- Metagenomic Laboratory, School of Biotechnology, University of Jammu, Jammu, India
Fusarium oxysporum has been reported to be the most devastating pathogen of Crocus sativus L., a commercially significant crop that yields the saffron spice. However, most of the pathogen isolations have been done from the diseased tissue, mostly from rotten corms, but no study has been conducted on diseased saffron fields. To fill the knowledge gap, the current study was carried out with the intention of recording the diversity of cultivable fungus species from saffron fields and screening them for pathogenicity towards saffron. The three study locations in Jammu and Kashmir, Srinagar (Pampore), Kishtwar, and Ramban, yielded a total of 45 fungal isolates. The internal transcribed spacer (ITS) of rDNA was used for the molecular identification. ITS rDNA-based sequence analysis classified all the operational taxonomic units (OTUs) into two phyla—Ascomycota (88.88%) and Mucoromycota (11.11%). Moreover, Fusarium (57.77%), Geotrichum (17.77%), Mucor (11.11%), Aspergillus (4.44%), Trichoderma (4.44%), Galactomyces (2.22%), and Colletotrichum (2.22%) all had different total abundances at the genus level. It was discovered that the saffron fields in Srinagar have fewer varied fungal species than the other two selected sites. All of the fungal isolates isolated including Fusarium solani, Aspergillus flavus, Trichoderma harzianum, Fusarium neocosmosporiellum, and Mucor circinelloides were pathogenic according to the pathogenicity test; however, injury to the saffron plant was found to be a must. These fungi were pathogenic in addition to F. oxysporum, which is well documented as a major cause of saffron corm rot diseases in Srinagar, but in the present study, injury was a must for F. oxysporum as well. The percentage disease severity index for both saffron roots and corms varied for each fungal isolate.
1 Introduction
Corm rot disease is reported to be the major biotic stress of Crocus sativus (saffron), resulting in severe yield loss (Cardone et al., 2020; Kothari et al., 2021; Kumar et al., 2022a). Though various bacterial and fungal pathogens are reported to cause corm rot (Gupta et al., 2021), Fusarium oxysporum is reported to be the most devastating (Palmero et al., 2014; Husaini and Jiménez, 2022). After the literature survey, we discovered various reports that are the basis for making F. oxysporum: the report in Italy is about 28 years old (Cappelli et al., 1991), that in Japan is 68 years old (Yamamoto et al., 1954), that in India is 38 years old (Shah and Srivastava, 1984), and that in Spain is 35 years old (Garcia-Jimenez and Alfaro Garcia, 1987). Recently, several other fungal species belonging to genera Fusarium, Rhizoctonia, Penicillium, Aspergillus, Sclerotium, Phoma, Stromatinia, Cochliobolus, and Rhizopus have been reported to be associated with saffron diseases (Gupta et al., 2021; Mirghasempour et al., 2022b), but most of the reports suggest F. oxysporum as the major pathogen. In our earlier report, after isolating the fungi from the rotten corm, we also found that out of the three fungi isolated, F. oxysporum strain R1 was the most severe pathogen and it was different than the commonly reported F. oxysporum f. sp. gladioli (Gupta and Vakhlu, 2015). We realized that there is no recent survey on the major cause of the corm rot causing pathogens, and in some of the reports, the pathogen has been assigned the formae speciales without any molecular phylogeny (Gupta et al., 2011). Identification and characterization of the pathogen on the basis of the host and without molecular phylogeny is not acceptable (Bhunjun et al., 2021). Another thing that caught our attention was that the pathogens were isolated from the diseased corms and no pathogen was isolated from the diseased fields and tested for pathogenicity. Moreover, in the last 3 years, nobody has reported F. oxysporum, neither from rotten corms nor from the soil, as a pathogen, let alone as the most devastating pathogen (Shuwen et al., 2019; Muñoz et al., 2020; Zhang et al., 2020; Mirghasempour et al., 2022a, Mirghasempour et al., 2022b).
While unraveling the mycobiome of underground parts of saffron by metagenomics, it was found that the saffron cormosphere is dominated by Basidiomycota during the flowering stage, Zygomycota during the dormant stage in cormosphere, and Ascomycota during the vegetative stage (Ambardar et al., 2016; Bhagat et al., 2021). However, Ambardar et al. (2016) have reported that the Ascomycota is dominant in mycobiome of bulk soil. With this background, the present study aimed at unraveling the cultivable fungal diversity in traditional and non-traditional saffron cultivating fields in Jammu and Kashmir, the only region in India where saffron is cultivated at a commercial level (Ganaie and Singh, 2019; Rather et al., 2022). Furthermore, the fungal isolates were screened for pathogenicity to saffron corms and roots. In particular, the objectives of this study were as follows: (1) to collect fungal isolates from traditional and non-traditional saffron fields, (2) to evaluate the pathogenicity of the isolated fungal species against saffron, and (3) to check in real time if F. oxysporum is the most severe pathogen causing rot in saffron, with an aim to recommend the strategy for yield increase.
2 Materials and methods
2.1 Soil sampling, study sites, and soil analysis
Soil samples were collected in the month of November 2018 from the three sampling sites—traditional saffron fields: Srinagar (Pampore)-34.02°N 74.93°E and Kishtwar-33.32°N 75.77°E and a non-traditional saffron field: Ramban-33.25°N 75.25°E (Figure 1). For each sampling site, soil samples were collected randomly from different spots, homogenized, and appropriately mixed to form one sample. The soil samples of three locations were sieved to remove the non-soil components such as plant and animal leftovers and pebbles. Each soil sample was then divided into three major parts: (i) the first part of the soil was preserved at −20°C, (ii) another part was used for the determination of the soil’s physio-chemical properties, and (iii) the last fraction of each soil sample was preserved at 4°C for the culture-dependent fungal communities’ analysis. The soil sample of three geographic locations was sent to Yara Fertilisers India Pvt. Ltd. Analysis for the analysis of standard physio-chemical parameters of soil. The “Standard” soil analysis included analysis and interpretation of the parameters–pH, electrical conductivity (EC, mmhos/cm), organic carbon (OC, %), nitrogen (N, kg/ha), phosphorus (P, kg/ha), potassium (K, kg/ha), sulfur (S, ppm), zinc (Zn, ppm), and boron (B, ppm). The results have been generated by Yara’s Megalab™ software.
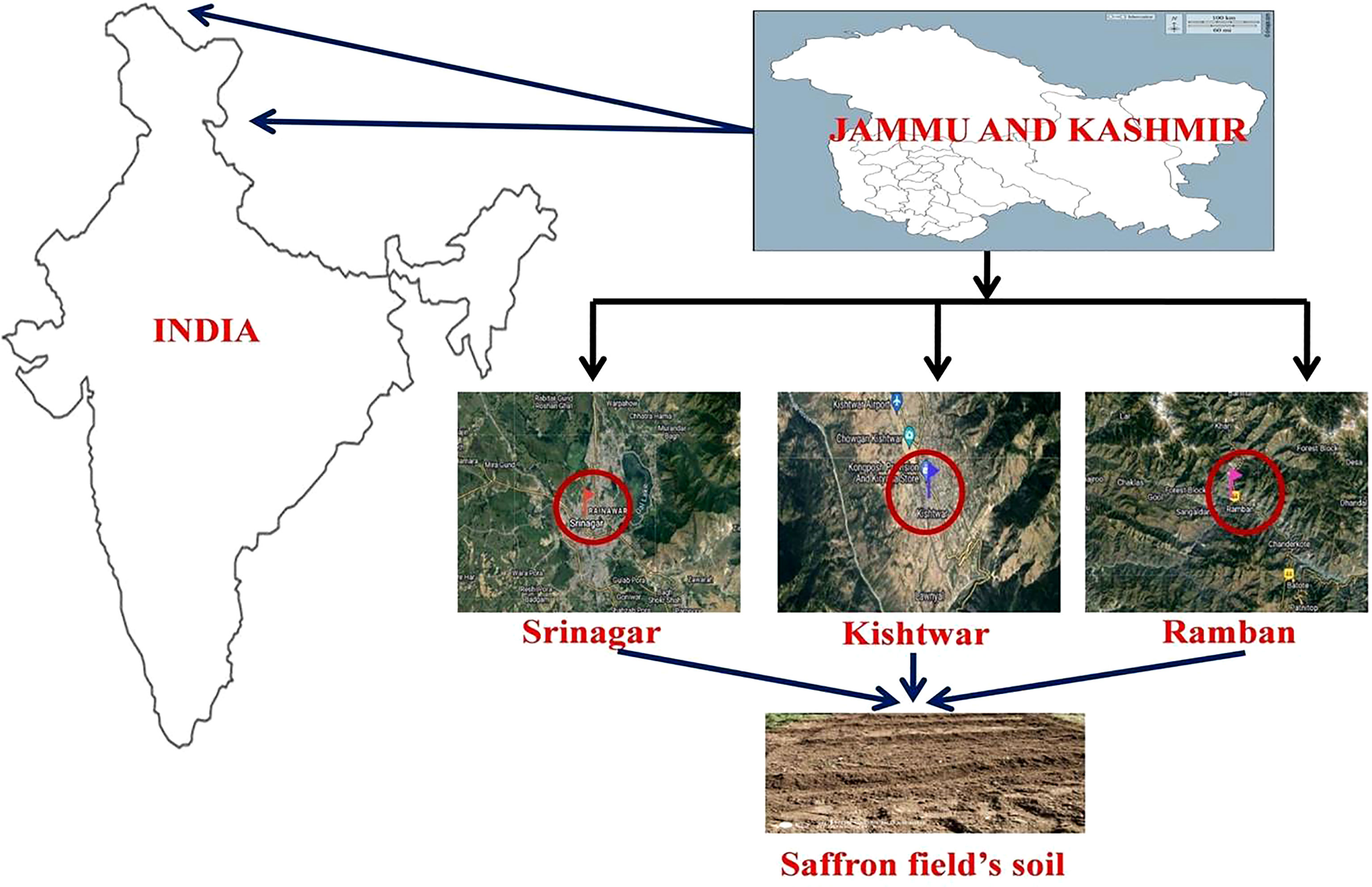
Figure 1 Picture representing the geographical regions (Srinagar, Kishtwar, and Ramban) of saffron fields from where soil samples were collected.
2.2 Isolation of fungi from the saffron fields
The isolation of fungi was done from all the three location soils, so as to identify the inhabitant fungus up to species/strain level. The serial dilution plating method was carried out for the dilution of soil samples as mentioned by Waksman (1992), with the purpose of avoiding overcrowding of fungal colonies in the soil in each dilution (Waksman, 1992). Two replicates were used for each dilution of each 1 g of soil sample up to 10−12 dilution. One hundred microliters (0.1 ml) of each dilution was plated on four different media: (1) Potato Dextrose Agar (PDA), (2) Czapek Dox Agar (CDA), (3) Sabouraud Dextrose Agar (SDA), and (4) Peptone pentachloronitrobenzene agar media (PPA). Chloramphenicol (25 mg/ml) was added to all media before pouring into Petri plates for preventing bacterial growth. The Petri plates were then incubated at 28 ± 2°C for 4–7 days. Each colony type thus obtained was transferred to a new PDA plate for macroscopic, morphological, and molecular identification. The maintenance of pure cultures was done by sub-culturing by incubating the plates at 28 ± 2°C.
2.3 DNA extraction and soil fungal isolate identification
The genomic DNA was extracted according to the method of Saghai-Maroof et al. (1984). The fresh mycelium of fungal cultures grown on PDA at 25°C for 7 days was used for DNA isolation. For the preliminary identification of the fungal colonies, the primer pair ITS1/ITS4—ITS1 (5′-TCCGTAGGTGAACCTGCGG-3′) and ITS4 (5′-TCCTCCGCTTATTGATATGC-3′)—was used for amplification and sequencing of the ITS region (Gupta and Vakhlu, 2015). The 10-μl PCR reaction contained the following: 5.3 μl of nuclease-free water (HIMEDIA, ML064), 1 μl of Kappa Taq buffer (10X), 0.1 μl of MgCl2 (25 mM), 0.4 μl of dNTPs (10 mM) (Promega U1518), 0.2 μl of Kappa Taq (5 U/μl) (KAPA Taq PCR KIT, KK1015), 1 μl of each primer (10 μM), and 1 μl (1,000 ng/μl) of each fungal DNA sample. The amplification was performed as follows: denaturation at 95°C for 5 min, followed by 35 cycles of 95°C for 30 s, annealing at 55°C for 1 min, and extension at 72°C for 1 min, with a final extension at 72°C for 7 min. The PCR amplicons were verified on 1% agarose gel electrophoresis by loading 4 µl of the PCR product and 2 µl of a reference ladder (0.5 μg/μl). The PCR products were outsourced for Sanger sequencing to Biologia Research Pvt. Ltd. (Haryana, India) and sequences were analyzed by BLAST (Basic Local Alignment Search Tool) (https://blast.ncbi.nlm.nih.gov/Blast.cgi).
2.4 Diversity analysis for fungal isolates
The alpha diversity was calculated as species richness and was measured for all the three sampling locations. The species richness was defined as the total number of species isolated from the particular soil location. The formula H′ = − Σ(Pi × InPi), where Pi denotes the proportional abundance of fungal isolates in a specific location, was used to calculate Shannon’s H′ diversity index (Ricotta, 2002). The relative abundance was estimated as a percentage of a fungal isolate belonging to a specific species/group as compared to the total number of isolates (Jan et al., 2022). All the diversity analyses were performed using PAST 3.04 software (Hammer et al., 2001).
2.5 Pathogenicity tests
2.5.1 Preparation of saffron corm for the pathogenicity test
The pathogenicity test of 45 fungal isolates on the saffron plant was conducted in pots. F. oxysporum R1 (Fox R1) earlier reported as a pathogenic strain to saffron corms was used as a reference point for pathogenicity (Gupta and Vakhlu, 2015). For negative control, plant inoculation with autoclaved distilled water was used. Healthy corms were disinfected by dipping them in 5% of sodium hypochlorite solution for 15 min. This was followed by three washes with autoclaved distilled water. The corms were planted in sterile plastic pots (17 cm diameter) containing two-thirds autoclaved sand and soil at a ratio of 1:1 (Palmero et al., 2014). The test was conducted in five different combinations with three replicates of each, and the combinations are tabulated in Table 1 for easy comprehension. For the corm injury, the apical end of corm was injured with autoclaved pipette tip (HIMEDIA, Pipette tips, Neutral color, 0.5–10 μl—CG308-1x1000NO) causing a 10-mm-deep and 2-mm-wide injury (Bhagat et al., 2022). For root injury, the tips of roots were cut 1 cm from the terminal end with sterile scissors.
