-0248.2003.00483.x
Marczak, L. B., Thompson, R. M., Richardson, J. S. (2007). Meta-analysis: Trophic level, habitat, and productivity shape the food web effects of resource subsidies. Ecology 88, 140–148. doi: 10.1890/0012-9658(2007)88[140:mtlhap]2.0.CO;2
Maxell, B. A., Miller, K. A. (1996). Demographic studies of the annual kelps Nereocystis luetkeana and Costaria costata (Laminariales, phaeophyta) in Puget Sound, Washington. Botanica Marina 39, 479–490. doi: 10.1515/botm.1996.39.1-6.479
Mittermayr, A., Fox, S. E., Sommer, U. (2014). Temporal variation in stable isotope composition (δ13C, δ15N and δ34S) of a temperate zostera marina food web. Mar. Ecol. Prog. Ser. 505, 95–105. doi: 10.3354/meps10797
Moksnes, P.-O., Gullström, M., Tryman, K., Baden, S. (2008). Trophic cascades in a temperate seagrass community. Oikos 117, 763–777. doi: 10.1111/j.0030-1299.2008.16521.x
Moore, J.W., Semmens, B.X. (2008). Incorporating uncertainty and prior information into stable isotope mixing models. Ecology Letters 11, 470–80. doi: 10.1111/j.1461-0248.2008.01163.x
Nakano, S., Murakami, M. (2001) Reciprocal subsidies: Dynamic interdependence between terrestrial and aquatic food webs. Proceedings of the National Academy of Sciences 98, 166–170. doi: 10.1073/pnas.98.1.166
Ng, C. A., Micheli, F. (2020). Size-dependent vulnerability to herbivory in a coastal foundation species. Oecologia 193, 199–209. doi: 10.1007/s00442-020-04655-3
O’Connor, M. I., Griffiths, G., Sanders-Smith, R., Hessing-Lewis, M., Davis, K. M., Forbes, C., et al. (2022). A reciprocal transplant experiment sheds new light on a classic marine seagrass-algal symbiosis and suggests influence of epiphytic symbiont on seagrass microbiota. Aquat. Bot. 179, 103511. doi: 10.1016/j.aquabot.2022.103511
Olson, A. M., Hessing-Lewis, M., Haggarty, D., Juanes, F. (2019). Nearshore seascape connectivity enhances seagrass meadow nursery function. Ecol. Appl. 29, e01897. doi: 10.1002/eap.1897
Orth, R. J., Van Montfrans, J. (1984). Epiphyte-seagrass relationships with an emphasis on the role of micrograzing: A review. Aquat. Bot. 18, 43–69. doi: 10.1016/0304-3770(84)90080-9
Pennings, S. C., Carefoot, T. H., Zimmer, M., Danko, J. P., Ziegler, A. (2000). Feeding preferences of supralittoral isopods and amphipods. Can. J. Zool. 78, 1918–1929. doi: 10.1139/z00-143
Peterson, C., Luettich, R., Micheli, F., Skilleter, G. (2004). Attenuation of water flow inside seagrass canopies of differing structure. Mar. Ecol. Prog. Ser. 268, 81–92. doi: 10.3354/meps268081
Polis, G. A., Hurd, S. D. (1996). “Allochthonous input across habitats, subsidized consumers, and apparent trophic cascades: Examples from the ocean-land interface,” in Food webs: Integration of patterns & dynamics. Eds. Polis, G. A., Winemiller, K. O. (Boston, MA: Springer US), 275–285. doi: 10.1007/978-1-4615-7007-3_27
Prentice, C., Hessing-Lewis, M., Sanders-Smith, R., Salomon, A. K. (2019). Reduced water motion enhances organic carbon stocks in temperate eelgrass meadows. Limnol. Oceanogr. 64 (6), 2389–2404. doi: 10.1002/lno.11191
R Core Team (2021). R: A language and environment for statistical computing (Vienna, Austria: R Foundation for Statistical Computing). Available at: https://www.R-project.org/.
