- College of Landscape Architecture, Northeast Forestry University, Harbin, China
Lilium pumilum is a perennial herb with ornamental edible and medicinal value. It is an excellent wild germplasm resource with wide distribution and strong resistance. The NAC family of transcription factors is unique to higher plants. The NAC family plays a regulatory role in plant growth and development and participates in plant responses to biotic and abiotic stresses. The LpNAC17 gene of L. pumilum was cloned and transformed into tobacco to investigate the response of transgenic tobacco to salt stress. The results showed that the net photosynthetic rate and contents of chlorophyll in LpNAC17 over-expressed tobacco were higher than those in the control plants, while the stomatal conductance, transpiration rate and intercellular CO2 concentration were lower than those in the controls. The activity of superoxide dismutase, peroxidase, catalase, and the content of proline in LpNAC17 over-expressed tobacco were higher than those in the controls, while the content of malondialdehyde, superoxide anion, and hydrogen peroxide were lower than that in the control. Nitro-blue tetrazolium staining and 3,3′-diaminobenzidine tissue localization showed that the contents of and H2O2 in transgenic tobacco was lower than in the controls. The expression levels of NtSOD, NtPOD, NtCAT, NtHAK1, NtPMA4, and NtSOS1 in the transgenic tobacco were higher than those in the controls. Therefore, this study provides a gene source for molecular breeding of salt-tolerant plants through genetic engineering, and lays a foundation for further research on salt-tolerant Lily.
Introduction
Plants are often affected by various abiotic stresses including the stresses of salt, drought, extreme temperature (high temperature, low temperature) during their growth and development. These stresses seriously affect the ecological and economic value of plants (Zhang and Ma, 2019). Thus, plants have to respond to these stresses to minimize the stress-induced damage (Pérez et al., 2013). Many studies have shown that plant cells can sense stresses and transmit stress signals to stress-responding transcription factors (TFs) through complex signaling pathways. TFs can regulate the expression of target genes in plants by binding its DNA binding domain to cis-acting elements in the promoter regions of target genes (Karam et al., 2002; Chen and Zhu, 2004; Huang et al., 2012). Many TF families such as the NAC, bZIP, MYB, and WRKY families have been found to play important roles in plant growth and development, and responses to abiotic stresses (Dortje et al., 2011). The NAC gene family is a large family of transcription factors unique to plants. So far, there are 117 NAC genes in Arabidopsis thaliana, 151 NACs in rice (Ooka et al., 2003; Mohammed et al., 2010), 163 NACs in Populus trichocarpa (Rui et al., 2010), 152 NACs in soybean (Dung et al., 2011), 283 NACs in upland cotton (Sun et al., 2018), 152 NACs in maize (Kaliyugam et al., 2014), 154 NACs in tobacco (Li et al., 2018), 168 NACs in durum wheat (Saidi et al., 2017). NAC TFs participate in plant morphogenesis, root growth and elongation, and the responses to various abiotic stresses. In Rosa chinensis “Old Blush,” the expression of RcNAC72 was significantly induced by drought, cold, salinity, and abscisic acid (ABA), and overexpression of RcNAC72 in A. thaliana enhanced its tolerance to drought stress (Jia et al., 2022). Knockout of OsNAC3 reduced rice sensitivity to ABA and increased its sensitivity to salt stress, whereas overexpression of OsNAC3 had the opposite effect (Zhang et al., 2021). Similarly, silencing and overexpression of the SlNAC6 gene in tomato decreased and enhanced tolerance to drought, respectively (Jian et al., 2021). MdNAC047 in apple (Malus domestica) enhances tolerance to salt stress by inducing ethylene accumulation (An et al., 2018). In the analysis of the expression pattern of SmNACs in eggplant, it was found that they are involved in the regulation of responses to a variety of abiotic stresses (Wan et al., 2021). In addition, the Pearl millet (Pennisetum glaucum) PgNAC21, the horsegram (Macrotyloma uniflorum) MuNAC4, and the tomato (Solanum lycopersicum) NAC transcription factor JUNGBRUNNEN1 (JUB1) also positively regulate responses to abiotic stresses (Pandurangaiah et al., 2014; Thirumalaikumar et al., 2018; Harshraj et al., 2019). However, studies have shown that NAC transcription factors also exhibit a negative regulatory role in plant responses to abiotic stresses, e.g., ANAC069 increased sensitivity to salt and osmotic stress by reducing reactive oxygen species scavenging capacity (He et al., 2017). Similarly, ZmNAC071 enhanced the sensitivity to ABA and osmotic stress by downregulating the expression of superoxide dismutase (SOD), peroxidase (POD), and other genes in transgenic A. thaliana (He et al., 2019). Therefore, NAC transcription factors regulate stress tolerance in plants through multiple pathways and are important for plants to cope with abiotic stress.
Lilium pumilum is a perennial wild herb. Its flower shape is beautiful and has high ornamental value. Moreover, its bulb and flower have edible and medicinal values. It is mainly distributed in Northeast and Northern China. It has a strong tolerance to cold, drought, and saline-alkali stresses and a strong resistance to diseases. It is an excellent wild germplasm resource (Wang et al., 2018a,b). So far, a small number of genes have been cloned from L. pumilum and transferred into tobacco. Studies have shown that over-expression of L. pumilum LpNAC13 and LpPEX7 genes can enhance salt and alkali tolerance in tobacco (He et al., 2020; Wang et al., 2020). The salt tolerance of L. pumilum APX and LpNAC6 overexpression plants was significantly enhanced under salt stress (Chen et al., 2010; Liu et al., 2020). However, there are still many valuable NAC transcription factors that have not been functionally characterized in L. pumilum. In the present study, we found that LpNAC17 in L. pumilum responds to salt stress according to the analysis of transcriptome data and NAC gene expression. We cloned the LpNAC17 gene from L. pumilum and transformed it into tobacco. We found that the overexpression of the LpNAC17 gene in tobacco significantly improved the salt resistance of transgenic plants, showing enhanced photosynthetic rate, osmotic regulation substance content, and antioxidant enzyme activities of transgenic tobacco under salt stress. These results indicated that LpNAC17 was positively correlated with salt tolerance, permitting further research on the molecular mechanism of LpNAC17-mediated regulation of salt stress in L. pumilum.
Results
Identification of the LpNAC17 gene in L. pumilum
Sequence analysis indicated that the open reading frame (ORF) of LpNAC17 gene is 507 bp long and encodes a NAC gene family protein of 168 amino acids in length. The molecular weight of LpNAC17 was 19021.02 kDa, and the theoretical isoelectric point was 9.64, indicating that LpNAC17 was a hydrophilic stable protein. The results of the prediction of the transmembrane structure and signal peptide showed that the protein does not contain a transmembrane domain or signal peptide, and is a non-secreted protein. A Blast search of Genbank showed that the highest sequence homology between L. pumilum and Cinnamomum micranthum was 91.1% for LpNAC17 (Figure 1A), and the phylogenetic analysis showed that LpNAC17 forms a monophylogenic group with its homologs in Prosopis alba and Phoenix dactylifera (Figure 1B). Real-time RT-PCR (qPCR) analysis showed that LpNAC17 was expressed in the root, bulb, and leaf of L. pumilum with the highest expression level in bulb (Figure 1C).
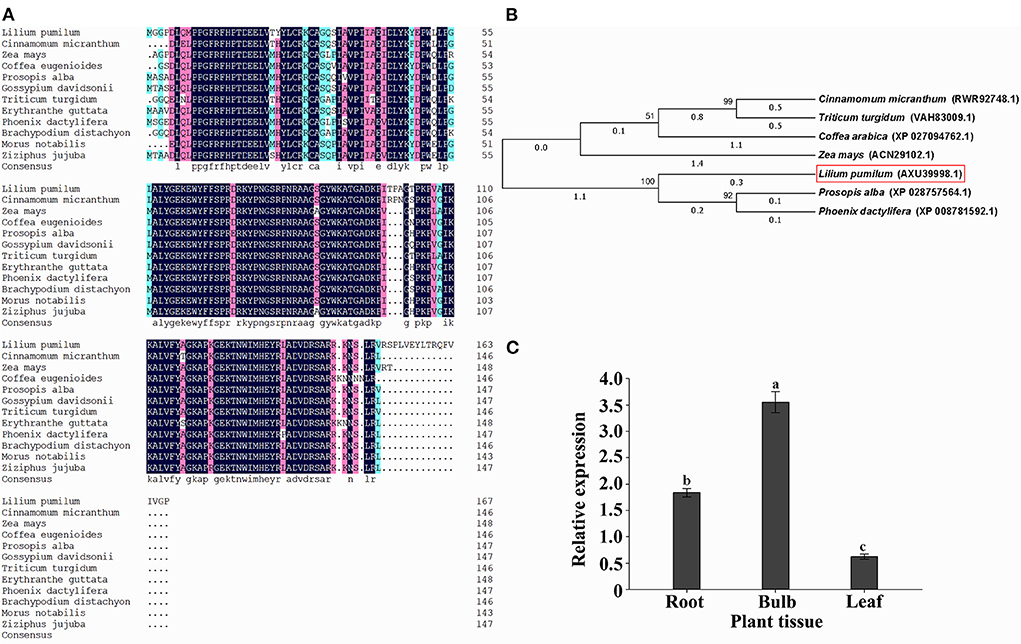
Figure 1. Sequence and expression analysis of LpNAC17. (A) Multiple sequence alignment of the deduced amino acid sequences of LpNAC17 from L. pumilum with other NACs from Cinnamomum micranthum (RWR92746.1), Zea mays (ACN29102.1), Coffea eugenioides (XP_027148352.1), Prosopis alba (XP_028757564.1), Gossypium davidsonii (MBA0625903.1), Triticum turgidum (VAH67189.1), Erythranthe guttata (XP_012844117.1), Phoenix dactylifera (XP_008781592.1), Brachypodium distachyon (XP_003564785.1), Morus notabilis (XP_010095416.1), Ziziphus jujuba (XP_015885739.1). (B) Phylogenetic tree of LpNAC17 and its homologs. (C) Expression analysis of LpNAC17 gene in different tissues of L. pumilum. LilyActin was used as the internal control gene. Bars represent the SE of three independent experiments and the different letters indicate the significant difference at P < 0.05.