2.5.2 Preparation of fungal inoculums for the pathogenicity test
The fungi inoculums were grown in potato dextrose broth at 27°C with constant shaking at 150 rpm in the incubator (ORBITEK). The inoculums were prepared by filtering 7-day-old fungal culture through double-layer cheesecloth. This was done to remove the mycelium from the inoculums to be used. For each isolate, the conidia suspension was maintained at 107 conidia/ml. One milliliter of each conidia suspension was mixed with the sand–soil combination in the pots and left for 1 month with controlled light and temperature conditions (12/12 h light/dark; 25/21°C) (Gupta and Vakhlu, 2015; Wani et al., 2016). Plants were watered regularly during this period to keep the soil–sand mixture moist. The presence and absence of rot symptoms on corm and root were evaluated after 10, 20, and 30 days of inoculation, respectively.
The pathogenicity and capacity of each isolate to cause rot symptoms on roots and corms were demonstrated according to Avan et al. (2021). The roots were assessed according to the rot symptoms defined on a 0–3 scale: 0 = no disease, 1 = one-third of the roots are necrotic, 2 = two-thirds of the roots are necrotic, 3 = all of the roots are necrotic. The corm rot symptoms were estimated by cutting the corm in half along their longer axes and two perpendicular diameters of internal lesions were determined. The mean of two diameters represented the lesion size of each corm (Azil et al., 2021). The rating index used for the evaluation of corm rotting was recorded according to the following: 0 = no symptoms of corm rot, 1 = rotting near point of injury, 2 = rotting inside the corm, 3 = rotting both inside and outside the corm, and 4 = whole corm rot (Wani et al., 2016). The disease severity index (DSI) values were calculated for each fungal isolate using the following formula as per Avan et al. (2021).
DSI—disease severity index NS—number of seedlings at each scale
SV—scale value HSV—the highest scale value
TS—total seedling evaluated
The percent disease severities were estimated by multiplying the value of disease severity index with 100. The pathogenicity profiling of fungal isolates was grouped into four categories: NP—non-pathogenic (below 40% disease severity); LP—low pathogenic (41%–60% disease severity); MP—moderately pathogenic (61%–80% disease severity); and HP—highly pathogenic (81%–100% disease severity). For the final evaluation of symptoms, 10, 20, and 30 days post-inoculation (dpi), five plants were chosen for five injury treatments in triplicates for each isolate (5 × 3 = 15 plants in total), and the experiment was conducted twice. Tissue sections with symptoms were used for the re-isolation of pathogens, and to confirm Koch’s postulates, recovered isolates were compared to primarily inoculated pathogens.
2.6 Statistical analysis
For corm rot pathogenicity results, the lesion sizes of every individual corm were analyzed statistically. The recorded data were subjected to one-way analysis of variance (ANOVA) using IBM SPSS statistics version 26. The significance of mean differences in lesion sizes was observed using Tukey’s test at 5% level of threshold significance. The data presented in Figures 2A–C depict mean values ± standard deviation (SD) for diameter of corm rot. In Figure 2, the mean diameter in the same day bar followed by lowercase letter(s) represents significant difference at p ≤ 0.05, according to Tukey’s test. The single asterisk (*) represents significant difference at p< 0.05, the double asterisk (**) represents significant difference at p< 0.01, and the triple asterisk (***) represents significant difference at p< 0.001; NS represents no significant difference.
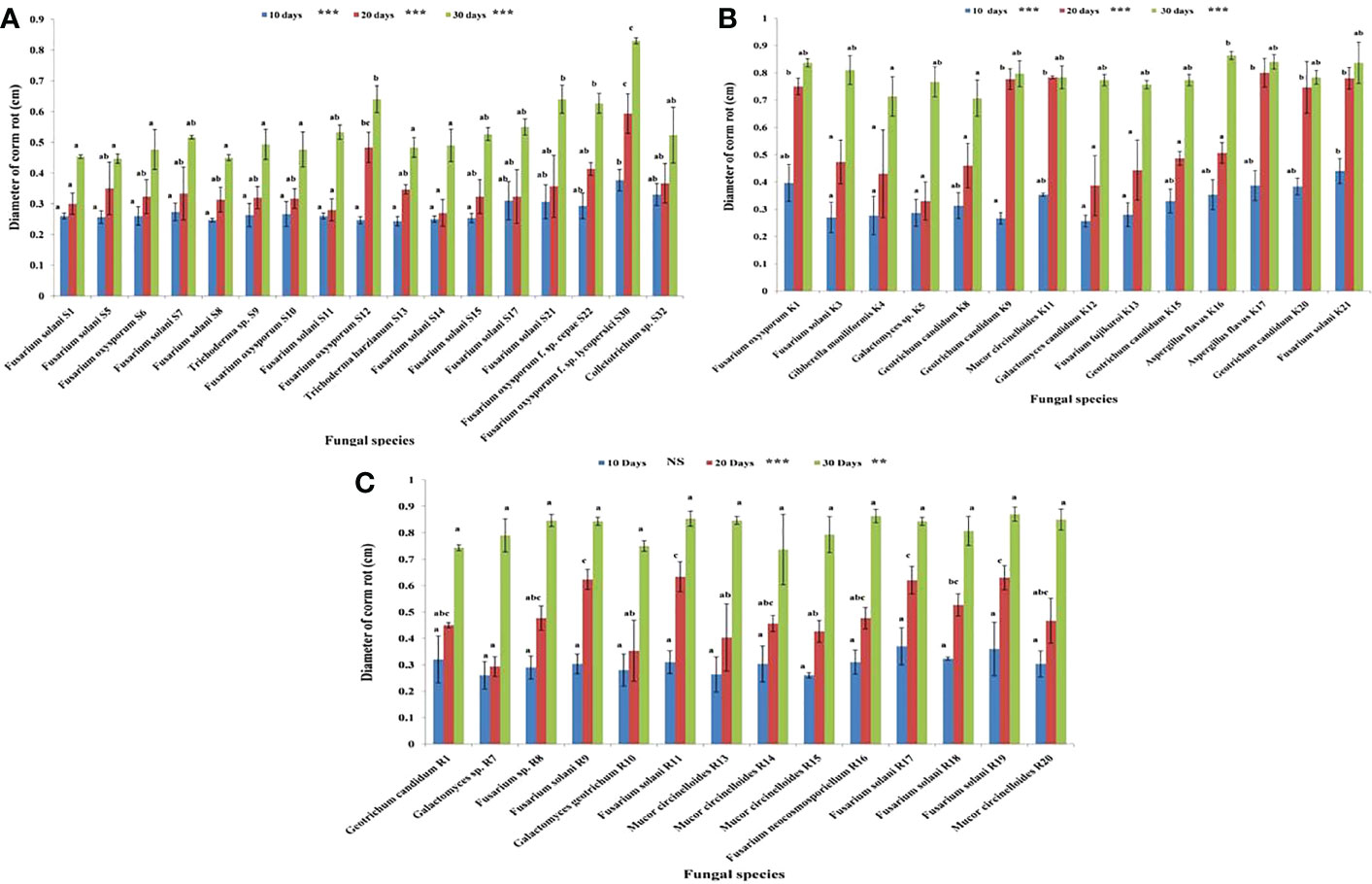
Figure 2 (A) Diameter of corm rot (in cm) caused by fungal species isolated from Srinagar soil at 10, 20, and 30 days post-inoculation. (B) Diameter of corm rot (in cm) caused by fungal species isolated from Kishtwar soil at 10, 20, and 30 days post-inoculation. (C) Diameter of corm rot (in cm) caused by fungal species isolated from Ramban soil at 10, 20, and 30 days post- inoculation. Mean diameters in the same day bar followed by lowercase letter(s) are significantly different at p ≤ 0.05, according to Tukey’s test. *, significance at p< 0.05; **, significance at p< 0.01; ***, significance at p< 0.001; NS, no significant difference.
3 Results
3.1 Soil analysis and diversity of fungal isolates
The location and various physio-chemical properties of the soil of the sampling sites are presented in Table 2. The soil of all the selected locations has an alkaline pH ranging from 7.6 to 8.5. The Srinagar (Pampore) soil has the highest pH of 8.5; however, the EC, OC, N, P, K, S, Zn, and B were comparatively lower than the other two locations, i.e., Kishtwar and Ramban. The EC, OC, S, Zn, and B contents were highest in Ramban soil, whereas the other soil contents such as N, P, and K were highest in the Kishtwar soil. A total of 45 fungal isolates were isolated from saffron fields across three different study locations (Figure 1). Based on ITS rDNA gene sequences followed by BLASTn analysis, these isolates were resolved into 45 operational taxonomic units (OTUs) distributed across seven genera, i.e., Fusarium, Geotrichum, Mucor, Aspergillus, Galactomyces, Trichoderma, and Colletotrichum. Majority of these fungal isolates represented >99% similarity with the reference strains. The ITS sequences have been deposited in GenBank, and accession numbers have been tabulated in Table 3. The phylogenetic relationship of 45 OTUs corresponded to a broad taxonomic range. The phylogenetic topology revealed that Ascomycota and Mucoromycota were the two phyla into which the entire representative isolates could be grouped (Supplementary Figure 1). Ascomycota was predominant, accounting for 88.88% of total OTUs, followed by Mucoromycota at 11.11%. Fusarium, a member of the Ascomycota phylum, was the most prevalent genus among all the identified genera distributed across these two phyla, accounting for 57.77% of all OTUs. Geotrichum, a genera of Ascomycota phylum, and Mucor of the phylum Mucoromycota accounted for approximately 17.77% and 11.11% of all OTUs, respectively. Both Aspergillus and Trichoderma belonging to the Ascomycota phylum were about 4.44% abundant. Out of all the seven genera, Galactomyces and Colletotrichum were the least abundant (2.22%) (Supplementary Figure 2).
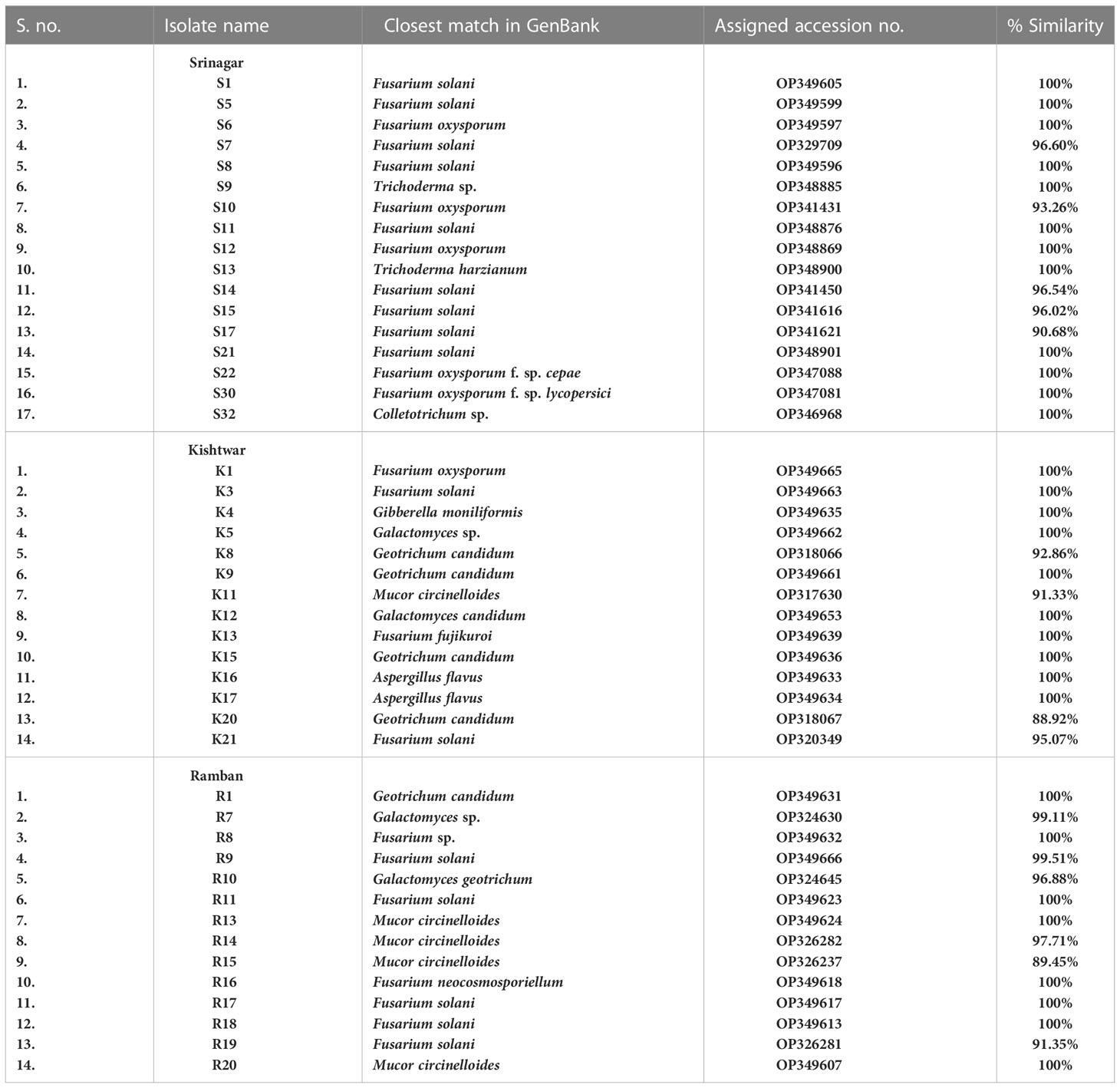
Table 3 Description of the OTUs representing the fungal species isolated from the three geographic locations of saffron fields (Srinagar, Kishtwar, and Ramban).