Recalde, F. C., Breviglieri, C. P. B., Romero, G. Q. (2020). Allochthonous aquatic subsidies alleviate predation pressure in terrestrial ecosystems. Ecology 101, e0307. doi: 10.1002/ecy.3074
Recalde, F. C., Postali, T. C., Romero, G. Q. (2016). Unravelling the role of allochthonous aquatic resources to food web structure in a tropical riparian forest. J. Anim. Ecol. 85, 525–536. doi: 10.1111/1365-2656.12475
Rodewald, A. D., Kearns, L. J., Shustack, D. P. (2011). Anthropogenic resource subsidies decouple predator–prey relationships. Ecol. Appl. 21, 936–943. doi: 10.1890/10-0863.1
Sampaio, E., Rodil, I. F., Vaz-Pinto, F., Fernández, A., Arenas, F. (2017). Interaction strength between different grazers and macroalgae mediated by ocean acidification over warming gradients. Mar. Environ. Res. 125, 25–33. doi: 10.1016/j.marenvres.2017.01.001
Savage, C. (2019). Seabird nutrients are assimilated by corals and enhance coral growth rates. Sci. Rep. 9, 4284. doi: 10.1038/s41598-019-41030-6
Slim, F. J., Hemminga, M. A., de la Morinière, E. C., van der Velde, G. (1996). Tidal exchange of macrolitter between a mangrove forest and adjacent seagrass beds (Gazi bay, Kenya). Netherland J. Aquat. Ecol. 30, 119–128. doi: 10.1007/BF02272233
Smale, D. A., Moore, P. J., Queiros, A. M., Higgs, S., Burrows, M. T. (2018). Appreciating interconnectivity between habitats is key to blue carbon management. Front. Ecol. Environ. 16, 71–73. doi: 10.1002/fee.1765
Smit, A.J., Brearley, A., Hyndes, G.A., Lavery, P.S., Walker, D.I. (2006). δ15N and δ13C analysis of a Posidonia sinuosa seagrass bed. Aquatic Botany 84, 277–82. doi: 10.1016/j.aquabot.2005.11.005
Spiller, D. A., Piovia-Scott, J., Wright, A. N., Yang, L. H., Takimoto, G., Schoener, T. W., et al. (2010). Marine subsidies have multiple effects on coastal food webs. Ecology 91, 1424–1434. doi: 10.1890/09-0715.1
Steinberg, P. D. (1985). Feeding preferences of tegula funebralis and chemical defenses of marine brown algae. Ecol. Monogr. 55, 333–349. doi: 10.2307/1942581
Stock, B. C., Jackson, A. L., Ward, E. J., Parnell, A. C., Phillips, D. L., Semmens, B. X. (2018). Analyzing mixing systems using a new generation of Bayesian tracer mixing models. PeerJ 6, e5096. doi: 10.7717/peerj.5096
Taylor, R. B., Brown, P. J. (2006). Herbivory in the gammarid amphipod Aora typica: relationships between consumption rates, performance and abundance across ten seaweed species. Mar. Biol. 149, 455–463. doi: 10.1007/s00227-006-0245-0
Valentine, J. F., Duffy, J. E. (2006). “The central role of grazing in seagrass ecology,” in Seagrasses: Biology, ecology and conservation. Eds. Larkum, A. W. D., Orth, R. J., Duarte, C. M. (Dordrecht: Springer Netherlands), 463–501. doi: 10.1007/978-1-4020-2983-7_20
Vargas, C.A., Martinez, R.A., San Martin, V., Aguayo, M., Silva, N., Torres, R. (2011). Allochthonous subsidies of organic matter across a lake–river–fjord landscape in the Chilean Patagonia: Implications for marine zooplankton in inner fjord areas. Continental Shelf Research, Fjord Oceanography of the Chilean Patagonia 31, 187–201. doi: 10.1016/j.csr.2010.06.016
Viejo, R. M. (1999). Mobile epifauna inhabiting the invasive Sargassum muticum and two local seaweeds in northern Spain. Aquat. Bot. 64, 131–149. doi: 10.1016/S0304-3770(99)00011-X
Wernberg, T., Vanderklift, M. A., How, J., Lavery, P. S. (2006). Export of detached macroalgae from reefs to adjacent seagrass beds. Oecologia 147, 692–701. doi: 10.1007/s00442-005-0318-7
Whalen, M. A., Duffy, J. E., Grace, J. B. (2013). Temporal shifts in top-down vs. bottom-up control of epiphytic algae in a seagrass ecosystem. Ecology 94, 510–520. doi: 10.1890/12-0156.1
Wickham, S. B., Shackelford, N., Darimont, C. T., Nijland, W., Reshitnyk, L. Y., Reynolds, J. D., et al. (2020). Sea Wrack delivery and accumulation on islands: factors that mediate marine nutrient permeability. Mar. Ecol. Prog. Ser. 635, 37–54. doi: 10.3354/meps13197
Zanden, M.J.V., Clayton, M. K., Moody, E. K., Solomon, C. T., Weidel, B. C. (2015). Stable Isotope Turnover and Half-Life in Animal Tissues: A Literature Synthesis. PLOS ONE 10, e0116182. doi: 10.1371/journal.pone.0116182
Keywords: seascape, epiphytes, stable isotopes, allochthonous subsidies, trophic interactions, eelgrass
Citation: Olson AM, Prentice C, Monteith ZL, VanMaanen D, Juanes F and Hessing-Lewis M (2022) Grazing preference and isotopic contributions of kelp to Zostera marina mesograzers. Front. Plant Sci. 13:991744. doi: 10.3389/fpls.2022.991744
Received: 11 July 2022; Accepted: 20 September 2022;
Published: 13 October 2022.
Edited by:
Mirta Teichberg, Marine Biological Laboratory (MBL), United StatesReviewed by:
Karine Gagnon, Norwegian Institute of Marine Research (IMR), NorwayCatherine Ann Pfister, The University of Chicago, United States
Copyright © 2022 Olson, Prentice, Monteith, VanMaanen, Juanes and Hessing-Lewis. This is an open-access article distributed under the terms of the Creative Commons Attribution License (CC BY). The use, distribution or reproduction in other forums is permitted, provided the original author(s) and the copyright owner(s) are credited and that the original publication in this journal is cited, in accordance with accepted academic practice. No use, distribution or reproduction is permitted which does not comply with these terms.
*Correspondence: Angeleen M. Olson, YW5nZWxlZW4ub2xzb25AaGFrYWkub3Jn
†These authors have contributed equally to this work