Expression of the LpNAC17 gene in L. pumilum under abiotic stress
We investigated how the expression of LpNAC17 in L. pumilum seedlings responded to the individual treatments of ABA, NaCl, drought and cold at 1, 3, 6, 12, 24, 48 h post treatment (hpt). Under ABA treatment, the expression levels of LpNAC17 in roots, bulbs and leaves were induced when compared with that in the mock-treated plants within 48 hpt (Figures 2A–C). The highest induced expression was 58.08 times in roots at 1 hpt, 3.97 times in bulbs at 48 h, and 141.04 times in leaves at 12 h, respectively. Under NaCl stress, the LpNAC17 expression in the three tissues were increased when compared with that in the mock-treated plants within 48 hpt (Figures 2D–F). The highest induced expression was 12.12 times in roots at 12 hpt, 31.41 times in bulbs at 12 hpt, and 3.24 times in leaves at 48 hpt. For the drought stress, the LpNAC17 expression in the three tissues were also induced when compared with that in the mock-treated plants within 48 hpt (Figures 2G–I). The highest induced expression was 86.82 times in roots at 24 hpt, 5.18 times in bulbs at 48 hpt, and 7.46 times in leaves at 48 hpt. The cold stress treatment also induced the LpNAC17 expression in the three tissues within 48 hpt (Figures 2J–L). The highest induced expression was 12.46 times in roots at 12 hpt, 26 times in bulbs at 24 hpt, and 714.58 times in leaves at 12 hpt. As a result, the expression levels of LpNAC17 in the three tissues were significantly induced by each of the ABA, NaCl, drought, and cold treatments.
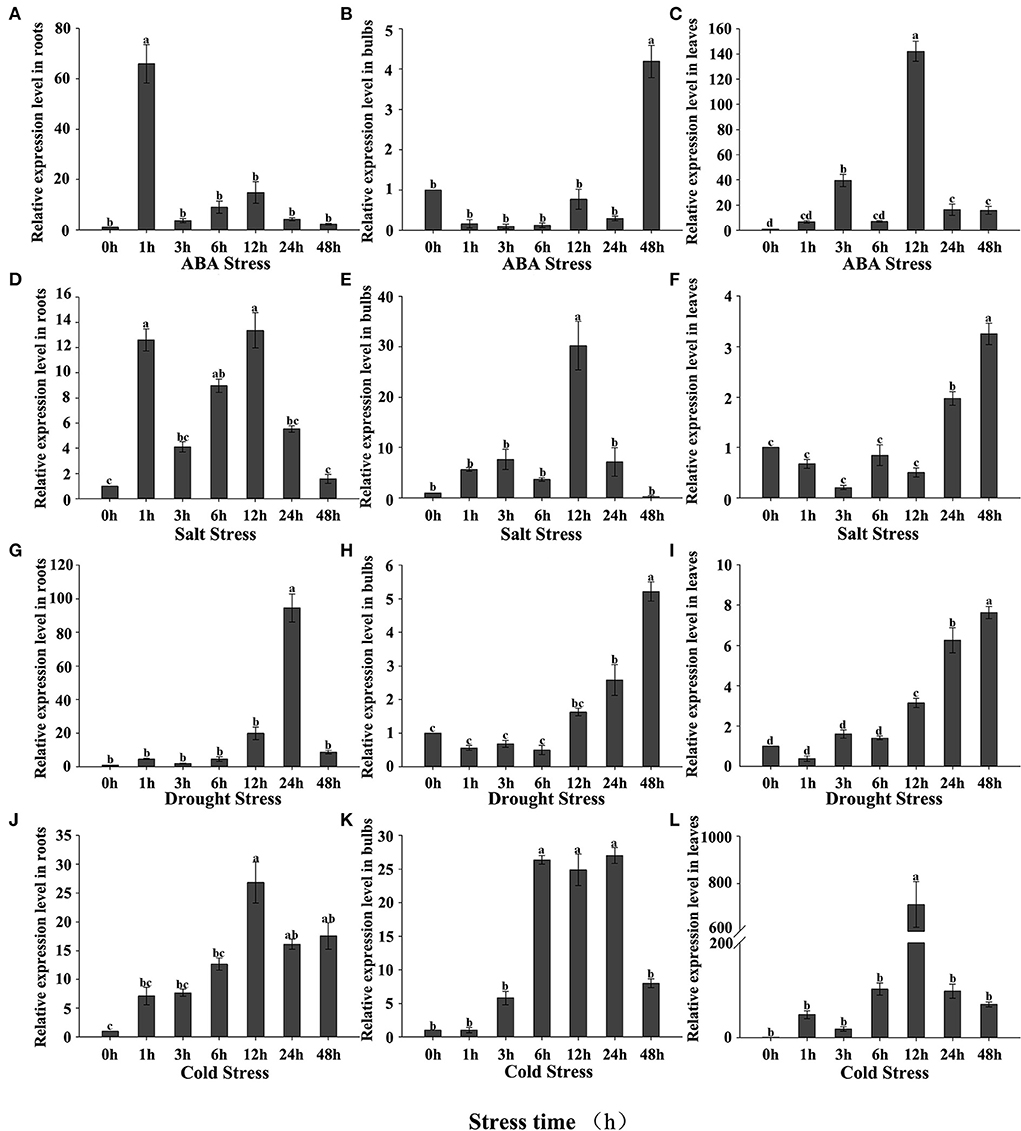
Figure 2. Expression patterns of LpNAC17 in roots, bulbs, and leaves of L. pumilum under abiotic stress treatment. (A–C) 150 μM ABA treatment. (D–F) 200 mM NaCl treatment. (G–I) 20% PEG-6000 treatment. (J–L) 2°C treatment. Bars represent the SE of three independent experiments and the different letters indicate the significant difference at P < 0.05.
Generation of transgenic tobacco lines overexpressing LpNAC17
The ORF of LpNAC17 was cloned into the pBI121 vector to form pBI121-LpNAC17- GFP (Figures 3A,B), followed by Agrobacterium-mediated tobacco transformation. A total of 6 potential transgenic lines with normal growth and development were obtained. PCR amplification of the transgene LpNAC17 confirmed the presence of the transgene in 4 out of the 6 potential transgenic lines (Figure 3C). qPCR analysis of the relative expression levels of LpNAC17 in the four transgenic lines showed that the single-copied homozygous OE-1 and OE-3 lines had high expression levels (Figure 3E), whose seedlings grew normally in the Kanamycin selection media (Figure 3D). Thus, these two lines were selected for subsequent experiments.
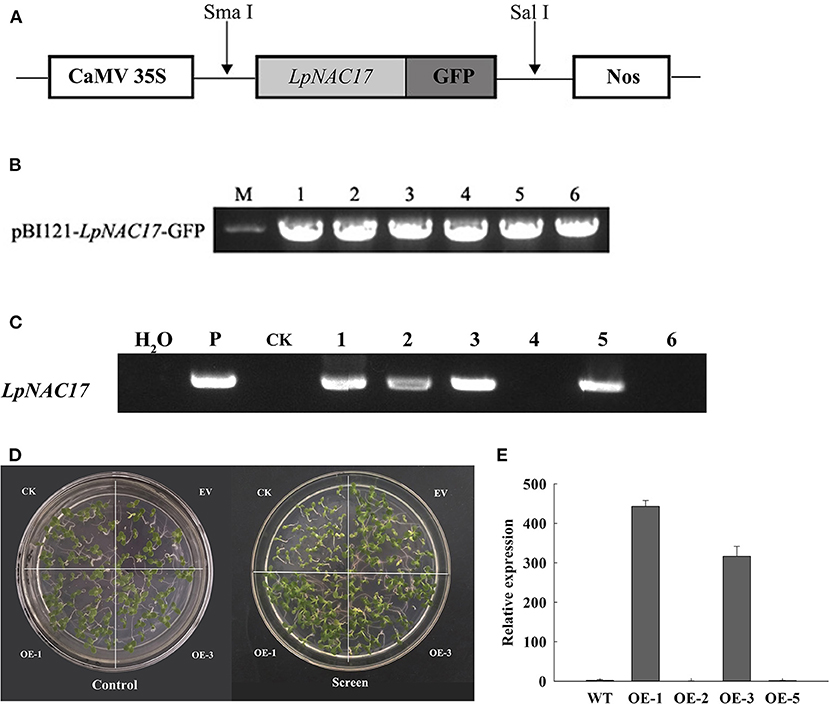
Figure 3. Generation of transgenic tobacco overexpressing LpNAC17. (A) Schematic diagram of T-DNA region of the expression vector containing 35S: LpNAC17-NosT. (B) Construction of binary vector pBI121- LpNAC17-GFP. M, 2000 DNA marker; 1–6, verification of binary vectors. (C) PCR confirmation of the presence of LpNAC17 in transgenic tobacco lines. P, plasmid DNA as a positive control; H2O, sterile distilled water as a negative control; CK, wild-type tobacco; #1, 2, 3, and 5, transgenic tobacco lines. (D) Growth of CK, EV and the OE-1 and OE-3 lines on the media containing Kanamycin. (E) Relative expression of LpNAC17 in the transgenic tobacco lines.