3.2 Diversity and distribution of fungal isolates across different locations
Among different locations, Pampore in Srinagar harbored the highest number of fungal isolates, i.e., 17, followed by Kishtwar and Ramban with 14 isolates each. The different location sites yielded variable results vis-a-vis the genera of fungal isolates. OTUs belonging to Ascomycota constituted the most abundant fungal isolates in all the three locations followed by Mucoromycota, but it was absent in Srinagar (Figure 3). The four genera present in Kishtwar soil were Fusarium, Geotrichum, Mucor, and Aspergillus. In Ramban soil, Fusarium,Geotrichum, Mucor, and Galactomyces were the four genera observed. Notably, only three genera, Fusarium, Trichoderma, and Colletotrichum, were isolated from Srinagar soil (Figures 4A–C). The four genera Geotrichum, Aspergillus, Mucor, and Galactomyces were absent in Srinagar but were isolated from the other two locations. The genera Trichoderma and Colletotrichum were exclusively isolated from Srinagar soil. However, Aspergillus was found only in Kishtwar. The genus Fusarium was present in all the three locations. Srinagar reported highest OTUs of Fusarium genera, i.e., 82.35% out of all the OTUs observed in Srinagar, followed by Trichoderma and Colletotrichum, accounting for 11.76% and 5.88% OTUs, respectively. Geotrichum and Fusarium were found to be abundant, i.e., 42.85% and 35.71% OTUs out of all the fungal isolates isolated from Kishtwar soil. However, Aspergillus and Mucor were lower in percentage, i.e., 14.28% and 7.14%, respectively, in Kishtwar. In Ramban, Fusarium and Mucor were the highly representative genera, with 50.00% and 28.57% OTUs present, respectively, of all the OTUs obtained in the Ramban region. The other two genera, namely, Geotrichum and Galactomyces, were 14.28% and 7.14% abundant, respectively (Figures 4A–C).
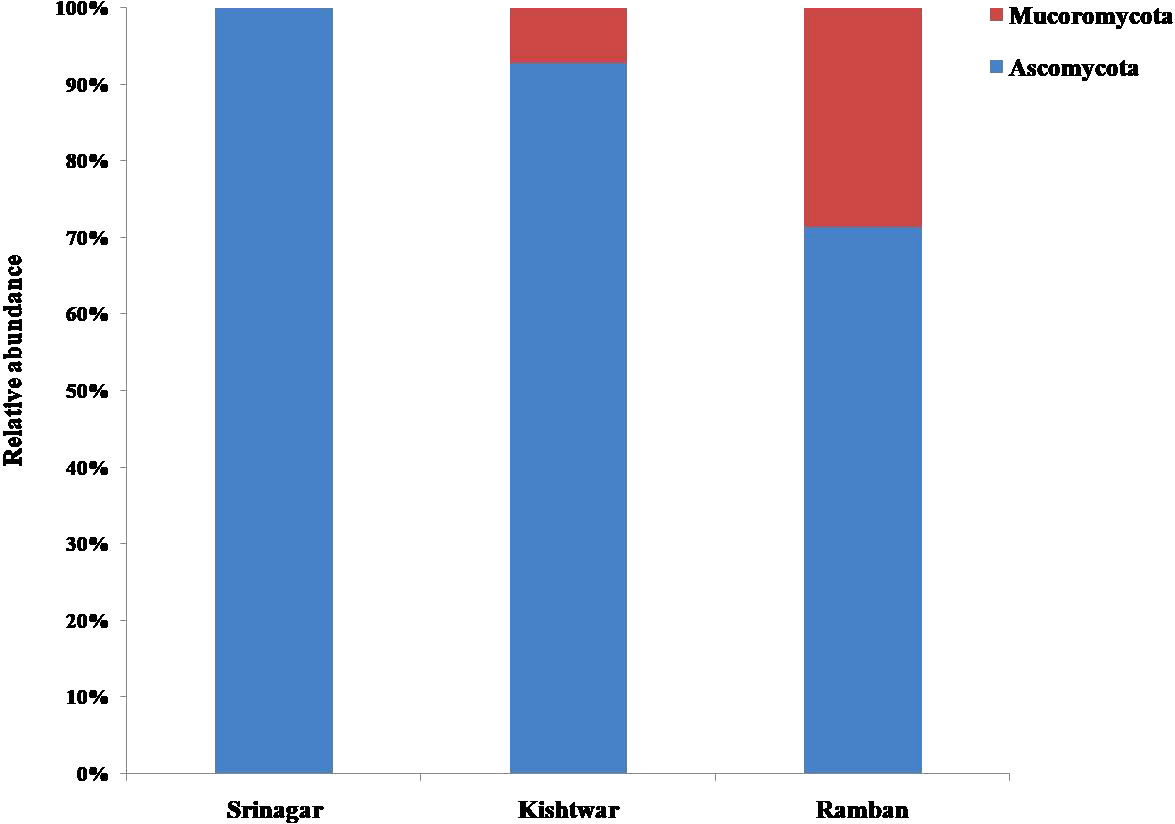
Figure 3 Relative abundance of individual operational taxonomic units (OTUs) at the phylum level across the three locations (Srinagar, Kishtwar, and Ramban).
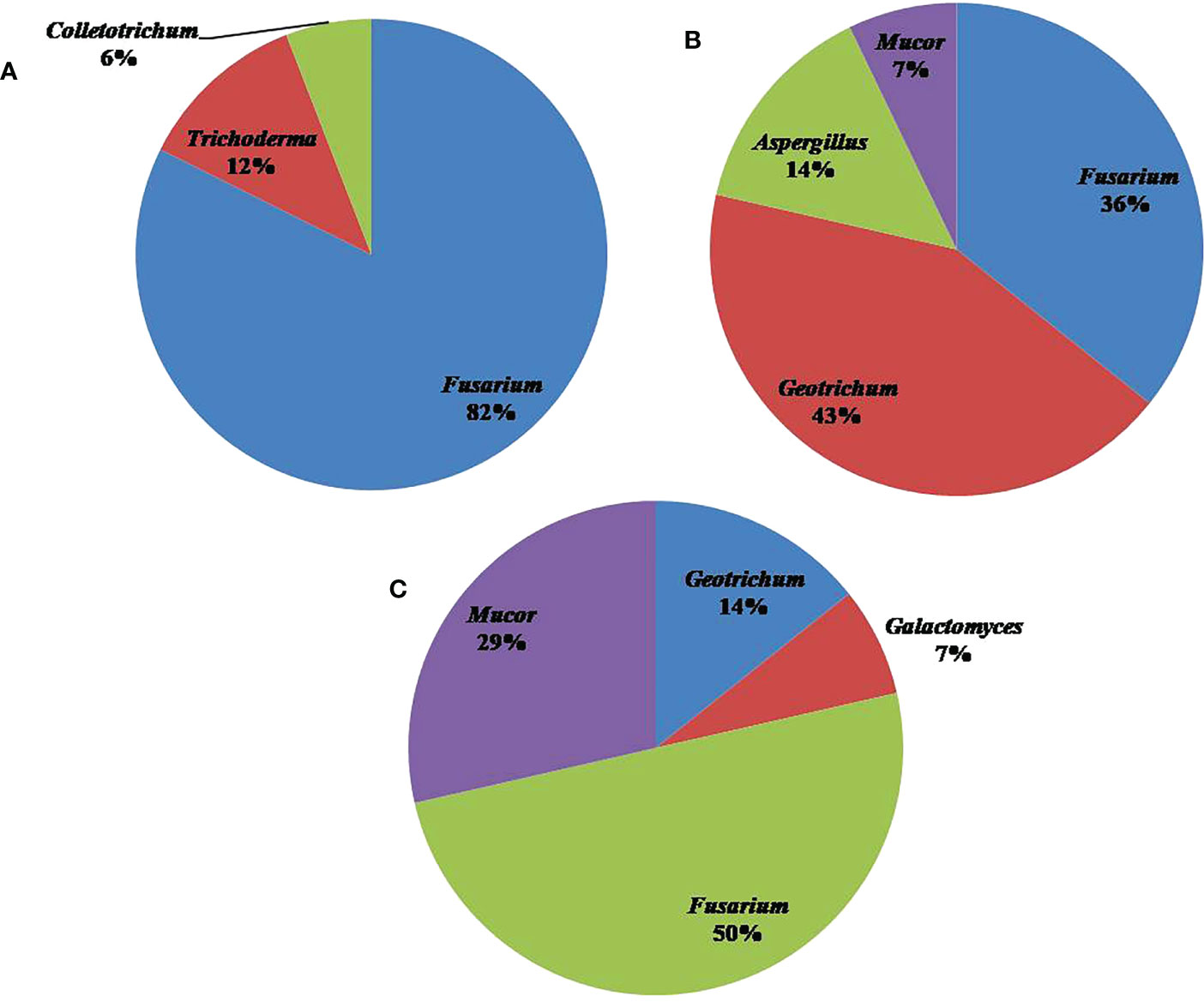
Figure 4 Pie chart representing the distribution and diversity of fungal genera in (A) Srinagar, (B) Kishtwar, and (C) Ramban.
In Kishtwar, the 14 species isolated were Geotrichum candidum (28.57%; n = 4), Fusarium solani (14.28%; n = 2), Aspergillus flavus (14.28%; n = 2), Galactomyces candidum (7.14%; n = 1), Fusarium fujikuroi (7.14%; n = 1), Mucor circinelloides (7.14%; n = 1), Galactomyces sp. (7.14%; n = 1), Gibberella moniliformis (7.14%; n = 1), and F. oxysporum (7.14%; n = 1). The species that were found in the Ramban soil were F. solani (35.71%; n = 5), M. circinelloides (28.57%; n = 4), G. candidum (7.14%; n = 1), Galactomyces sp. (7.14%; n = 1), Fusarium sp. (7.14%; n = 1), Galactomyces geotrichum (7.14%; n = 1), and Fusarium neocosmosporiellum (7.14%; n = 1). In the Srinagar soil, the fungal isolates corresponded to the following species: F. solani (52.94%; n = 9), F. oxysporum (29.41%; n = 5), Trichoderma sp. (5.88%; n = 1), Trichoderma harzianum (5.88%; n = 1), and Colletotrichum sp. (5.88%; n = 1). The graphic representation of this diversity is given in Figures 5A–C.
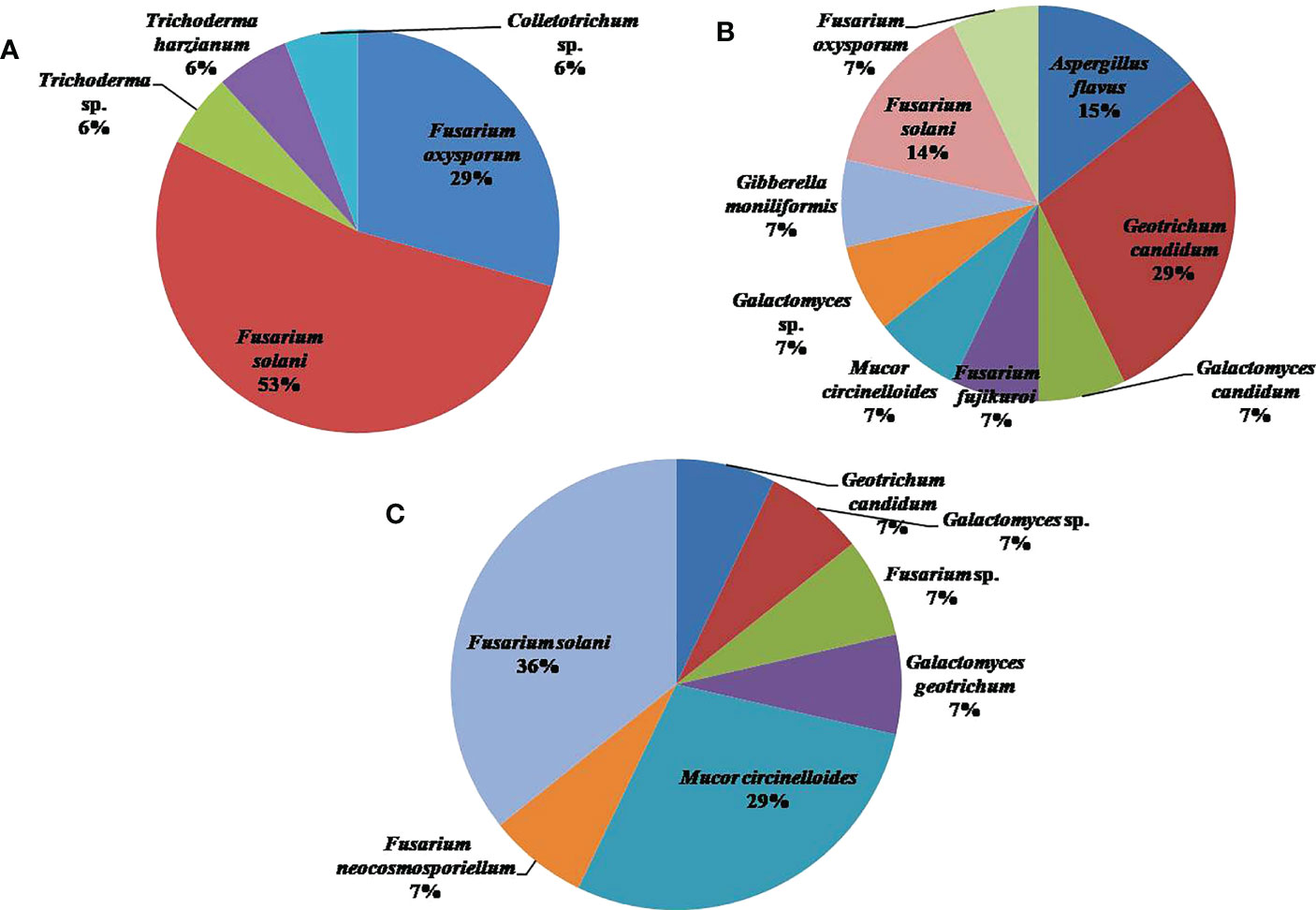
Figure 5 Pie chart representing the distribution and diversity of fungal species in (A) Srinagar, (B) Kishtwar, and (C) Ramban.
It was observed that Srinagar soil recorded the highest species richness with 17 OTUs, followed by Kishtwar and Ramban with 14 OTUs each (Figure 6A). The Shannon (H′) index was highest for the Kishtwar region (H′= 1.197), followed by Ramban (H′= 1.171). The Srinagar location had the lowest H′ index of 0.5783 (Figure 6B).