The effect of the overexpressed LpNAC17 on salt tolerance
To explore whether the transgenic tobacco lines overexpressing LpNAC17 are tolerant of salt stress, the 5-week-old wild-type tobacco (CK), transgenic tobacco lines with the empty vector (EV) and the OE-1 and OE-3 lines were used for salt stress treatment. There was no apparent difference in the morphology of the tobacco plants at 0 day post treatment (dpt; Figures 4A,B). After 7 dpt, 2–3 leaves on the lower part of each of CK and EV tobacco plants began to wilt, but the growth of OE-1 and OE-3 plants was not affected. After the 15 dpt, the leaves on the lower part of the CK and EV tobacco plants started to turn yellow and drooped, and the upper leaves began to wilt, while the leaves near the root of OE-1 and OE-3 lines just began to wilt, indicating an enhanced tolerance to salt stress in the transgenic tobacco lines overexpressing LpNAC17.
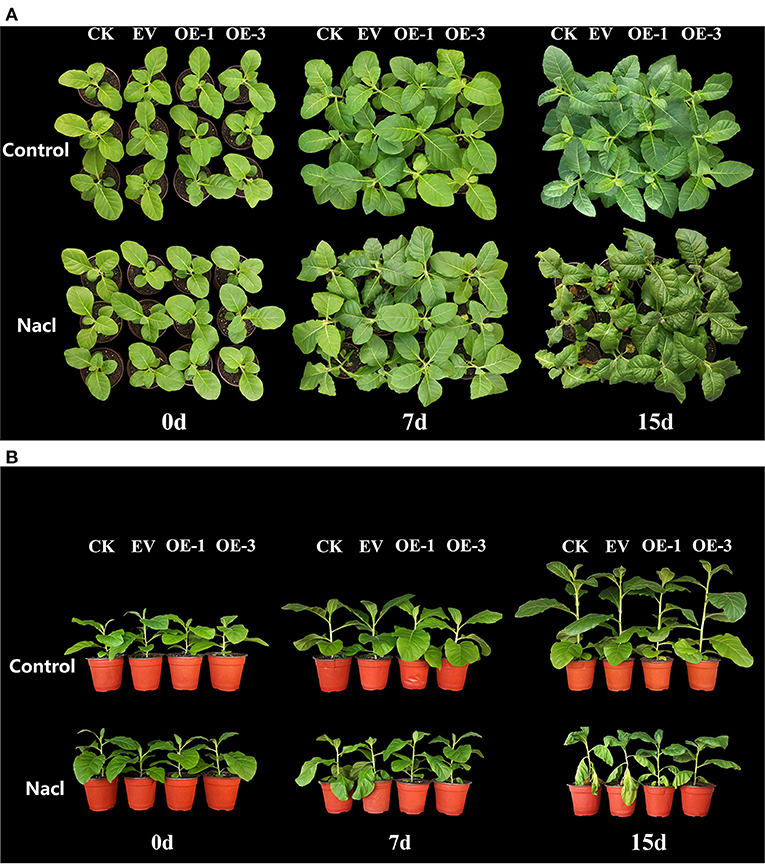
Figure 4. Analysis of salt tolerance in the transgenic tobacco lines overexpressing LpNAC17. (A) Comparative top view images. (B) Comparative side view images.
The effect of the overexpressed LpNAC17 on photosynthetic capacity under salt stress
Photosynthesis is the basis of energy conversion and the most sensitive physiological activity to salt stress in plants. Net photosynthetic rate (Pn) can directly reflect the photosynthetic capacity of plants, since it is positively correlated with the latter. We found that the Pn of all of the tobacco leaves decreased gradually with the increase in salt stress treatment duration (Figure 5A). However, the Pn of OE-1 and OE-3 lines was significantly higher than that of CK and EV at 7 and 15 dpt (P < 0.05), and the Pn of OE-1 and OE-3 lines was 1.85 and 1.29 times higher than that of CK at 15 dpt.
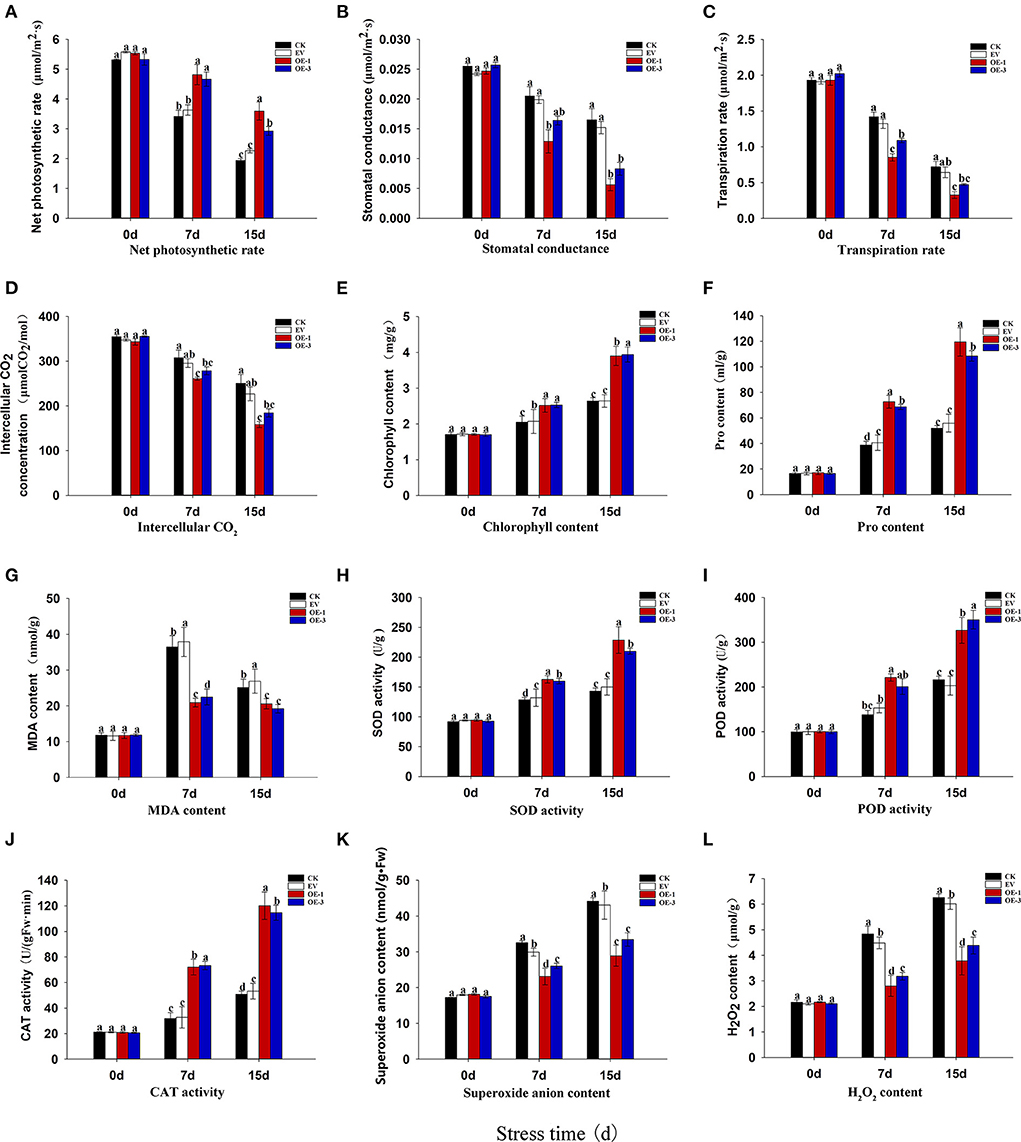
Figure 5. Analysis of photosynthetic capacity in the transgenic tobacco lines overexpressing LpNAC17 under salt stress. (A) Net photosynthetic rate (Pn). (B) Stomatal conductance (Gs). (C) Transpiration rate (Tr). (D) Intercellular CO2 concentration (Ci). (E) Chlorophyll (Chl) content. (F) Proline (Pro) content. (G) malondialdehyde (MDA) content. (H) Superoxide dismutase (SOD) activities. (I) Peroxidase (POD) activities. (J) catalase (CAT) activities. (K) Superoxide anion () content. (L) Hydrogen peroxide (H2O2) content. Bars represent the SE of three independent replicates and the different letters indicate the significant difference at the same time point (P < 0.05).
The stomatal conductance (Gs), transpiration rate (Tr), and intercellular CO2 concentration (Ci) of all the tobacco plants showed an obvious decreasing trend with the increase in the stress treatment duration (Figures 5B–D). However, the decrease degrees of these three parameters in the OE-1 and OE-3 lines were significantly greater than that of CK and EV (P < 0.05). The Gs, Tr, Ci of the OE-1 and OE-3 lines were decreased by 65.79 and 49.67%, 54.80 and 34.52%, 36.75 and 26.36%, respectively, when compared with CK at 15 dpt, indicating that salt stress had significant inhibitory effect on the three parameters, and the inhibitory effect on the OE-1 and OE-3 lines was more obvious than in the CK and EV. The decrease in Ci may be caused by the joint action of Gs and Pn. The decrease in Gs restricts the entry of external gas into tobacco leaves, while the increase in Pn consumes more carbon dioxide in leaves, thus leading to the decline in Ci in tobacco leaves.
Chlorophyll (Chl) synthesis is an important component in plant photosynthesis. At 15 dpt, the Chl content of all the tobacco leaves increased continuously, and the OE-1 and OE-3 lines showed a significantly higher Chl content than that of CK and EV (Figure 5E). The Chl contents in the OE-1 and OE-3 lines were 1.47 and 1.49 times higher than that of CK, respectively, at 15 dpt. The increase of Chl content may be one of the reasons for the increased Pn in tobacco.
The effect of the overexpressed LpNAC17 on ROS levels and ROS scavenger activities under salt stress
The proline (Pro) contents were gradually increased in all the tobacco plants with the increase in salt stress treatment time, while the Pro contents in the OE-1 and OE-3 lines were significantly higher than that of CK and EV at 7 and 15 dpt (Figure 5F). The malondialdehyde (MDA) contents in all the tobacco plants increased at 7 dpt and then decreased at 15 dpt (Figure 5G). However, the MDA content in the OE-1 and OE-3 lines was significantly lower than that in CK and EV at both time points.
To study the effect of the overexpressed LpNAC17 on reactive oxygen species (ROS) homeostasis, we examined the activities of key ROS scavengers superoxide dismutase (SOD), peroxidase (POD), and catalase (CAT). There was no significant difference between treatments at 0 dpt. The activities of the SOD (Figure 5H), POD (Figure 5I), and CAT (Figure 5J) in all the tobacco leaves were gradually increased when plants were subjected to salt stress, and their activities in the OE-1 and OE-3 lines were significantly higher than those of CK and EV at 7 and 15 dpt (P < 0.05).
In order to further determine the ROS content in tobacco leaves, we measured the contents of superoxide anion () and hydrogen peroxide (H2O2). The contents of and H2O2 gradually accumulated in all the tobacco plants with the increase in salt stress treatment time (Figures 5K,L). However, the contents of and H2O2 in the OE-1 and OE-3 lines were significantly lower than that in CK and EV at 7 and 15 dpt (P < 0.05). Histochemical staining analysis using nitro-blue tetrazolium (NBT; for ) and 3,3′-diaminobenzidine (DAB; for H2O2) staining showed the leaves of the OE-1 and OE-3 lines were stained much lighter than that of the CK and EV at 7 and 15 dpt (Figures 6A,B), indicating less contents of and H2O2 in the OE-1 and OE-3 lines. These results were consistent with the content determination of and H2O2 in Figures 5K,L.
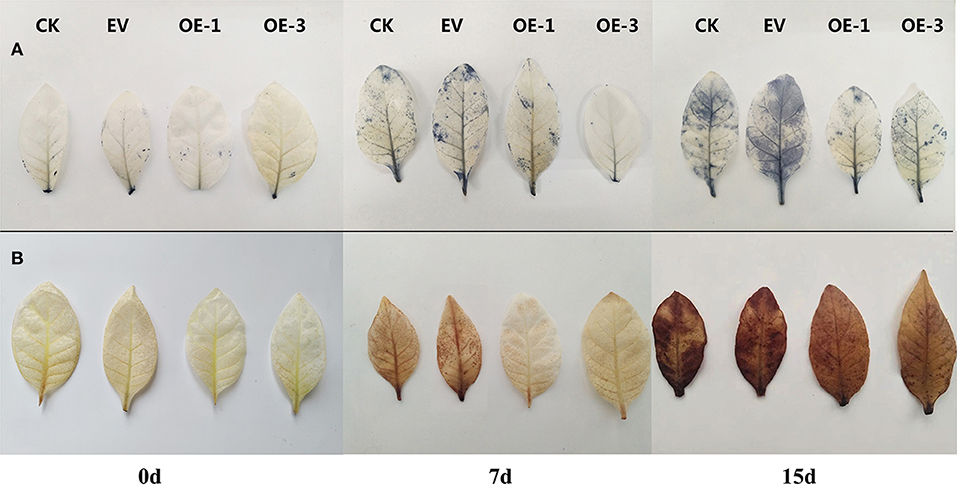
Figure 6. Histochemical staining of the leaves of the transgenic tobacco lines overexpressing LpNAC17 under salt stress. Accumulation of (A) O2 −and (B) H2O2 was revealed by histochemical staining with NBT and DAB, respectively.
The effect of the overexpressed LpNAC17 on expression levels of stress-related genes NtSOD, NtPOD, NtCAT, NtHAK1, NtPMA4, and NtSOS1 under salt stress
In order to further verify the role of LpNAC17 in salt stress tolerance, the relative expression levels of stress-related genes NtSOD, NtPOD, NtCAT, NtHAK1, NtPMA4, and NtSOS1 were quantified using qPCR. We found that the relative expression levels of these genes were significantly up-regulated in all the tobacco plants at 7 and 15 dpt but the increase of each gene in the OE-1 and OE-3 lines was significantly higher than that of the CK and EV at both time points (Figures 7A–F). The expression levels of NtSOD, NtPOD, and NtCAT in the OE-1 and OE-3 lines were 1.66 and 1.55, 2.60 and 2.19, 1.59 and 1.78 times higher than that of CK at 7 and 15 dpt, respectively. The expression levels of NtHAK1, NtPMA4, and NtSOS1 in the OE-1 and OE-3 lines were 4.04 and 3.72, 3.64 and 3.85, 2.80 and 2.84 times higher than that of CK at both time points, respectively.
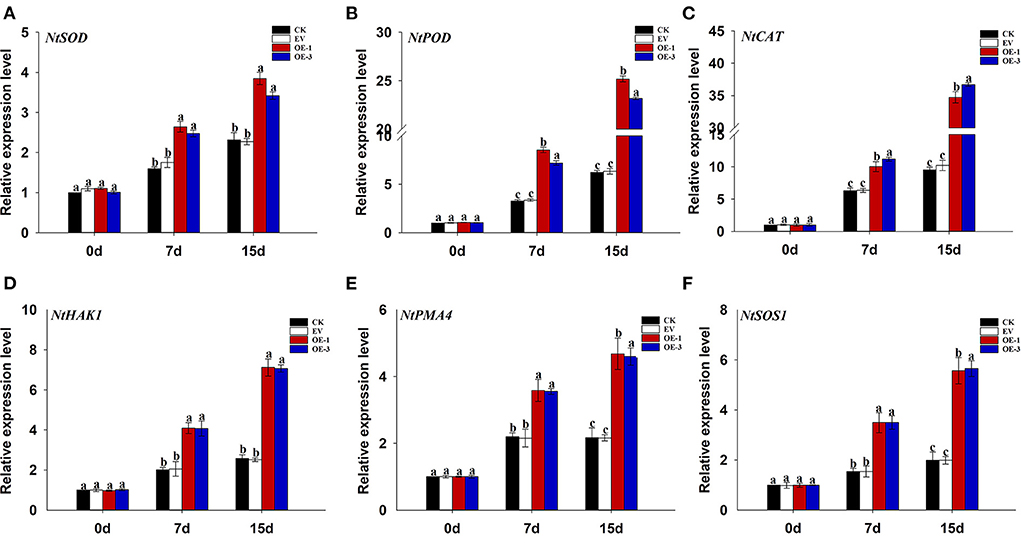
Figure 7. Analysis of the changes in the expression of stress-related genes in the leaves of the transgenic tobacco lines overexpressing LpNAC17 under salt stress. (A) NtSOD. (B) NtPOD. (C) NtCAT. (D) NtHAK1. (E) NtPMA4. (F) NtSOS1. The tobacco Actin gene was used as the internal control gene. Bars represent the SE of three independent replicates and the different letters indicate the significant difference at the same time point (P < 0.05).
Discussion
To withstand the negative effects caused by salt stress, plants have evolved various biochemical and molecular mechanisms, such as regulating osmotic balance and ions balance to respond to the stress (Munns, 1993; Chen and Polle, 2010; Ruiz-Lozano et al., 2012; Gong et al., 2020). A number of TF genes have been reported as having effect on improving tolerance to salt and other abiotic stresses. Numerous studies have shown that plant NAC family members play a key role in response to abiotic stresses. It has been reported that the over-expression of ONAC063 (Naoki et al., 2009), GmNAC20 (Yu et al., 2011), GmNAC11 (Yu et al., 2011), GmNAC06 (Li et al., 2020), VvNAC17 (Ju et al., 2020), MlNAC12 (Yang et al., 2018), and StNAC053 (Wang et al., 2021) genes can enhance plant salt tolerance. L. pumilum is a kind of perennial herb with strong resistance, which is mainly distributed in most areas of north China. A few NAC genes from L. pumilum have been studied and found to improve salt tolerance (Cao, 2019). In our pre-screening of the salt-tolerance NAC family genes in L. pumilum, LpNAC17 with highly salt-inducible expression was selected for the present study.