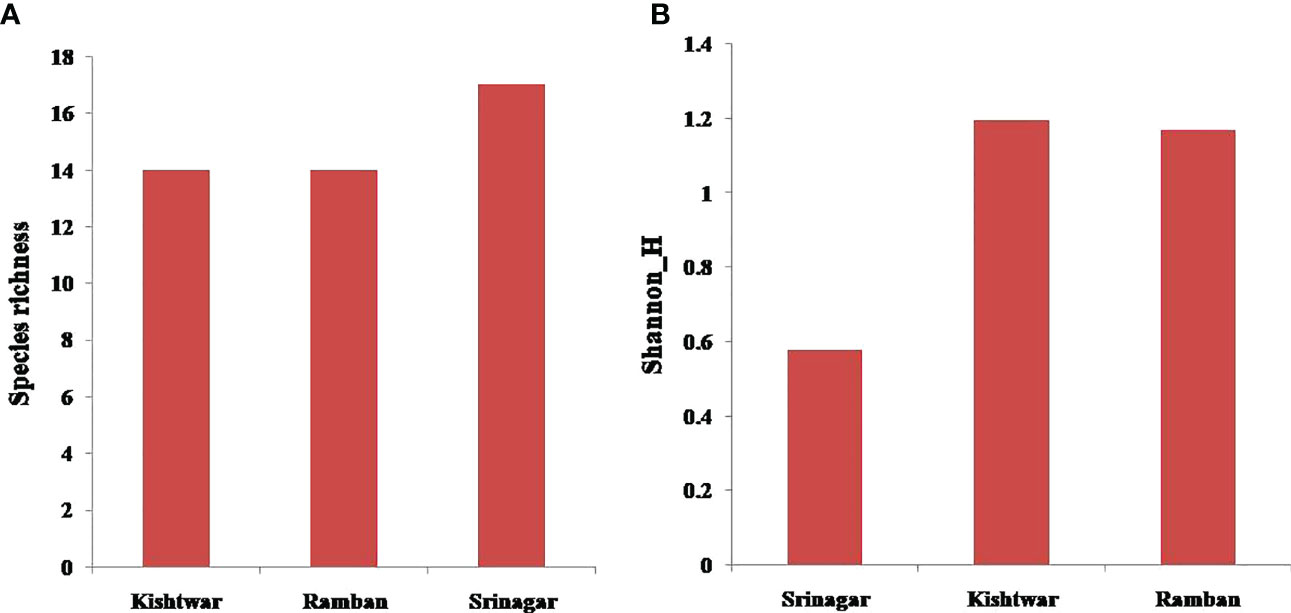
Figure 6 (A) Species richness across the three locations (Srinagar, Kishtwar and Ramban). (B) Shannon’s (H′) index across the three locations (Srinagar, Kishtwar, and Ramban).
3.3 Pathogenicity test of fungal isolates
All the fungal isolates were found to be pathogenic on saffron corms as well as on roots, but only when they were artificially injured. Non-injured and injured corm/root in the absence of fungal inoculums remained healthy and did not develop any visible symptoms during the experiment. However, the pathogenicity results of all the 45 fungal isolates had variability in the range of percent disease severity index.
3.3.1 Corms rot profiling
Pathogenicity profiling was grouped into four categories and was estimated on both corms and roots in correspondence to the point of fungal inoculation. The corm rot symptoms were observed for 1 month to follow the progress of disease symptoms after inoculation with each isolate. At 10 days post-inoculation (dpi), almost all the fungal isolates looked either non-pathogenic or low pathogenic as symptoms were invisible or mildly visible on the saffron corm. However, from 20 dpi to 30 dpi, disease severity index increased from the category of low pathogenic to moderately pathogenic. Of the Ramban soil fungal isolates, five fungal isolates were non-pathogenic, including four M. circinelloides strains and one G. geotrichum strain at 10 dpi. Conversely, nine other fungal strains such as G. candidum, Galactomyces sp., F. solani (5), Fusarium sp., and F. neocosmosporiellum were low pathogenic at 10 dpi. All these fungal isolates were moderately pathogenic at 30 dpi (up to 75% disease severity index). Similarly, all the fungal strains isolated from the Kishtwar soil were also moderately pathogenic, showing maximum of 75% disease severity index at 30 dpi to the saffron corm. In contrast to Ramban and Kishtwar, most of the fungal strains isolated from the Srinagar soil were low pathogenic at 30 dpi. F. oxysporum (3), F. solani (1), and Colletotrichum sp. (1) were the five strains among the 17 reported in Srinagar that were moderately pathogenic to the saffron corm at 30 dpi (Table 4; Figures 7A–C). F. oxysporum R1, which was considered as positive control, was non-pathogenic at 10 dpi (25% DSI); however, percentage disease severity index was increased to 50% at 20 dpi and 66.66% at 30 dpi, respectively. The three out of six F. oxysporum that were isolated in the present study were more pathogenic than F. oxysporum R1, as they exhibited 75% DSI at 30 dpi. These three F. oxysporum species were named F. oxysporum S12, F. oxysporum f. sp. lycopersici S30, and F. oxysporum K1.
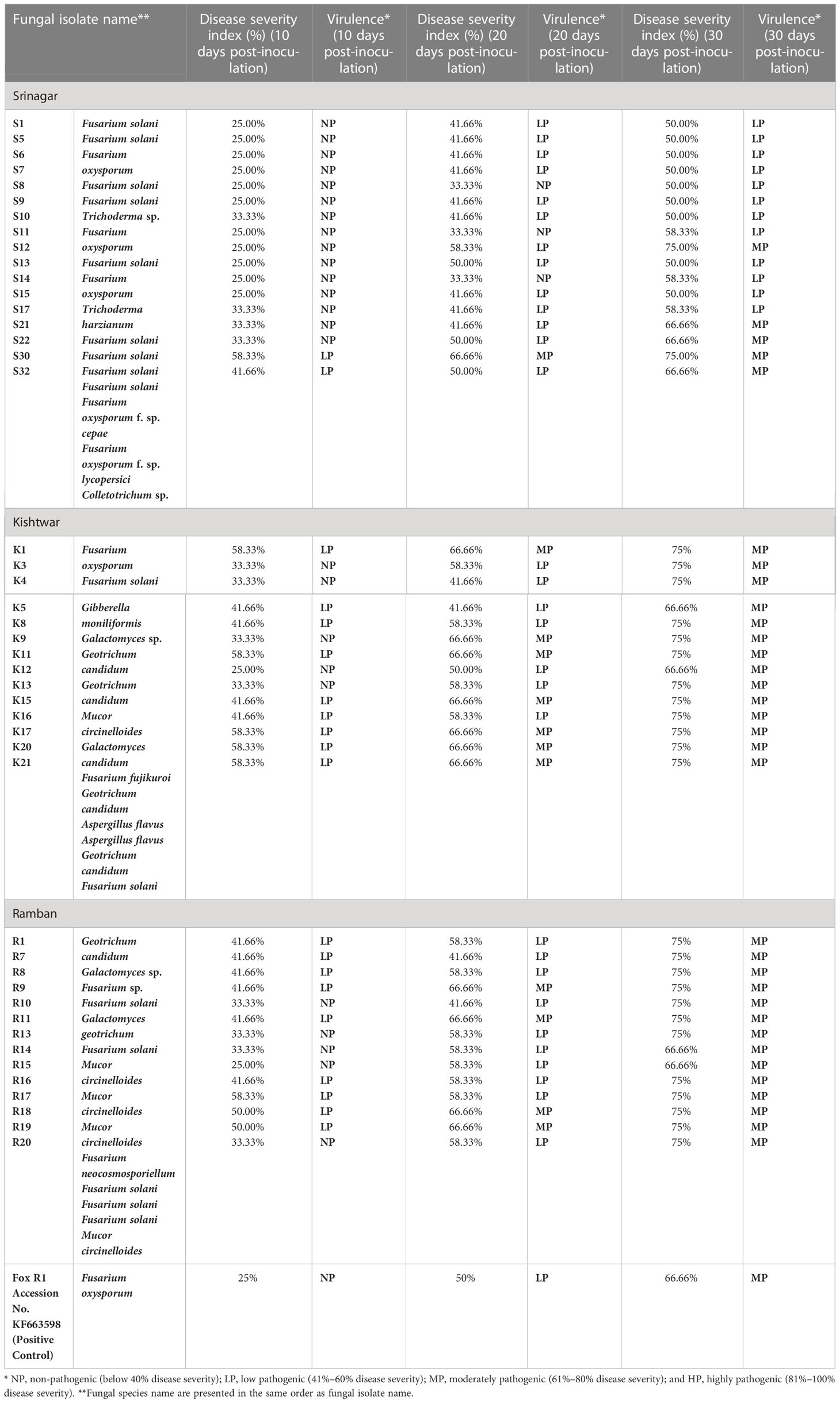
Table 4 Corm rot pathogenicity profiling of fungal species isolated from Srinagar, Kishtwar, and Ramban location.
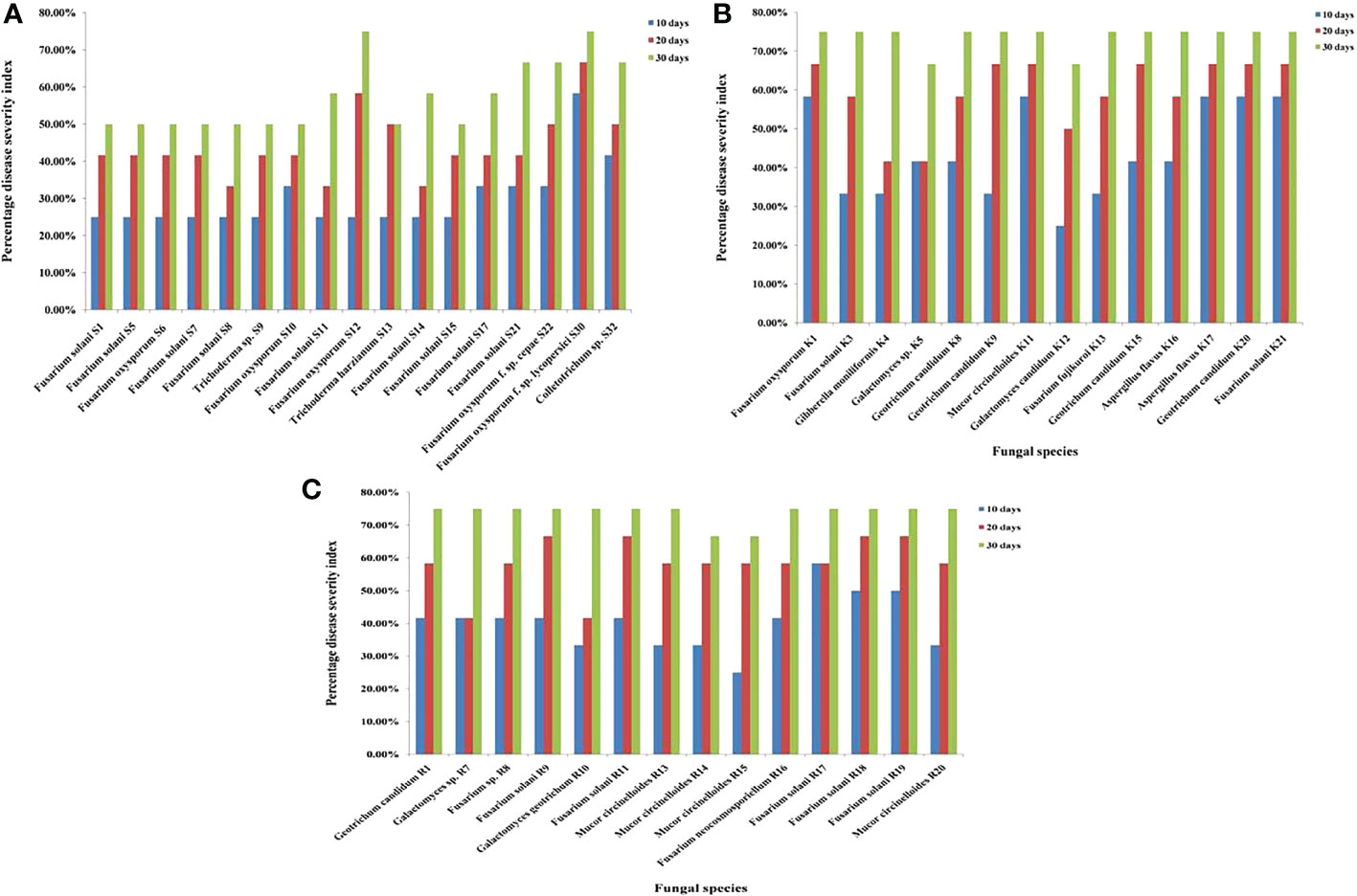
Figure 7 (A) Pathogenicity test of fungal species isolated from Srinagar soil on saffron corms at 10, 20, and 30 days post-inoculation (dpi). (B) Pathogenicity test of fungal species isolated from Kishtwar soil on saffron corms at 10, 20 and 30 dpi. (C) Pathogenicity test of fungal species isolated from Ramban soil on saffron corms at 10, 20, and 30 dpi.
The mean of lesion sizes formed by fungal species were uneven, ranging from the 0.24 cm by T. harzianum S13 to 0.44 cm by F. solani K21 at 10 dpi. Moreover, at 20 dpi, the lesion size further increased from 0.27 cm by F. solani S14 to 0.80 cm by A. flavus K17. At 30 dpi, the lesion size was observed in the range of 0.44 cm by F. solani S5 to 0.87 cm by F. solani R19. It was observed that the diameter of the lesion was in the range of 0.26–0.37 cm at 10 dpi, 0.29–0.63 cm at 20 dpi, and 0.73–0.87 cm at 30 dpi for the fungal isolates obtained from Ramban soil. However, the variable range of 0.25–0.44 cm at 10 dpi, 0.33–0.80 cm at 20 dpi, and 0.70–0.86 cm at 30 dpi was observed in case of Kishtwar. In contrast to Ramban and Kishtwar isolates, Srinagar isolates had a lesion size estimated at 0.24–0.37 cm at 10 dpi, 0.27–0.59 cm at 20 dpi, and 0.44–0.83 cm at 30 dpi (Figures 2A–C).
3.3.2 Root rot profiling
As in the case of corms, in roots as well, all the inoculated fungal isolates exhibited slight browning of roots as the initial symptoms. Eventually, the progression of infection resulted in browning, black necrotic lesions, and wilting of the roots (Figure 8). All the isolates were in non-pathogenic category at 10 dpi with the exception of F. neocosmosporiellum R16 with 44.44% disease severity, T. harzianum S13 with 44.44%, and F. oxysporum f. sp. lycopersici S30 with 44.44%. At 20 dpi, fungal isolates could be divided into a mixed proportion of non-pathogenic, low pathogenic, and moderately pathogenic categories. Highly pathogenic isolates were reported only in Ramban and Srinagar soil at 30 dpi. The four F. solani strains, namely, R9, R17, R18, and R19, had 88.88% disease severity index at 30 dpi; hence, they were highly pathogenic strains identified in Ramban soil. However, highly pathogenic strains obtained from Srinagar at 30 dpi were T. harzianum S13 (100%), F. solani S5 (100%), Trichoderma sp. S9 (88.88%), F. solani S11 (88.88%), and F. oxysporum f. sp. lycopersici S30 (88.88%) (Table 5; Figures 9A–C). The already reported F. oxysporum R1 taken as a positive control was as pathogenic as F. oxysporum f. sp. lycopersici S30 that was isolated in the present study. F. oxysporum R1 resulted in 88.88% disease severity at 30 dpi, similar to F. oxysporum f. sp. lycopersici S30. However, F. oxysporum R1 was more pathogenic to saffron roots than other F. oxysporum species isolated in the study but not as pathogenic as T. harzianum S13 that affected roots more severely, i.e., 100% DSI at 30 dpi. The pathogenicity of all the isolates was confirmed by Koch’s postulates after their re-isolation from the symptomatic tissues, and the identities of the re-isolate species were confirmed using microscopic, macroscopic, and molecular analysis fulfilling Koch’s postulates.
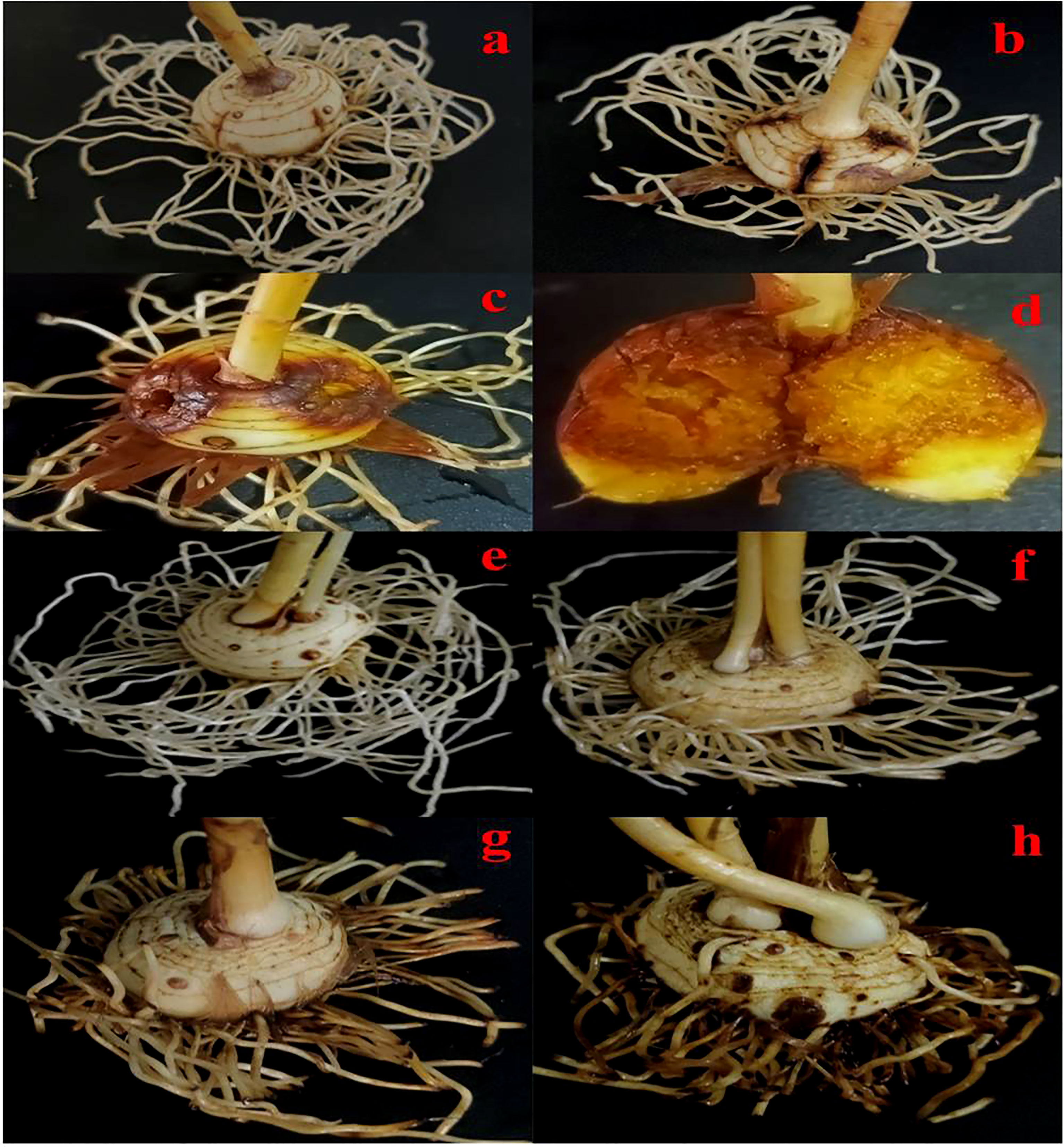
Figure 8 Symptoms of rot on saffron corms and roots caused by fungal isolates. (A) Poorly developed symptoms on corms, (B) moderate symptoms of corm rot, (C,D) severe corm rot, (E) no symptoms of saffron root rot, (F) poorly developed symptoms on roots, (G) moderate symptoms of root rot, and (H) severe root rot.
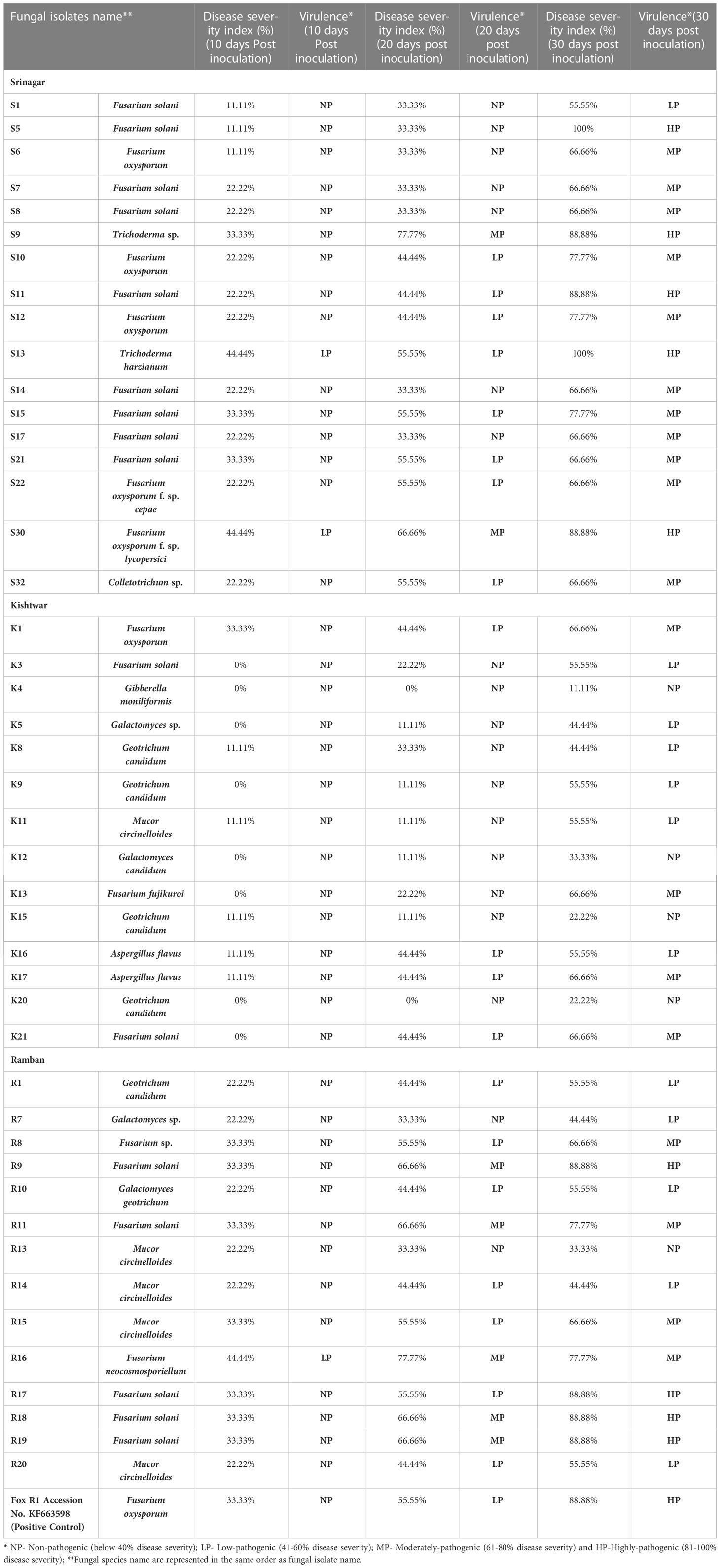
Table 5 Root rot pathogenicity profiling of fungal species isolated from Srinagar, Kishtwar and Ramban location.
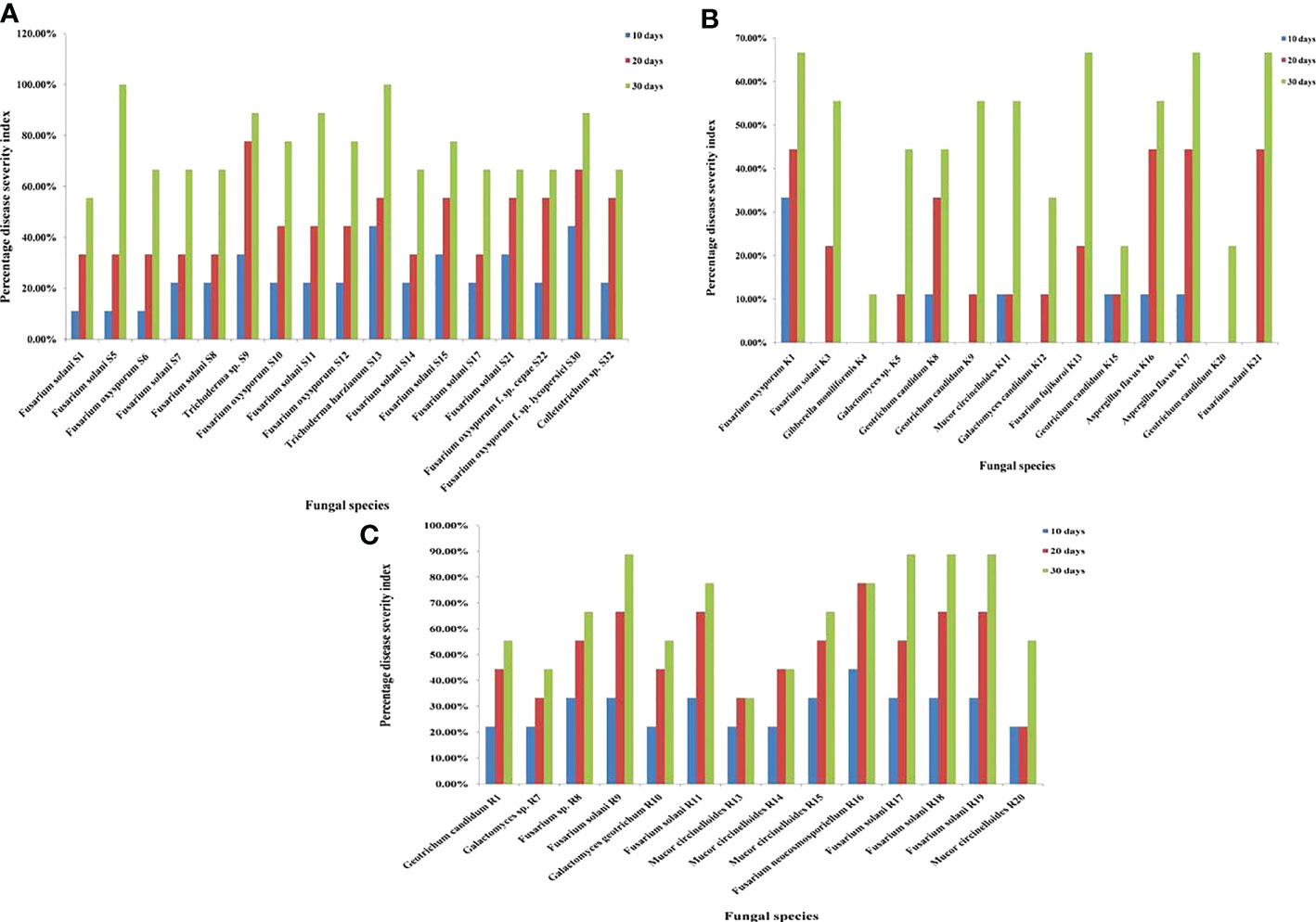
Figure 9 (A) Pathogenicity test of fungal species isolated from Srinagar soil on saffron roots at 10, 20, and 30 days post-inoculation (dpi). (B) Pathogenicity test of fungal species isolated from Kishtwar soil on saffron roots at 10, 20, and 30 dpi. (C) Pathogenicity test of fungal species isolated from Ramban soil on saffron roots at 10, 20, and 30 dpi.