LpNAC17 is expressed in all tissues under normal growth condition. It showed an up-regulated expression following drought, salt, cold, and ABA treatments, suggesting that LpNAC17 is involved in the ABA signaling pathway. Leaf wilting was found in all tobacco plants under salt stress, but the transgenic lines overexpressing LpNAC17 suffered less damage. Photosynthesis is one of the important indicators reflecting the response ability of plant photosynthesis to stress (Liu et al., 2013). Studies have shown that salt stress can affect the activity of Chl enzymes and destroy the chloroplast structure, thereby reducing the Chl content in plant leaves (Zhu and Zhu, 1999; Zhou, 2020). In the present study, the contents of Chl and Pn in the transgenic tobacco overexpressing LpNAC17 was significantly higher than that of the control plants, indicating that LpNAC17 gene can enhance photosynthesis of tobacco under salt stress by increasing the Chl content. However, the role of LpNAC17 in promoting Chl synthesis or protecting Chl from degradation under salt stress is unclear and needs further study. In addition, the Tr, Ci, and Gs of the transgenic lines overexpressing LpNAC17 decreased more rapidly than that of the control plants under salt stress. Many previous studies have also shown that the reductions in these indicators are often accompanied by the reductions in Pn (Farquhar and Sharkey, 1982; Huo et al., 2020; Jia et al., 2021). However, Delatorre-Herrera et al. (2021) found that the rate of CO2 assimilation was less dependent on stomatal conductance in the salt-tolerant ecotype (Amarilla) of quinoa. They showed that there is another diffusion mechanism involved in carbon dioxide assimilation, such as mesophyll conduction (Gm), and found that the salt-tolerant ecotype performed better under salt stress mainly due to higher photochemical efficiency and greater ribulose-1,5-bisphosphate carboxylase/oxygenase (RubisCO) activity in that ecotype (Delatorre-Herrera et al., 2021). These findings suggest that plant species and their tolerance mechanisms vary in their gas exchange properties. Therefore, we speculate that the transgenic lines overexpressing LpNAC17 may also maintain a higher Pn through other mechanisms, such as higher Chl content and higher photochemical efficiency, and greater RubisCO activity. The change of ABA content in plant leaves is caused by salt stress, and ABA is closely related to stomatal closure. Previous studies have shown that overexpressing apple MdUGT88F4 gene in transgenic lines resulted in lower ABA content under salt stress than that of wild-type plants, and the transcription level of ABA synthesis gene MdNCED3 in the leaves of each line was increased by salt treatment (Chen, 2021). In the present study, the LpNAC17 gene was up-regulated by ABA treatment (Figures 2A–C), indicating that LpNAC17 may regulate the salt tolerance of the transgenic tobacco plants through the ABA signaling pathway, which needs further examination.
Under salt stress, a large amount of ROS is produced. Excessive ROS could increase membrane lipid peroxidation and cause damage to the cellular membrane (Gill and Tuteja, 2010), resulting in the generation of massive secondary products such as MDA that can indirectly reflect ROS in plants (Moore and Roberts, 1998; Farmer and Mueller, 2013). In this study, we found that the content of MDA in LpNAC17 transgenic plants was lower than that in the control plants under salt stress, indicating that the cell membrane damage of the transgenic plants under salt stress was lower than that of control plants. In order to reduce the damage of salt stress to plants, plants usually increase the concentration of osmotic substances, such as Pro, to improve plant tolerance. Osmotic adjustment substances play roles in protecting cellular macromolecules, protecting the cell membrane structure to improve stress tolerance (Zhang et al., 2020). It has been reported in previous studies that NAC TFs regulate the content of osmotic regulators in plants in response to salt stress. For example, tobacco plants overexpressing CsNAC1/2/3 gene accumulated higher levels of osmotically active substances than the control plants under salt stress (Liu et al., 2022), and transgenic plants overexpressing VuNAC1/2 exhibited higher Pro content and lower MDA content than the control plants under salt stress (Srivastava et al., 2022). In the present study, Pro content in the transgenic plants overexpressing LpNAC17 is significantly higher than in the controls under salt stress. This result implied that LpNAC17 can reduce the damage to cell membrane under salt stress to a certain extent, and play a role in maintaining the stability of cell membrane under salt stress. These data suggest that over-expression of LpNAC17 could positively regulate Pro content to confer a better ability to resist salt stress in the transgenic tobacco.
The antioxidant enzymes play an important role in coping with excess ROS to reduce oxidative stress. To maintain cell homeostasis, the antioxidant system in plants plays a dominant role in enhancing the activities of antioxidant enzymes, including SOD, POD, and CAT, to prevent plants from being damaged by salt stress (Jaleel et al., 2009). Antioxidant enzymes can eliminate the damage of and ROS to cells. Many reports indicate that NAC TFs are actively involved in the regulation of ROS metabolism and induction of important ROS scavenging enzymes (Jia et al., 2019; Figueroa et al., 2021; Jin et al., 2021; Mei et al., 2021). Consistent with these studies, we found that under salt stress, the activities of SOD, CAT, and POD were increased in LpNAC17 over-expression tobacco while the contents of and H2O2 and the level of ROS were less than those in the control plants. These results indicated that the improvement of ROS homeostasis is an important reason for LpNAC17-mediated salt tolerance.
In order to further verify the function of LpNAC17 under salt stress, the expression levels of several key genes of plant stress tolerance were detected in transgenic plants overexpressing LpNAC17 after salt stress. The results showed that under salt stress, the expression of genes related to scavenging ROS (NtPOD, NtSOD, NtCAT) were induced, and improving plant salt tolerance could be achieved by scavenging excess ROS in plants (Lai et al., 2020). We found that the expression levels of NtPOD, NtSOD, NtCAT, NtSOS1, NtHAK1, and NtPMA4 were significantly up-regulated in the transgenic tobacco overexpressing LpNAC17 when compared with the control plants, indicating that LpNAC17 could improve the salt tolerance of transgenic tobacco by increasing the expression of ROS genes and sodium-potassium ion balance genes. SOS1 plays a role in the process of Na+ efflux from cells, which can protect plants from salt poisoning caused by excessive intracellular Na+ (Eduardo, 2000). PMA4 can regulate cellular K+ uptake and balance K+ concentration (Moriau et al., 1993). Moreover, HAK1 can balance intracellular Na+/K+ and prevent excessive intracellular Na+ content from causing toxicity to cells (Qin et al., 2015).
We present a working model for the putative regulatory function of LpNAC17 in responses to salt stress in transgenic tobacco (Figure 8). Overexpression of the TF LpNAC17 can affect the expression of multiple stress-related genes and indirectly upregulate the activity of ROS scavenging enzymes. Furthermore, increased LpNAC17 expression may trigger the ABA signaling pathway. Taken together, all these changes resulted in decreased ROS accumulation, enhanced photosynthetic capacity, and increased salt tolerance in transgenic tobacco overexpressing LpNAC17.
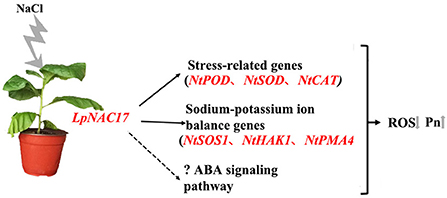
Figure 8. Putative working model of the LpNAC17 regulatory function in the transgenic tobacco in response to salt stress treatment. Overexpression of LpNAC17 in tobacco activates the expression of stress-related genes and sodium and potassium ion balance factors, and possibly the ABA signaling pathway, leading to lower accumulation of reactive oxygen species (ROS) and higher Pn under salt stress and thus improved salt tolerance.
Conclusion
In conclusion, a stress-responsive NAC gene LpNAC17 from L. pumilum was identified and its function was characterized. Overexpression of LpNAC17 enhanced the salinity tolerance of transgenic tobacco at the morphological, physiological and molecular levels. This study provides a functional gene for lily salt tolerance breeding, and lays a foundation for revealing the molecular mechanism of NAC transcription factors under salt stress and for further studying the salt tolerance mechanism in L. pumilum.
Materials and methods
Gene cloning and sequence analysis
Total RNA was extracted from bulbs of L. pumilum and cDNA synthesis was conducted as described in Wang et al. (2020). Gene-specific primers were designed for the LpNAC17 gene (accession #: MF398208.1) are shown in Supplementary Table S1. PCR amplification was performed with KOD-plus-neo (Toyobo, Japan). The PCR products were digested with SmaI and SalI and cloned into the PBI121-GFP empty vector with the LpNAC17 gene being driven by the full-length 35S promoter, followed by being transformed into Escherichia coli DH-5α. After Sanger sequencing confirmation, the destination vector was transformed into Agrobacterium tumefaciens EHA105 by the electroporation method.
The amino acid sequence of the LpNAC17 protein was analyzed using various bioinformatics tools as described in Ma et al. (2017). BLASTp was used to search for homologous sequences in Genbank, and sequences with high sequence homology were selected for sequence alignment. Phylogenetic analysis was constructed by using DNAMAN.
Tobacco transformation and analysis of transgenic tobacco lines
Tobacco transformation was performed using Agrobacterium-mediated transformation of tobacco leaf disks using 50 mg/L Kanamycin for transgenic plant selection (Wang, 2020). Genomic DNA was extracted using the modified CTAB method and PCR was performed as described above. Total RNA extraction and qPCR were conducted as described above. Copy number of the transgene was determined by the segregation ration, and two single-copied homozygous transgenic lines with high transgene expression were selected for subsequent experiments.
Plant abiotic stress treatment
Twenty-eight-day-old seedlings of L. pumilum were pre-cultured with Hoagland's nutrient solution for 1 week and then transferred to Hoagland's nutrient solution containing 200 mM NaCl, 20% PEG or 150 μM ABA. For cold stress, pre-cultured seedlings were transferred to Hoagland's nutrient solution and cultivated in a 2°C artificial climate incubator. Seedlings treated with Hoagland's nutrient solution or 25°C were used as the negative control plants.
Two-week-old transgenic tobacco seedlings overexpressing LpNAC17 were grown on soil: vermiculite: perlite =3:1:1 culture medium at 26°C with 16 h light/8 h darkness for 3 weeks, followed by the treatment with 100 mL NaCl solution with a concentration of 300 mmol/L once per 2 days. Water was used as the mock treatment. Phenotypic analysis and leaf tissue harvest were conducted at 0, 7 and 15 d of salt stress.
Real-time RT-PCR analyses
Total RNA was extracted from the roots, bulbs and leaves of L. pumilum after each treatment at the time points of 0, 1, 3, 6, 12, 24, or 48 hpt using TRIzol. Total RNA was also extracted from leaves of transgenic tobacco under 300 mM NaCl stress on 0, 7, and 15 dpt. cDNA synthesis was conducted using the ReverTra Ace® qPCR RT Kit (Toyobo, Japan). qPCR was conducted using the 2 × Fast qPCR Master Mixture (CWBIO, China) and 3 biological replicates as described in Zhao et al. (2021). qPCR was performed with the following cycling conditions: 94°C for 2 min followed by 35 cycles at 94°C for 15 s, 60°C for 15 s, and 72°C for 10s. Each 20 μL of reaction mix included 10 μL of 2 × Fast qPCR Master Mixture, 0.4 μL of cDNA, 0.4 μL of Primer-F, 0.4 μL of Primer-R, 8.8 μL of dd H2O. The LilyActin gene (accession #: JX826390) and the tobacco NtActin gene (accession #: no.U60495) were used as the internal control gene for and tobacco, respectively. The relative expression of genes was analyzed using the 2−ΔΔCt method.
Assays of photosynthetic parameters in tobacco under salt stress
Li-6400 portable photosynthetic instrument (LI-COR, USA) was used to measure the photosynthetic parameters of tobacco plant leaves from 9:00 a.m. to 10:00 a.m. The photosynthetic parameters included leaf net photosynthetic rate (Pn), stomatal conductance (Gs), intercellular CO2 concentration (Ci), and transpiration rate (Tr). Before measurement, the photosynthetic instrument was adjusted and preheated. Three plants (replicates) were randomly selected from each line, and the 3rd to 4th leaf were selected from each plant. After the readings stabilized, the data were recorded. Each leaf blade was recorded for 5 times, and the average value was used for each replicate.
Determination of physiological indexes of transgenic tobacco under salt stress
Malondialdehyde (MDA) accumulation was measured using the thiobarbituric acid-based method (Hodges et al., 1999). The proline (Pro) content was measured using the acid ninhydrin method (Bates et al., 1973). The chlorophyll (Chl) content was determined using the acetone extraction method (Zou et al., 2000; Liu and Li, 2016b,c). The superoxide dismutase (SOD) activity was measured using the nitro blue tetrazolium colorimetric method (Liu and Li, 2016a). The peroxidase (POD) activity was measured using the guaiacol colorimetric method (Zou et al., 2000; Liu and Li, 2016b,c). The catalase (CAT) activity was measured using the ammonium molybdate colorimetric method (Zou et al., 2000; Liu and Li, 2016b,c). The superoxide anion () was determined by hydroxylamine oxidation method (Huang et al., 2010). The hydrogen peroxide (H2O2) content was determined using hydrogen peroxide (H2O2) content kit (Greis Biotechnology, China). The levels of and H2O2 were detected with p-Nitro-Blue tetrazolium chloride (NBT) (Liu et al., 2008) and 3,3′- diaminobenzidine tetrahydrochloride (DAB) (Mason et al., 2016) staining, respectively. For the determination of antioxidant enzymes (SOD, POD, and CAT), pre-weighed tobacco leaves (0.5 g) were placed in a pre-cooled mortar, and 5 mL of pre-cooled 50 mmol·L−1 (pH 7.8) phosphate buffer (added in several times) was added. The leaves were ground into a homogenate under an ice bath, centrifuged at 3,000 rpm for 10 min at 4°C, and the supernatant was used for the determination of antioxidant enzymes. All these experiments were performed in triplicate.
Data availability statement
The data presented in the study are deposited in the NCBI repository, accession number: MF398208.
Author contributions
YpingW, YC, and YZ conceived the project and wrote the manuscript. YpingW, YC, BL, YingW, SS, JW, MT, and HY conducted the experiment, collected the data, and helped with manuscript preparation. YingW, YC, and YZ analyzed the data. All the authors approved the manuscript.
Funding
This research was funded by the Fundamental Research Funds for Central Universities (2572019BK04) and the Heilongjiang Province Natural Science Foundation (LH2019C004).
Acknowledgments
We are very grateful to the editor and reviewers for critically evaluating the manuscript and providing constructive comments for its improvement.
Conflict of interest
The authors declare that the research was conducted in the absence of any commercial or financial relationships that could be construed as a potential conflict of interest.
Publisher's note
All claims expressed in this article are solely those of the authors and do not necessarily represent those of their affiliated organizations, or those of the publisher, the editors and the reviewers. Any product that may be evaluated in this article, or claim that may be made by its manufacturer, is not guaranteed or endorsed by the publisher.
Supplementary material
The Supplementary Material for this article can be found online at: https://www.frontiersin.org/articles/10.3389/fpls.2022.993841/full#supplementary-material
References
An, J. P., Yao, J. F., Xu, R. R., You, C. X., Wang, X. F., and Hao, Y. J. (2018). An apple NAC transcription factor enhances salt stress tolerance by modulating the ethylene response. Physiol. Plant. 164, 279–289. doi: 10.1111/ppl.12724
Bates, L. S., Waldren, R. P., and Teare, I. D. (1973). Rapid determination of free proline for water-stress studies. Plant Soil 39, 205–207. doi: 10.1007/BF00018060
Cao, S. J. (2019). Cloning and Salt-Resistant Function Analysis in Tobacco of LpNAC6 and LpNAC20 Genes From Lilium pumilum (Master thesis). Northeast Forestry University, Harbin, China.
Chen, L., Xin, H. B., Sun, X. R., Yin, H., Li, X. X., and Yi, M. F. (2010). Molecular cloning APX from Lilium longiforum and overexpressing to Arabidopsis thaliana enhanced salt tolerance. Acta Hortic. Sin. 37, 1983–1990. doi: 10.16420/j.issn.0513-353x.2010.12.027
Chen, S., and Polle, A. (2010). Salinity tolerance of populus. Plant Biol. 12, 317–333. doi: 10.1111/j.1438-8677.2009.00301.x
Chen, W. J., and Zhu, T. (2004). Networks of transcription factors with roles in environmental stress response. Trends Plant Sci. 9, 591–596. doi: 10.1016/j.tplants.2004.10.007
Chen, X. F. (2021). Function Analysis of Apple MdUGT88F4 Gene Under Drought and Salt Stress. (Master thesis). Northwest A&F University, Xianyang, China.