4 Discussion
Previously, pathogenic fungal diversity associated with the saffron plant has been investigated on saffron corm samples including infected corms, corms that could or could not germinate, and corms that could germinate but were unable to bloom by both culture-dependent and -independent techniques (Gupta and Vakhlu, 2015; Ambardar et al., 2016; Bhagat et al., 2021; Shuwen et al., 2021; Mirghasempour et al., 2022b). Apart from the saffron corms, fungal diversity has also been estimated from roots, stems, leaves, and other tissues of saffron (El Aymani et al., 2019; Belfiori et al., 2021). As far as fungal diversity in the saffron fields is conserved, there are only two reports to our knowledge and both are from Morocco (Chamkhi et al., 2018; El Aymani et al., 2019). Therefore, the present study was initiated with an aim to unravel cultivable fungal diversity associated with saffron fields in Jammu and Kashmir in both traditional and non-traditional areas of saffron cultivation, i.e., traditional: Srinagar and Kishtwar, and non-traditional: Ramban. Furthermore, these fungal species were screened against saffron plant for causing symptoms of corm and root rot so as to evaluate the pathogenic and non-pathogenic fungi. Additionally the status of F. oxysporum as the most severe pathogen in saffron was also checked.
A total of 45 fungi were isolated from the saffron field soil of all the three regions. Ascomycota was the dominant community observed in the present study. Egidi et al. (2019) have also reported Ascomycota as the dominant fungal community in soil worldwide. Kumar et al. (2022b) reported the positive correlation of Ascomycota with phosphorus (P). They had investigated the effect of soil physical–chemical properties on bacterial and fungal community in two different compartments of soil of two different crop types—Chinese cabbage and flower cabbage. The soil with a high concentration of P has abundant Ascomycota that plays an important role in the absorption and utilization of soil P by the plants. Zhang et al. (2019) suggested the negative correlation of total nitrogen with the abundance of Mucoromycota.
The present study wherein samples have been evaluated in the vegetative phase is consistent with the earlier report from saffron cormosphere during the vegetative phase (Bhagat et al., 2021) but in contrast to the flowering and dominant phase (Ambardar et al., 2016). This confirms our previous hypothesis wherein we have reported that the mycobiome of cormosphere is dynamic and changes at each growth stage (Ambardar et al., 2016). Interestingly, studies on endophytic fungi of the saffron plant also report Ascomycota as a dominant endophytic fungal group (Wani et al., 2016; Belfiori et al., 2021; Jan et al., 2022). Ambardar et al. (2016) found Ascomycota to be dominant phylum in the bulk soil of saffron at both growth stages, i.e., dominant and flowering.
Seven genera, representing 45 OTUs in the present study, include Fusarium, Geotrichum, Mucor, Aspergillus, Trichoderma, Galactomyces, and Colletotrichum. The present report is in tune with the study conducted by El Aymani et al. (2019) on soil, roots, and corms of the saffron plant in the Taliouine region, Morocco. They found Fusarium, Aspergillus, Trichoderma, Rhizopus, and Penicillium genera in abundance. Interestingly, Fusarium, Mucor, and Trichoderma were the common genera observed in the present work and by Belfiori and colleagues in 10 different Italian saffron cultivation sites (Belfiori et al., 2021). Trichoderma, naturally in association with the saffron plant, has been reported by other research groups as well (El Aymani et al., 2019; Belfiori et al., 2021). Other genera that have been isolated by Belfiori et al. (2021) from the rotten saffron plant parts (corms, stems, and leaves) were Rhizopus, Epicoccum, Aureobasidium, and Talaromyces. In contrast to our results, Shuwen et al. (2021) have reported distinct fungal genera from corm samples of South Tai Lake Agricultural Park (Huzhou city, China) having different corm growth parameters such as germination and blooming. Infected corms had high levels of Trichocomaceae and Talaromyces genus, but non-germinating corms had high levels of the Aspergillaceae genus. In corms that could germinate but not bloom and in corms that could germinate and produce two blooms per corm, Penicillium and Dothideomycetes were found. Cladosporium, Lambertella, Passalora, Eurotiomycetes, Eurotiales, Aspergillus, Cladosporiaceae, and Capnodiales were the other fungal communities reported at the genus level by Shuwen et al. (2021).
Since few taxa and mostly F. oxysporum are associated in causing corm rot disease in saffron (Palmero et al., 2014; Gupta et al., 2020; Mirghasempour et al., 2022a), the isolated fungi in the present study were tested for pathogenicity to screen for pathogenic and non-pathogenic taxa. In our experiments with the previously isolated F. oxysporum R1 (Gupta and Vakhlu, 2015), it was established that it needs injury to invade the plant, and without injury, it cannot cause infection in the laboratory experiments (Bhagat et al., 2022). On similar lines, the pathogenicity was checked by injuring the corm as explained in detail in the Materials and methods section. According to the current findings, all the fungal species were pathogenic to corm and root but all fungi could cause disease only if the corm or root tissue was injured and no symptoms were observed in tissue without injury (Figure 8). In the present study, injury was found to be a must in the case of all the fungi screened as in the control, where the corms were sown in the soil infested with fungal spores but not injured. Since, in the present study, near-natural conditions were created by inoculating soil with fungal spores and planting corms and letting them grown naturally, we hypothesize that, in natural conditions, injury/natural wounds are the primary reason for fungal infection. There are reported reasons responsible for the occurrence of natural injury in corm such as injury being caused by the digging, storage, and re-sowing of corms (Gupta et al., 2021). In addition, nematodes and rodents have also been reported to attack the corms and cause injury (Khan and Sharma, 2020). Some researchers have reported the infection without injury and by just dipping the corm or germinated roots in the spore suspension (Palmero et al., 2014; Gupta et al., 2020; Mirghasempour et al., 2022b) but the present study and others have reported the infection after injuring the corm artificially (Gupta and Vakhlu, 2015; Wani et al., 2016; Wani et al., 2018; Zhang et al., 2020; Bhagat et al., 2022; Luo et al., 2022). There are many reports on other plants wherein the screening for pathogenicity is done by injury such as cork oak (Luque et al., 2000), kiwi fruit (Di Marco et al., 2004), grape vines (Halleen et al., 2007), Dendrobium nobile, and Dendrobium officinale (Sarsaiya et al., 2020), potato (Azil et al., 2021).
The establishment of Koch’s postulates suggested that F. oxysporum, F. solani, F. fujikuroi, F. neocosmosporiellum, and G. moniliformis (Fusarium moniliformae) were pathogenic to saffron but F. oxysporum was not the most severe. However, there was noticeable difference in the disease severity caused by various pathogens. F. oxysporum S12, F. oxysporum S30, and F. oxysporum K1 caused maximum disease severity of 75% at 30 dpi in saffron corms. The 75% disease severity was observed in other isolated strains such as F. solani K3, G. moniliformis K4, G. candidum K8, M. circinelloides K11, and A. flavus K16. F. oxysporum formae speciales such as iridiacearum, gladioli, and saffrani have already been recognized as the worst pathogens for saffron (Palmero et al., 2014; Najari et al., 2018; Belfiori et al., 2021; Gupta et al., 2021; Mirghasempour et al., 2022a). They cause corm rot disease in saffron and, therefore, resulted in severe losses in the saffron yield globally (Mirghasempour et al., 2022a). Interestingly, Wani et al. (2016) reported that endophytic F. oxysporum CSE15 exhibits latent pathogenic behavior in saffron and has strong antimycotic and plant growth-promoting properties. Moreover, the saprotroph, pathotroph, and symbiotroph trophic modes have all been well documented in the case of Fusarium genera (Belfiori et al., 2021). El Aymani et al. (2019) have presented F. solani as saffron-associated species. Additionally, F. solani can cause diseases in several crops including pea, Zea mays (Okello et al., 2019; Gibert et al., 2022). Quite a number of reports suggest that F. oxysporum is the most common cause of corm rot and devastating fungi (Yamamoto et al., 1954; Di Primo and Cappelli, 2000; Gupta et al., 2011; Gupta and Vakhlu, 2015; Gupta et al., 2020; Ahmad et al., 2022). The literature survey revealed F. oxysporum as a common corm rot pathogen, and to our understanding, the basis of this is the isolation of the F. oxysporum from the rotten corm, but there are reports that confirmed other fungal pathogens such as Fusarium nirenbergiae, F. annulatum, F. commune, F. culmorum, F. roseum, Penicillium cyclopium, Aspergillus niger, Rhizopus oryzae, and Phoma spp. as saffron corm rot agents (Gupta et al., 2021; Mirghasempour et al., 2022b). In addition, the reports claiming F. oxysporum to be the dominant corm rot causing pathogen are about two decades (and more) old, and no recent survey of saffron fields to find dominant fungal pathogen has been carried out (Table 6).
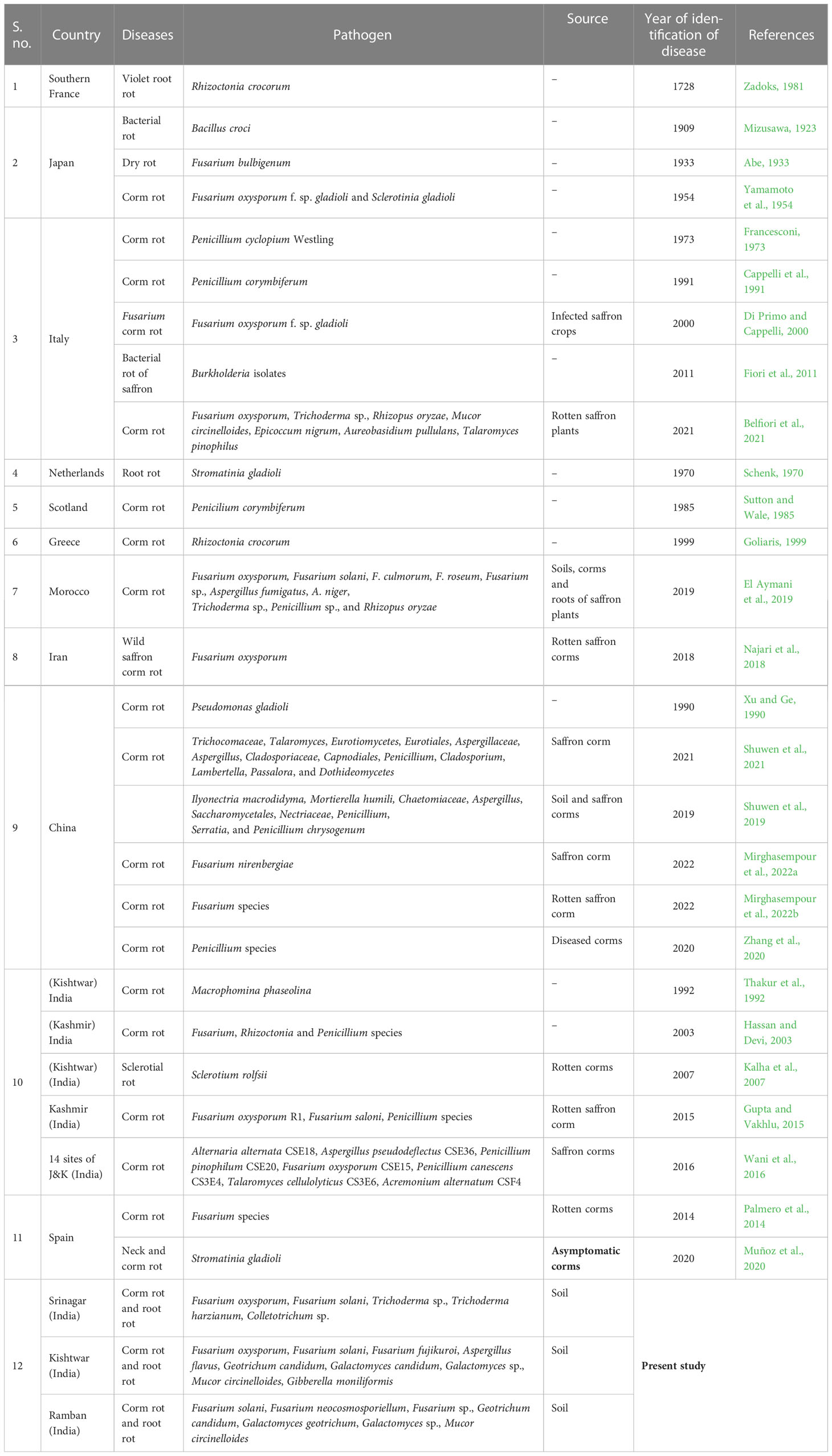
Table 6 Worldwide reports of saffron diseases caused by various pathogens, with the year of identification and source of isolation.
To the best of our knowledge, F. fujikuroi, F. neocosmosporiellum, and G. moniliformis are being reported as pathogens from the saffron fields of Jammu and Kashmir, for the first time. The presence of these species has been studied in different plants. F. fujikuroi has previously been accounted as endophytic fungi, isolated from Debregeasia salicifolia (Nisa et al., 2020). Moreover, F. fujikuroi has been reported as herbicidal (Daniel et al., 2018). Nonetheless, F. neocosmosporiellum has earlier been reported as a potent pathogen of mango, causing mango malformation disease (Molina-Cárdenas et al., 2021). Additionally, F. neocosmosporiellum has also been isolated from the infected peanut plants (Xu et al., 2021). On the other hand, G. moniliformis is the well-known causal agent of ear rot and stalk rot diseases of maize (Gai et al., 2018).
Colletotrichum also has been documented as pathogens by many reports (Zakaria, 2021) such as sugarcane (Franco et al., 2022), capsicum (Giacomin et al., 2021), and banana (Sudarma et al., 2021). Moreover, Colletotrichum species have been reported as endophytes, having various bioactive metabolites (Santra and Banerjee, 2022). The Aspergillus species isolated in this study on close examination resembled A. flavus; however, El Aymani et al. (2019) have found Aspergillus fumigates and A. niger species on close association with the saffron plant. In addition to all the above-mentioned fungal species, one more species, G. candidum, whose sexual stage is named G. geotrichum, was isolated in the present study. The asexual stage G. candidum belongs to the family Moniliaceae, whereas the sexual stage G. geotrichum belongs to the Candida family. There are more than 10 synonyms reported for G. candidum (Ma et al., 2018). In the present study, both the sexual and the asexual stage were found and both were pathogenic to the saffron plant. As such, there are no data available regarding G. candidum in context to the saffron plant. However, previous studies have already revealed G. candidum as a strongly virulent pathogen to carrots (Horita and Hatta, 2016), citrus plants (Wang et al., 2021), strawberry (Ma et al., 2018), and tomatoes (Thomidis et al., 2021).