Delatorre-Herrera, J., Ruiz, K. B., and Pinto, M. (2021). The importance of non-diffusional factors in determining photosynthesis of two contrasting quinoa ecotypes (Chenopodium quinoa Willd.) subjected to salinity conditions. Plants 10, 927. doi: 10.3390/plants10050927
Dortje, G., Ines, L., and Oksoon, Y. (2011). Plant tolerance to drought and salinity: stress regulating transcription factors and their functional significance in the cellular transcriptional network. Plant Cell Rep. 30, 1383–1391. doi: 10.1007/s00299-011-1068-0
Dung, T. L., Rie, N., Yasuko, W., Keiichi, M., Kazuko, Y. S., Kazuo, S., et al. (2011). Genome-wide survey and expression analysis of the plant-specific NAC transcription factor family in soybean during development and dehydration stress. DNA Res. 18, 263–276. doi: 10.1093/dnares/dsr015
Eduardo, B. (2000). Sodium transport and salt tolerance in plants. Curr. Opin. Cell Biol. 12, 431–434. doi: 10.1016/S0955-0674(00)00112-5
Farmer, E. E., and Mueller, M. J. (2013). ROS-mediated lipid peroxidation and RES-activated signaling. Annu. Rev. Plant Physiol. 64, 429–450. doi: 10.1146/annurev-arplant-050312-120132
Farquhar, G. D., and Sharkey, T. D. (1982). Stomatal conductance and photosynthesis. Annu. Rev. Plant Physiol. 3, 317–345. doi: 10.1146/annurev.pp.33.060182.001533
Figueroa, N., Lodeyro, A. F., Carrillo, N., and Gómez, R. (2021). Meta-analysis reveals key features of the improved drought tolerance of plants overexpressing NAC transcription factors. Environ. Exp. Bot. 186, 104449. doi: 10.1016/j.envexpbot.2021.104449
Gill, S. S., and Tuteja, N. (2010). Reactive oxygen species and antioxidant machinery in abiotic stress tolerance in crop plants. Plant Physiol. Biochem. 48, 909–930. doi: 10.1016/j.plaphy.2010.08.016
Gong, Z. Z., Xiong, L. M., Shi, H. Z., Yang, S. H., Herrera-Estrella, L. R., Xu, G. H., et al. (2020). Plant abiotic stress response and nutrient use efficiency. Sci. China. Life Sci. 63, 635–674. doi: 10.1007/s11427-020-1683-x
Harshraj, S., Ambika, D., Daisuke, T., Shashi, K. G., Liu, S. K., and Tetsuo, T. (2019). Pearl millet stress-responsive NAC transcription factor PgNAC21 enhances salinity stress tolerance in Arabidopsis. Plant Physiol. Biochem. 135, 546–553. doi: 10.1016/j.plaphy.2018.11.004
He, H., Zhu, G. Q., Chen, S. Y., Xu, C., and Jin, S. M. (2020). Cloning of LpPEX7 gene from Lilium pumilum and its expression characteristics under salt stress. Bull. Bot. Res. 40, 274–283. doi: 10.7525/j.issn.1673-5102.2020.02.015
He, L., Bian, J., Xu, J. Y., and Yang, K. J. (2019). Novel maize NAC transcriptional repressor ZmNAC071 confers enhanced sensitivity to ABA and osmotic stress by downregulating stress-responsive genes in transgenic Arabidopsis. J. Agric. Food Chem. 67, 8905–8918. doi: 10.1021/acs.jafc.9b02331
He, L., Shi, X. X., Wang, Y. M., Guo, Y., Yang, K. J., and Wang, Y. C. (2017). Arabidopsis ANAC069 binds to C[A/G]CG[T/G] sequences to negatively regulate salt and osmotic stress tolerance. Plant Mol. Biol. 93, 369–387. doi: 10.1007/s11103-016-0567-3
Hodges, D. M., DeLong, J. M., Forney, C. F., and Prange, R. K. (1999). Improving the thiobarbituric acid-reactive-substances assay for estimating lipid peroxidation in plant tissues containing anthocyanin and other interfering compounds. Planta 207, 604–611. doi: 10.1007/s004250050524
Huang, G. T., Ma, S. L., Bai, L. P., Zhang, L., Ma, H., Jia, P., et al. (2012). Signal transduction during cold, salt, and drought stresses in plants. Mol. Biol. Rep. 39, 969–987. doi: 10.1007/s11033-011-0823-1
Huang, X. S., Liu, J. H., and Chen, X. J. (2010). Overexpression of PtrABF gene, a bZIP transcription factor isolated from Poncirus trifoliata, enhances dehydration and drought tolerance in tobacco via scavenging ROS and modulating expression of stress-responsive genes. BMC Plant Biol. 10, 230. doi: 10.1186/1471-2229-10-230
Huo, L. Q., Sun, X., Guo, Z. J., Jia, X., Che, R. M., Sun, Y. M., et al. (2020). MdATG18a overexpression improves basal thermotolerance in transgenic apple by decreasing damage to chloroplasts. Hortic. Res. 7, 21. doi: 10.1038/s41438-020-0243-2
Jaleel, C. A., Riadh, K., Gopi, R., Manivannan, P., Inès, J., Al-Juburi, H. J., et al. (2009). Antioxidant defense responses: physiological plasticity in higher plants under abiotic constraints. Acta Physiol. Plant. 31, 427–436. doi: 10.1007/s11738-009-0275-6
Jia, D. F., Jiang, Q., Nocker, S. V., Gong, X. Q., and Ma, F. W. (2019). An apple (Malus domestica) NAC transcription factor enhances drought tolerance in transgenic apple plants. Plant Physiol. Biochem. 139, 504–512. doi: 10.1016/j.plaphy.2019.04.011
Jia, X., Mao, K., Wang, P., Wang, Y., Jia, X. M., Huo, L. Q., et al. (2021). Overexpression of MdATG8i improves water use efficiency in transgenic apple by modulating photosynthesis, osmotic balance, and autophagic activity under moderate water deficit. Hortic. Res. 8, 81. doi: 10.1038/s41438-021-00521-2
Jia, X., Zeng, Z., Lyu, Y., and Zhao, S. (2022). Drought-responsive NAC transcription factor RcNAC72 is recognized by RcABF4, interacts with RcDREB2A to enhance drought tolerance in Arabidopsis. Int. J. Mol. Sci. 23, 1755. doi: 10.3390/ijms23031755
Jian, W., Zheng, Y. X., Yu, T. T., Cao, H. H., Chen, Y., Cui, Q. Y., et al. (2021). SlNAC6, a NAC transcription factor, is involved in drought stress response and reproductive process in tomato. J. Plant Physiol. 264, 153483. doi: 10.1016/j.jplph.2021.153483
Jin, X. Y., Long, Y. F., Xiong, S. Q., Yang, Z., Chen, W., Hawar, A., et al. (2021). SbNAC2 enhances abiotic stress tolerance by upregulating ROS scavenging activities and inducing stress-response genes in sorghum. Environ. Exp. Bot. 192, 104664. doi: 10.1016/j.envexpbot.2021.104664
Ju, Y. L., Yue, X. F., Min, Z., Wang, X. H., Fang, Y. L., and Zhang, J. X. (2020). VvNAC17, a novel stress-responsive grapevine (Vitis vinifera L.) NAC transcription factor, increases sensitivity to abscisic acid and enhances salinity, freezing, and drought tolerance in transgenic Arabidopsis. Plant Physiol. Biochem. 146, 98–111. doi: 10.1016/j.plaphy.2019.11.002
Kaliyugam, S., Rinku, S., Krishan, K., Shiv, K. Y., Firoz, H., and Nepolean, T. (2014). Genome-wide identification and expression pattern of drought-responsive members of the NAC family in maize. Meta Gene 2, 407–417. doi: 10.1016/j.mgene.2014.05.001
Karam, B. S., Rhonda, C. F., and Luis, O. (2002). Transcription factors in plant defense and stress responses. Curr. Opin. Plant Biol. 5, 430–436. doi: 10.1016/S1369-5266(02)00289-3
Lai, W., He, P., Xu, M. Y., Chen, L. H., Liu, S. Q., and Yang, Y. G. (2020). Research progress on cucumber salt tolerance and salt tolerance related genes. China Veg. 6, 16–22. CNKI:SUN:ZGSC.0.2020-06-005
Li, M., Chen, R., Jiang, Q., Sun, X. J., Zhang, H., and Hu, Z. (2020). GmNAC06, a NAC domain transcription factor enhances salt stress tolerance in soybean. Plant Mol. Biol. 105, 1–13. doi: 10.1007/s11103-020-01091-y
Li, W., Li, X. X., Chao, J. T., Zhang, Z. L., Wang, W. F., and Guo, Y. F. (2018). NAC family transcription factors in tobacco and their potential role in regulating leaf senescence. Front. Plant Sci. 9, 1900. doi: 10.3389/fpls.2018.01900
Liu, B., Cao, S. J., Wang, Y., Cui, Y., Yue, H., and Zhang, Y. N. (2020). Overexpression of LpNAC6 gene in Lilium pumilum enhancing salt tolerance in transgenic tobacco. J. Beijing For. Univ. 42, 69–79. doi: 10.12171/j.1000-1522.20190342
Liu, H., Hu, H. R., Tang, K. L., Rehman, M., Du, G. H., Huang, Y., et al. (2022). Overexpressing hemp salt stress induced transcription factor genes enhances tobacco salt tolerance. Ind. Crop. Prod. 177, 114497. doi: 10.1016/j.indcrop.2021.114497
Liu, R. H., Fu, S. Y., and Zhang, H. Y. (2008). A novel spectrophotometric assay for superoxide radical anion quantification. 2nd Int. Papermaking Environ. Confer. 27, 355–359.
Liu, Z. B., Cheng, R. M., Xiao, W. F., Wang, R. L., Feng, X. H., and Wang, X. R. (2013). Effect of waterlogging on photosynthetic and physioecological characteristics of plants. World Forestry Res. 26, 33–38. doi: 10.13348/j.cnki.sjlyyj.2013.03.001
Ma, X. J., Zhang, B., Liu, C. J., Tong, B. T., Guan, T., and Xia, D. (2017). Expression of a populus histone deacetylase gene 84KHDA903 in tobacco enhances drought tolerance. Plant Sci. 265, 1–11. doi: 10.1016/j.plantsci.2017.09.008
Mason, G. A., Lemus, T., and Queitsch, C. (2016). The mechanistic underpinnings of an ago1-mediated, environmentally dependent, and stochastic phenotype. Plant Physiol. 170, 2420–2431. doi: 10.1104/pp.15.01928
Mei, F. M., Chen, B., Li, F. F., Zhang, Y. F., Kang, Z. S., Wang, X. J., et al. (2021). Overexpression of the wheat NAC transcription factor TaSNAC4-3A gene confers drought tolerance in transgenic Arabidopsis. Plant Physiol. Biochem. 160, 37–50. doi: 10.1016/j.plaphy.2021.01.004
Mohammed, N., Ramaswamy, M., Akhter, M. S., Kouji, S., Hiroaki, K., Hisako, O., et al. (2010). Genome-wide analysis of NAC transcription factor family in rice. Gene 465, 30–44. doi: 10.1016/j.gene.2010.06.008
Moore, K., and Roberts, L. J. (1998). Measurement of lipid peroxidation. Free Radic. Res. 28, 659–671. doi: 10.3109/10715769809065821
Moriau, L., Bogaerts, P., Jonniaux, J. L., and Boutry, M. (1993). Identification and characterization of a second plasma membrane H+-ATPase gene subfamily in Nicotiana plumbaginifolis. Plant Mol. Biol. 21, 955–963. doi: 10.1007/BF00023594
Munns, R. (1993). Physiological processes limiting plant growth in saline soils: some dogmas and hypotheses. Plant Cell Environ. 16, 15–24. doi: 10.1111/j.1365-3040.1993.tb00840.x
Naoki, Y., Takanari, I., Youichi, K., Minami, M., Hirohiko, H., Masaki, I., et al. (2009). Tolerance to various environmental stresses conferred by the salt-responsive rice gene ONAC063 in transgenic Arabidopsis. Planta 229, 1065–1075. doi: 10.1007/s00425-009-0895-5
Ooka, H., Satoh, K., Doi, K., Nagata, T., Otomo, Y., Murakami, K., et al. (2003). Comprehensive analysis of NAC family genes in Oryza sativa and Arabidopsis thaliana. DNA Res. 10, 239–247. doi: 10.1093/dnares/10.6.239
Pandurangaiah, M., Lokanadha, R. G., Sudhakarbabu, O., Nareshkumar, A., Kiranmai, K., and Lokesh, U. (2014). Overexpression of horsegram (Macrotyloma uniflorum Lam. Verdc.) NAC transcriptional factor (MuNAC4) in groundnut confers enhanced drought tolerance. Mol. Biotechnol. 56, 758–769. doi: 10.1007/s12033-014-9754-0
Pérez, R. M., Vives, V., Zandalinas, S. I., López, M. F., Muñoz, V., and Gómez, A. (2013). Biotechnological approaches to study plant responses to stress. Biomed Res. Int. 2013, 654120. doi: 10.1155/2013/654120
Qin, L. J., Song, L. L., Zhao, D., and Zhao, D. G. (2015). Overexpression of Nicotinan tabacum high-affinity potassium ion transporter protein gene (HAK1) improves the salt-tolerance in tobacco (N. tabacum) plants. J. Agric. Biotechnol. 23, 1576–1587. doi: 10.3969/j.issn.1674-7968.2015.12.006
Rui, B. H., Guang, Q., Ying, Z. K., De, J. K., Qian, G., and Gong, K. Z. (2010). Comprehensive analysis of NAC domain transcription factor gene family in Populus trichocarpa. BMC Plant Biol. 10, 145. doi: 10.1186/1471-2229-10-145
Ruiz-Lozano, J. M., Porcel, R., Azcon, C., and Aroca, R. (2012). Regulation by arbuscular mycorrhizae of the integrated physiological response to salinity in plants: new challenges in physiological and molecular studies. J. Exp. Bot. 63, 4033–4044. doi: 10.1093/jxb/ers126
Saidi, N. M., Mergby, D., and Brini, F. (2017). Identification and expression analysis of the NAC transcription factor family in durum wheat (Triticum turgidum L. ssp. durum). Plant Physiol. Biochem. 112, 117–128. doi: 10.1016/j.plaphy.2016.12.028
Srivastava, R., Kobayashi, Y., Koyama, H., and Sahoo, L. (2022). Overexpression of cowpea NAC transcription factors promoted growth and stress tolerance by boosting photosynthetic activity in Arabidopsis. Plant Sci. 319, 111251. doi: 10.1016/j.plantsci.2022.111251
Sun, H., Hu, M. L., Li, J. Y., Chen, L., Li, M., Zhang, S. Q., et al. (2018). Comprehensive analysis of NAC transcription factors uncovers their roles during fiber development and stress response in cotton. BMC Plant Bio. 18, 150. doi: 10.1186/s12870-018-1367-5
Thirumalaikumar, V. P., Devkar, V., Mehterov, N., Ali, S., Ozgur, R., Turkan, I., et al. (2018). NAC transcription factor JUNGBRUNNEN1 enhances drought tolerance in tomato. Plant Biotechnol. J. 16, 354–366. doi: 10.1111/pbi.12776
Wan, F. X., Gao, J., Wang, G. L., Niu, Y., Wang, L. Z., Zhang, X. G., et al. (2021). Genome-wide identification of NAC transcription factor family and expression analysis of ATAF subfamily members under abiotic stress in eggplant. Sci. Hortic. 289, 110424. doi: 10.1016/j.scienta.2021.110424
Wang, J. F., Lian, W. R., Cao, Y. Y., Wang, X. Y., Wang, G., Qi, C. D., et al. (2018a). Overexpression of BoNAC019, a NAC transcription factor from Brassica oleracea, negatively regulates the dehydration response and anthocyanin biosynthesis in Arabidopsis. Sci Rep. 8, 1–15. doi: 10.1038/s41598-018-31690-1
Wang, Q., Guo, C., Li, Z. Y., Sun, J. H., Deng, Z. C., Wen, L. C., et al. (2021). Potato NAC transcription factor StNAC053 enhances salt and drought tolerance in transgenic Arabidopsis. Int. J. Mol. Sci. 22, 2568–2568. doi: 10.3390/ijms22052568
Wang, W., Su, X. X., Tian, Z. P., Liu, Y., Zhou, Y. W., and He, M. (2018b). Transcriptome profiling provides insights into dormancy release during cold storage of Lilium pumilum. BMC Genom. 19, 196. doi: 10.1186/s12864-018-4536-x
Wang, Y. (2020). Study on the Stress Resistance of Tobacco of Transgenic LpNAC5 and LpNAC13 From Lilium pumilum (Master thesis). Northeast Forestry University, Harbin, China.
Wang, Y., Cao, S. J., Guan, C. J., Kong, X., Wang, Y. P., Cui, Y., et al. (2020). Overexpressing the NAC transcription factor LpNAC13 from Lilium pumilum in tobacco negatively regulates the drought response and positively regulates the salt response. Plant Physiol. Bioch. 149, 96–110. doi: 10.1016/j.plaphy.2020.01.036
Yang, X. W., He, K., Chi, X. Y., Chai, G. H., Wang, Y. P., Jia, C. L., et al. (2018). Miscanthus NAC transcription factor MlNAC12 positively mediates abiotic stress tolerance in transgenic Arabidopsis. Plant Sci. 277, 229–241. doi: 10.1016/j.plantsci.2018.09.013
Yu, J. H., Wei, W., Qing, X. S., Hao, W. C., Yu, Q. Z., Fang, W., et al. (2011). Soybean NAC transcription factors promote abiotic stress tolerance and lateral root formation in transgenic plants. Plant J. 68, 302–313. doi: 10.1111/j.1365-313X.2011.04687.x
Zhang, D., and Ma, W. H. (2019). The role of NAC transcription factors in plant response to abiotic stress. Biotechnol. Bull. 35, 144–151. doi: 10.13560/j.cnki.biotech.bull.1985.2019-0525
Zhang, S., Li, X., Fan, S., Zhou, L. J., and Wang, Y. (2020). Overexpression of HcSCL13, a Halostachys caspica GRAS transcription factor, enhances plant growth and salt stress tolerance in transgenic Arabidopsis. Plant Physiol. Biochem. 151, 243. doi: 10.1016/j.plaphy.2020.03.020
Zhang, X., Long, Y., Chen, X. X., Zhang, B. L., Xin, Y. F., Li, L. Y., et al. (2021). A NAC transcription factor OsNAC3 positively regulates ABA response and salt tolerance in rice. BMC Plant Biol. 21, 546. doi: 10.1186/s12870-021-03333-7
Zhao, F., Maren, N. A., Kosentka, P. Z., Liao, Y. Y., Lu, H., Duduit, J. R., et al. (2021). An optimized protocol for stepwise optimization of real-time RT-PCR analysis. Hortic. Res. 8, 179. doi: 10.1038/s41438-021-00616-w
Zhou, K. Y. (2020). Transcriptome Analysis and Research of Related Gene Function of Starch Accumulation in Soybean Chloroplast Under Salt Stress (Master thesis). Zhejiang University, Hangzhou, China.
Zhu, G. X., and Zhu, Q. D. (1999). Advances in the research on the effects of NaCl on photosynthesis. Chin. Bull. Bot. 16, 332–338.
Keywords: Lilium pumilum, LpNAC17, salt stress, transcription factor, ornamental plant
Citation: Wang Y, Cui Y, Liu B, Wang Y, Sun S, Wang J, Tan M, Yan H and Zhang Y (2022) Lilium pumilum stress-responsive NAC transcription factor LpNAC17 enhances salt stress tolerance in tobacco. Front. Plant Sci. 13:993841. doi: 10.3389/fpls.2022.993841
Received: 14 July 2022; Accepted: 15 August 2022;
Published: 02 September 2022.
Edited by:
Milan Kumar Lal, Central Potato Research Institute (ICAR), IndiaReviewed by:
Arvind Kumar Dubey, University of Nebraska, United StatesRajesh Singhal, Indian Grassland and Fodder Research Institute (ICAR), India
Copyright © 2022 Wang, Cui, Liu, Wang, Sun, Wang, Tan, Yan and Zhang. This is an open-access article distributed under the terms of the Creative Commons Attribution License (CC BY). The use, distribution or reproduction in other forums is permitted, provided the original author(s) and the copyright owner(s) are credited and that the original publication in this journal is cited, in accordance with accepted academic practice. No use, distribution or reproduction is permitted which does not comply with these terms.
*Correspondence: Yanni Zhang, dGRjcXRnenlAMTI2LmNvbQ==
†These authors have contributed equally to this work