In accordance with our studies, M. circinelloides and Trichoderma sp. have also been isolated from the rotten saffron plant parts by Belfiori et al. (2021). Although M. circinelloides is a well-known phytopathogen (Medina-Córdova et al., 2016), members of the Trichoderma genus are popular for their protection activities and represent global distribution as plant endophytes. Moreover, they are well-known antagonists for pathogenic fungal species and also act as plant growth promoters (Cuervo-Parra et al., 2022). Trichoderma have been used to manage many diseases and hence are commercially available biological control agents. They have been applied to a variety of plants for disease management, including tomato (Li et al., 2018), soybeans (Cruz et al., 2017), beans (Sánchez-García et al., 2017), cocoa (Romero-Cortes et al., 2019), and onion (Bunbury-Blanchette and Walker, 2019). In the saffron plant, Gupta et al. (2020) have evaluated the efficacy of Trichoderma asperellum on the corm rot causative agent, F. oxysporum, and found reduction in the disease incidence. The isolated T. harzianum has been observed as a pathogen in our study; however, it was an evident biocontrol agent reported by other studies (da Silva et al., 2022; Moreira et al., 2022). Hence, further research is required to determine its potential role as a pathogen antagonist.
Out of the fungi isolated, we found all of them to be pathogenic with varying degrees of disease severity. The six F. oxysporum strains isolated in the present study are not exclusively severe saffron corm rot pathogens; there were other fungi isolated that were equally severe such as F. solani, G. moniliformis, G. candidum, M. circinelloides, and A. flavus. However, out of six isolated F. oxysporum strains, none was found to be the most severe saffron root rot pathogen. In addition, we found that it is the injury that makes corm susceptible to the opportunistic fungi in the soil, and all the fungi that had been isolated were pathogenic only if a corm/root was injured, not otherwise. Under natural conditions, however, other parameters may play a role in infection such as humidity and temperature (Victorino et al., 2021), but to our understanding, injury is most fatal if it is caused by natural conditions such as farming practices, rodents, and nematodes.
5 Conclusion
Pathogens are evolving fast, invading new plant hosts, and climate change is adding to that. Based on decades-old reports, F. oxysporum f. sp. gladiolus has been reported as the most severe pathogen of saffron, causing corm rot without any molecular phylogeny. There are various strains of F. oxysporum present in the soil as it is the dominant taxa of the most dominant soil phylum, i.e., Ascomycota. The severity in the strains may vary, and this needs to be validated by data. The data for the isolation and characterization of the pathogenic F. oxysporum causing corm rot are isolated, random, and scarce. In our opinion, to establish the pathogen as most severe, causing the devastating rot to the world’s costliest spice, comparative data with robust experimentation are required. Moreover, in the present study, it was also observed that all the fungal isolates cause rot symptoms, when the corm is injured; thus, it is injury that can be a major cause of the corm rot and yield loss rather than the pathogen. Although this hypothesis needs to be further validated in field experiments, we strongly recommend sowing injury-free corms so as to prevent future potential outbreaks instead of the unchecked use of chemical fungicides. This study revealed that Srinagar, which is a traditional area for saffron cultivation in India, has less diverse fungal species as compared to the other two studied locations and that T. harzianum, which is used as a biocontrol, is also a potential pathogen. We recommend screening of symptoms and injury-free quality corms before sowing and regulated use of chemical as well as biological agents in saffron fields for sustainable saffron production.
Data availability statement
The datasets presented in this study can be found in online repositories. The names of the repository/repositories and accession number(s) can be found in the article/Supplementary Material.
Author contributions
JV conceived the research idea. RM and JV designed the experiments. RM with the help of TA performed the experiments. JV supervised the experiments. RM performed data analysis. JV and RM together drafted the manuscript. NB provided inputs in manuscript. All authors contributed to the article and approved the submitted version.
Funding
This study was supported by a grant from the Core Research Grant, Science and Engineering Research Board, Department of Science and Technology (CRG/2021/002257).
Conflict of interest
The authors declare that the research was conducted in the absence of any commercial or financial relationships that could be construed as a potential conflict of interest.
Publisher’s note
All claims expressed in this article are solely those of the authors and do not necessarily represent those of their affiliated organizations, or those of the publisher, the editors and the reviewers. Any product that may be evaluated in this article, or claim that may be made by its manufacturer, is not guaranteed or endorsed by the publisher.
Supplementary material
The Supplementary Material for this article can be found online at: https://www.frontiersin.org/articles/10.3389/fpls.2023.1074185/full#supplementary-material
References
Abe, T. (1933). Studies on a new dry rot disease of the bulb of Crocus sativus l. caused by Fusarium bulbigenum cke. et. mass var. blasticola (Rostr.) wr. Trans. Tottori Soc Agric. Sci. 4, 3.
Ahmad, T., Bashir, A., Farooq, S., Riyaz-Ul-Hassan, S. (2022). Burkholderia gladioli E39CS3, an endophyte of Crocus sativus linn., induces host resistance against corm-rot caused by Fusarium oxysporum. J. Appl. Microbiol. 132, 495–508. doi: 10.1111/jam.15190
Ambardar, S., Singh, H. R., Gowda, M., Vakhlu, J. (2016). Comparative metagenomics reveal phylum level temporal and spatial changes in mycobiome of belowground parts of Crocus sativus. PloS One 11, e0163300. doi: 10.1371/journal.pone.0163300
Avan, M., Palacıoğlu, G., Aksoy, C., Kaya, R., Bayraktar, H., Katırcıoğlu, Y. Z., et al. (2021). Characterization and pathogenicity of Rhizoctonia species causing root rot and damping-off on sugar beet in Turkey. Curr. Microbiol. 78, 1939–1948. doi: 10.1007/s00284-021-02470-4
Azil, N., Stefańczyk, E., Sobkowiak, S., Chihat, S., Boureghda, H., Śliwka, J. (2021). Identification and pathogenicity of fusarium spp. associated with tuber dry rot and wilt of potato in Algeria. Eur. J. Plant Pathol. 159, 495–509. doi: 10.1007/s10658-020-02177-5
Belfiori, B., Rubini, A., Riccioni, C. (2021). Diversity of endophytic and pathogenic fungi of saffron (Crocus sativus) plants from cultivation sites in Italy. Diversity 13, 535. doi: 10.3390/d13110535
Bhagat, N., Magotra, S., Gupta, R., Sharma, S., Verma, S., Verma, P. K., et al. (2022). Invasion and colonization of pathogenic Fusarium oxysporum R1 in Crocus sativus l. during corm rot disease progression. J. Fungi 8, 1246. doi: 10.3390/jof8121246
Bhagat, N., Sharma, S., Ambardar, S., Raj, S., Trakroo, D., Horacek, M., et al. (2021). Microbiome fingerprint as biomarker for geographical origin and heredity in Crocus sativus: A feasibility study. Front. Sustain. Food Syst. 5. doi: 10.3389/fsufs.2021.688393
Bhunjun, C. S., Phillips, A. J., Jayawardena, R. S., Promputtha, I., Hyde, K. D. (2021). Importance of molecular data to identify fungal plant pathogens and guidelines for pathogenicity testing based on koch’s postulates. Pathogens 10, 1096. doi: 10.3390/pathogens10091096
Bunbury-Blanchette, A. L., Walker, A. K. (2019). Trichoderma species show biocontrol potential in dual culture and greenhouse bioassays against Fusarium basal rot of onion. Biol. Control 130, 127–135. doi: 10.1016/j.biocontrol.2018.11.007
Cappelli, C., Buonaurio, R., Polverari, A. (1991). Occurrence of Penicillium corymbiferum on saffron in Italy. Plant Pathol. 40, 148–149. doi: 10.1111/j.1365-3059.1991.tb02304.x
Cardone, L., Castronuovo, D., Perniola, M., Cicco, N., Candido, V. (2020). Saffron (Crocus sativus l.), the king of spices: An overview. Sci. Hortic. 272, 109560. doi: 10.1016/j.scienta.2020.109560
Chamkhi, I., Sbabou, L., Aurag, J. (2018). Endophytic fungi isolated from Crocus sativus l. (saffron) as a source of bioactive secondary metabolites. Pharmacogn. Mag. 10. doi: 10.5530/pj.2018.6.195
Cruz, T. A., Rivero, G. D., Martínez, C. B., Echevarría, H. A., Tania, R. A. (2017). Evaluation of the antifungal activity of trichoderma asperellum samuels against fungal pathogens that affect soybean cultivation (Glycine max l.). Cult. Trop. 38, 15–12.
Cuervo-Parra, J. A., Pérez España, V. H., Zavala-González, E. A., Peralta-Gil, M., Aparicio Burgos, J. E., Romero-Cortes, T. (2022). Trichoderma asperellum strains as potential biological control agents against Fusarium verticillioides and Ustilago maydis in maize. Biocontrol Sci. Technol. 32, 624–647. doi: 10.1080/09583157.2022.2042196
Daniel, J. J., Jr., Zabot, G. L., Tres, M. V., Harakava, R., Kuhn, R. C., Mazutti, M. A. (2018). Fusarium fujikuroi: A novel source of metabolites with herbicidal activity. Biocatal. Agric. Biotechnol. 14, 314–320. doi: 10.1016/j.bcab.2018.04.001
da Silva, F. L., Aquino, E. N., da Cunha, D. C., Hamann, P. R. V., Magalhães, T. B., Steindorff, A. S., et al. (2022). Analysis of Trichoderma harzianum TR 274 secretome to assign candidate proteins involved in symbiotic interactions with Phaseolus vulgaris. Biocatal. Agric. Biotechnol., 102380. doi: 10.1016/j.bcab.2022.102380
Di Marco, S., Calzarano, F., Osti, F., Mazzullo, A. (2004). Pathogenicity of fungi associated with a decay of kiwifruit. Australas. Plant Pathol. 33, 337–342. doi: 10.1071/AP04024
Di Primo, P., Cappelli, C. (2000). Preliminary characterization of Fusarium oxysporum f. sp. gladioli causing fusarium corm rot of saffron in Italy. Plant Dis. 84, 806–806. doi: 10.1094/PDIS.2000.84.7.806C
Egidi, E., Delgado-Baquerizo, M., Plett, J. M., Wang, J., Eldridge, D. J., Bardgett, R. D., et al. (2019). A few ascomycota taxa dominate soil fungal communities worldwide. Nat. Commun. 10, 1–9. doi: 10.1038/s41467-019-10373-z
El Aymani, I., Qostal, S., Mouden, N., Selmaoui, K., Touhami, A. O., Benkirane, R., et al. (2019). Fungi associated with saffron (Crocus sativus) in Morocco. Plant Cell Biotechnol. Mol. Biol., 1180–1188.
Fiori, M., Ligios, V., Schiaffino, A. (2011). Identification and characterization of Burkholderia isolates obtained from bacterial rot of saffron (Crocus sativus l.) grown in Italy. Phytopathol. Mediterr. 50, 450–461.
Francesconi, A. (1973). The rotting of bulbs of Crocus sativus l. by Penicillium cyclopium westling.
Franco, F. P., Túler, A. C., Gallan, D. Z., Gonçalves, F. G., Favaris, A. P., Peñaflor, M. F. G., et al. (2022). Colletotrichum falcatum modulates the olfactory behavior of the sugarcane borer, favoring pathogen infection. FEMS Microbiol. Ecol. 98 (4), fiac035. doi: 10.1093/femsec/fiac035
Gai, X., Dong, H., Wang, S., Liu, B., Zhang, Z., Li, X., et al. (2018). Infection cycle of maize stalk rot and ear rot caused by Fusarium verticillioides. PloS One 13, e0201588. doi: 10.1371/journal.pone.0201588
Garcia-Jimenez, J., Alfaro Garcia, A. (1987). “Fusarium oxysporum schlecht. as causal agent of a seedborne disease of saffron (Crocus sativus l.),” in Proceedings of the 7th Congress of the Mediterranean Phytopathological Union. 156.
Giacomin, R. M., de Fátima Ruas, C., Baba, V. Y., De Godoy, S. M., Sudré, C. P., dos Santos Bento, C., et al. (2021). Phenotypic, molecular and pathogenic characterization of Colletotrichum scovillei infecting Capsicum species in Rio de Janeiro, Brazil. PeerJ 9, e10782. doi: 10.7717/peerj.10782
Gibert, S., Edel-Hermann, V., Gautheron, E., Gautheron, N., Sol, J. M., Capelle, G., et al. (2022). First report of Fusarium avenaceum, Fusarium oxysporum, Fusarium redolens, and Fusarium solani causing root rot in pea in France. Plant Dis. 106, 1297. doi: 10.1094/PDIS-04-21-0833-PDN
Gupta, V., Kalha, C. S., Razdan, V. K. (2011). Dolly. etiology and management of corm rot of saffron in kishtwar district of jammu and Kashmir. India. J. Mycol. Plant Pathol. 41, 361–366.
Gupta, V., Kumar, K., Fatima, K., Razdan, V. K., Sharma, B. C., Mahajan, V., et al. (2020). Role of biocontrol agents in management of corm rot of saffron caused by Fusarium oxysporum. Agronomy 10, 1398. doi: 10.3390/agronomy10091398
Gupta, V., Sharma, A., Rai, P. K., Gupta, S. K., Singh, B., Sharma, S. K., et al. (2021). Corm rot of saffron: Epidemiology and management. Agronomy 11, 339. doi: 10.3390/agronomy11020339
Gupta, R., Vakhlu, J. (2015). Native bacillus amyloliquefaciens W2 as a potential biocontrol for Fusarium oxysporum R1 causing corm rot of Crocus sativus. Eur. J. Plant Pathol. 143, 123–131. doi: 10.1007/s10658-015-0670-3
Halleen, F., Mostert, L., Crous, P. W. (2007). Pathogenicity testing of lesser-known vascular fungi of grapevines. Australas. Plant Pathol. 36, 277–285. doi: 10.1071/AP07019
Hammer, Ø., Harper, D. A., Ryan, P. D. (2001). PAST: Paleontological statistics software package for education and data analysis. Palaeontol. Electron. 4, 9.
Hassan, M. G., Devi, L. S. (2003). Corm rot diseases of saffron in Kashmir valley. Indian Phytopathol. 56 (1).
Horita, H., Hatta, Y. (2016). Sour rot of carrot caused by Geotrichum candidum in Japan. J. Gen. Plant Pathol. 82, 65–68. doi: 10.1007/s10327-015-0638-3
Husaini, A. M., Jiménez, A. J. L. (2022). Understanding saffron biology using omics-and bioinformatics tools: stepping towards a better Crocus phenome. Mol. Biol. Rep., 1–16. doi: 10.1007/s11033-021-07053-x
Jan, B., Reshi, Z. A., Mohiddin, F. A. (2022). Site and organ-specific culture-dependent endophytic diversity of Crocus sativus L.(Saffron) in Kashmir himalaya, India. Microb. Ecol. 83, 989–1006. doi: 10.1007/s00248-021-01817-5
Kalha, C. S., Gupta, V., Gupta, D., Priya, S. (2007). First report of sclerotial rot of saffron caused by Sclerotium rolfsii in India. Plant Dis. 91, 1203–1203. doi: 10.1094/PDIS-91-9-1203B
Khan, M. R., Sharma, R. K. (2020). Fusarium-nematode wilt disease complexes, etiology and mechanism of development. Indian Phytopathol. 73, 615–628. doi: 10.1007/s42360-020-00240-z
Kothari, D., Thakur, R., Kumar, R. (2021). Saffron (Crocus sativus l.): gold of the spices–a comprehensive review. Hortic. Environ. Biotechnol., 62, 661–6777. doi: 10.1007/s13580-021-00349-8
Kumar, A., Devi, M., Kumar, R., Kumar, S. (2022a). Introduction of high-value Crocus sativus (saffron) cultivation in non-traditional regions of India through ecological modelling. Sci. Rep. 12, 1–11. doi: 10.1038/s41598-022-15907-y
Kumar, U., Saqib, H. S. A., Islam, W., Prashant, P., Patel, N., Chen, W., et al. (2022b). Landscape composition and soil physical–chemical properties drive the assemblages of bacteria and fungi in conventional vegetable fields. Microorganisms 10, 1202. doi: 10.3390/microorganisms10061202
Li, Y. T., Hwang, S. G., Huang, Y. M., Huang, C. H. (2018). Effects of Trichoderma asperellum on nutrient uptake and Fusarium wilt of tomato. Crop Prot. 110, 275–282. doi: 10.1016/j.cropro.2017.03.021
Luo, J., Zhang, A., Tan, K., Yang, S., Ma, X., Bai, X., et al. (2022). Study on the interaction mechanism between Crocus sativus and Fusarium oxysporum based on dual RNA-seq. doi: 10.21203/rs.3.rs-1677222/v1
Luque, J., Parladé, J., Pera, J. (2000). Pathogenicity of fungi isolated from Quercus suber in Catalonia (NE Spain). For. Pathol. 30, 247–263. doi: 10.1046/j.1439-0329.2000.00208.x
Ma, W., ZhanG, Y., WanG, C., Liu, S., Liao, X. (2018). A new disease of strawberry, fruit rot, caused by Geotrichum candidum in China. Plant Prot. Sci. 54, 92–100. doi: 10.17221/76/2017-PPS
Medina-Córdova, N., López-Aguilar, R., Ascencio, F., Castellanos, T., Campa-Córdova, A. I., Angulo, C. (2016). Biocontrol activity of the marine yeast Debaryomyces hansenii against phytopathogenic fungi and its ability to inhibit mycotoxins production in maize grain (Zea mays l.). Biol. Control 97, 70–79. doi: 10.1016/j.biocontrol.2016.03.006
Mirghasempour, S. A., Studholme, D. J., Chen, W., Cui, D., Mao, B. (2022a). Identification and characterization of Fusarium nirenbergiae associated with saffron corm rot disease. Plant Dis. 106, 486–495. doi: 10.1094/PDIS-04-21-0871-RE
Mirghasempour, S. A., Studholme, D. J., Chen, W., Zhu, W., Mao, B. (2022b). Molecular and pathogenic characterization of Fusarium species associated with corm rot disease in saffron from China. J. Fungi 8, 515. doi: 10.3390/jof8050515
Mizusawa, Y. (1923). A bacterial rot disease of saffrons. JJP. 1, 1–12. doi: 10.3186/jjphytopath.1.5_1
Molina-Cárdenas, L., López-Urquídez, G. A., Amarillas-Bueno, L. A., Vega-Gutierrez, T. A., Tirado-Ramírez, M. A., Velázquez-Alcaraz, T. D. J., et al. (2021). Mango malformation disease caused by Fusarium neocosmosporiellum in Mexico. Can. J. Plant Pathol. 43, 714–721. doi: 10.1080/07060661.2021.1880483
Moreira, V. D. A., Oliveira, C. E. D. S., Jalal, A., Gato, I. M. B., Oliveira, T. J. S. S., Boleta, G. H. M., et al. (2022). Inoculation with Trichoderma harzianum and Azospirillum brasilense increases nutrition and yield of hydroponic lettuce. Arch. Microbiol. 204, 1–12. doi: 10.1007/s00203-022-03047-w
Muñoz, R. M., Lerma, M. L., Castillo, P., Armengol, J., Somoza, E., Woodhall, J. W. (2020). First report of Stromatinia gladioli causing neck and corm rot of Crocus sativus in Spain. Plant Dis. 104, 282. doi: 10.1094/PDIS-02-19-0294-PDN
Najari, G., Nourollahi, K., Piri, M. (2018). The first report of Fusarium oxysporum causal agent of wild saffron corm rot disease in Iran. Saffron Agron. technol. 6, 119–123. doi: 10.22048/jsat.2017.59518.1185
Nisa, S., Khan, N., Shah, W., Sabir, M., Khan, W., Bibi, Y., et al. (2020). Identification and bioactivities of two endophytic fungi Fusarium fujikuroi and Aspergillus tubingensis from foliar parts of Debregeasia salicifolia. Arab J. Sci. Eng. 45, 4477–4487. doi: 10.1007/s13369-020-04454-1
Okello, P. N., Petrović, K., Kontz, B., Mathew, F. M. (2019). Eight species of Fusarium cause root rot of corn (Zea mays) in south Dakota. Plant Health Prog. 20, 38–43. doi: 10.1094/PHP-11-18-0075-RS
Palmero, D., Rubio-Moraga, A., Galvez-Patón, L., Nogueras, J., Abato, C., Gómez-Gómez, L., et al. (2014). Pathogenicity and genetic diversity of Fusarium oxysporum isolates from corms of Crocus sativus. Ind. Crops Prod. 61, 186–192. doi: 10.1016/j.indcrop.2014.06.051
Rather, A. M., Rashid, S., Sultan, R., Nawchoo, I. A., Wani, I. A., Kamili, A. N. (2022). Hurdles and clarifications for the cultivation of saffron in jammu and Kashmir. J. Agric. Food Res. 10, 100344. doi: 10.1016/j.jafr.2022.100344
Ricotta, C. (2002). Bridging the gap between ecological diversity indices and measures of biodiversity with shannon’s entropy: comment to izsák and papp. Ecol. Modell. 152, 1–3. doi: 10.1016/S0304-3800(01)00468-9
Romero-Cortes, T., López-Pérez, P. A., Pérez, E. V. H., Medina-Toledo, A. K., Aparicio-Burgos, J. E., Cuervo-Parra, J. A. (2019). Confrontation of Trichoderma asperellum VSL80 against Aspergillus niger via the effect of enzymatic production. Chil. J. Agric. Anim. Sci. 35, 68–80. doi: 10.4067/S0719-38902019005000202
Saghai-Maroof, M. A., Soliman, K. M., Jorgensen, R. A., Allard, R. W. L. (1984). Ribosomal DNA spacer-length polymorphisms in barley: Mendelian inheritance, chromosomal location, and population dynamics. Proc. Natl. Acad. Sci. 81, 8014–8018. doi: 10.1073/pnas.81.24.8014
Sánchez-García, B. M., Espinosa-Huerta, E., Villordo-Pineda, E., Rodríguez-Guerra, R., Mora-Avilés, M. A. (2017). Trichoderma spp. native strains molecular identification and in vitro antagonistic evaluation of root phytopathogenic fungus of the common bean (Phaseolus vulgaris l.) cv. montcalm. Agrociencia 51, 63–79.
Santra, H. K., Banerjee, D. (2022). Bioactivity study and metabolic profiling of Colletotrichum alatae LCS1, an endophyte of club moss Lycopodium clavatum l. PloS One 17, e0267302. doi: 10.1371/journal.pone.0267302
Sarsaiya, S., Jain, A., Jia, Q., Fan, X., Shu, F., Chen, Z., et al. (2020). Molecular identification of endophytic fungi and their pathogenicity evaluation against Dendrobium nobile and Dendrobium officinale. Int. J. Mol. Sci. 21, 316. doi: 10.3390/ijms21010316
Schenk, P. K. (1970). Root rot in Crocus. Neth. J. Plant Pathol. 76, 159–164. doi: 10.1007/BF01974324
Shuwen, H., Qing, Z., Jin, L., Miao, D. (2021). Fungal diversity on the surface of saffron corms with different growth characteristics. Plant Biosyst. 155, 302–309. doi: 10.1080/11263504.2020.1739163
Shuwen, H., Xi, Y., Liqin, L., Yumei, Y., Guifen, Z., Chong, Y., et al. (2019). Limiting factors of saffron corm production from the perspective of microorganisms. Sci. Hortic. 247, 165–174. doi: 10.1016/j.scienta.2018.11.002
Sudarma, I. M., Suniti, N. W., Darmiati, N. N. (2021). Molecular identification of anthrachnose pathogen (Colletotrichum musae) in banana fruits and the use of exophitic and endophyticfungi to control the pathogen. GPH-IJAR. 4, 01–11.
Sutton, M. W., Wale, S. J. (1985). The control of Penicillium corymbiferum on Crocus and its effect on corm production. Plant Pathol. 34, 566–570. doi: 10.1111/j.1365-3059.1985.tb01408.x
Thakur, R. N., Singh, C., Kaul, B. L. (1992). First report of corm rot in Crocus sativus. Indian Phytopathol. 45.
Thomidis, T., Prodromou, I., Farmakis, A., Zambounis, A. (2021). Effect of temperature on the growth of Geotrichum candidum and chemical control of sour rot on tomatoes. Trop. Plant Pathol. 46, 545–552. doi: 10.1007/s40858-021-00453-1
Victorino, Í. M. M., Voyron, S., Caser, M., Orgiazzi, A., Demasi, S., Berruti, A., et al. (2021). Metabarcoding of soil fungal communities associated with alpine field-grown saffron (Crocus sativus l.) inoculated with AM fungi. J. Fungi 7, 45. doi: 10.3390/jof7010045
Waksman, S. A. (1922). A method for counting the number of fungi in the soil. J. Bacteriol. 7, 339–341. doi: 10.1128/jb.7.3.339-341.1922
Wang, W., Feng, G., Li, X., Ruan, C., Ming, J., Zeng, K. (2021). Inhibition of three citrus pathogenic fungi by peptide PAF56 involves cell membrane damage. Foods 10, 2031. doi: 10.3390/foods10092031
Wani, Z. A., Ahmad, T., Nalli, Y., Ali, A., Singh, A. P., Vishwakarma, R. A., et al. (2018). Porostereum sp., associated with saffron (Crocus sativus l.), is a latent pathogen capable of producing phytotoxic chlorinated aromatic compounds. Curr. Microbiol. 75, 880–887. doi: 10.1007/s00284-018-1461-9
Wani, Z. A., Mirza, D. N., Arora, P., Riyaz-Ul-Hassan, S. (2016). Molecular phylogeny, diversity, community structure, and plant growth promoting properties of fungal endophytes associated with the corms of saffron plant: An insight into the microbiome of Crocus sativus Linn. Fungal Biol. 120, 1509–1524. doi: 10.1016/j.funbio.2016.07.011
Xu, C. X., Ge, Q. X. (1990). A preliminary study on corm rot of Crocus sativus l. Acta Agric. Univ. Zhejiangensis. 16, 241–246.
Xu, M., Zhang, X., Guo, Z., Yu, J., Wu, J., Li, X., et al. (2021). First report of peanut foot rot caused by Fusarium neocosmosporiellum in Shandong province, China. J. Plant Pathol. 103, 1059–1060. doi: 10.1007/s42161-021-00867-5
Yamamoto, W., Omatsu, T., Takami, K. (1954). Studies on the corm rots of Crocus sativus L1 on saprophytic propagation of Sclerotinia gladioli and Fusarium oxysporum f. sp. gladioli on various plants and soils. Sci. Rep. Hyogo Univ. Agric. 1, 64–70.
Zadoks, J. C. (1981). Mr. DuHamel’s 1728 treatise on the violet root rot of saffron crocus: physical explanation of a disease that perishes several plants in the gastinois, and saffron in particular (No. 81-7). Landbouwhogeschool.
Zakaria, L. (2021). Diversity of colletotrichum species associated with anthracnose disease in tropical fruit crops–a review. Agriculture 11, 297. doi: 10.3390/agriculture11040297
Zhang, T., Huang, C., Deng, C., Zhang, Y., Feng, Y., Hu, J., et al. (2020). First report of corm rot on saffron caused by Penicillium solitum in China. Plant Dis. 104, 579–579. doi: 10.1094/PDIS-09-19-1927-PDN
Keywords: saffron fields, Fusarium oxysporum, fungal pathogens, injury, saffron corms
Citation: Mansotra R, Ali T, Bhagat N and Vakhlu J (2023) Injury and not the pathogen is the primary cause of corm rot in Crocus sativus (saffron). Front. Plant Sci. 14:1074185. doi: 10.3389/fpls.2023.1074185
Received: 19 October 2022; Accepted: 03 January 2023;
Published: 24 January 2023.
Edited by:
Brigitte Mauch-Mani, Université de Neuchâtel, SwitzerlandReviewed by:
Elsherbiny A. Elsherbiny, Mansoura University, EgyptVishal Gupta, Sher-e-Kashmir University of Agricultural Sciences and Technology of Jammu, India
Copyright © 2023 Mansotra, Ali, Bhagat and Vakhlu. This is an open-access article distributed under the terms of the Creative Commons Attribution License (CC BY). The use, distribution or reproduction in other forums is permitted, provided the original author(s) and the copyright owner(s) are credited and that the original publication in this journal is cited, in accordance with accepted academic practice. No use, distribution or reproduction is permitted which does not comply with these terms.
*Correspondence: Jyoti Vakhlu, anlvdGltZXRhZ2Vub21pY0BnbWFpbC5jb20=