- 1Tsinghua-Peking Joint Center for Life Sciences, and MOE Key Laboratory of Bioinformatics, School of Life Sciences, Tsinghua University, Beijing, China
- 2Fujian Provincial Key Laboratory of Agroecological Processing and Safety Monitoring, School of Life Sciences, Fujian Agriculture and Forestry University, Fuzhou, China
- 3Key Laboratory of Crop Physiology and Molecular Ecology, Fujian Agricultural and Forestry University, Fuzhou, China
- 4Institute of Environmental Microbiology, College of Resources and Environment, Fujian Agricultural and Forestry University, Fuzhou, China
Background: Ratoon rice cropping has been shown to provide new insights into overcoming the current challenges of rice production in southern China. However, the potential mechanisms impacting yield and grain quality under rice ratooning remain unclear.
Methods: In this study, changes in yield performance and distinct improvements in grain chalkiness in ratoon rice were thoroughly investigated, using physiological, molecular and transcriptomic analysis.
Results: Rice ratooning induced an extensive carbon reserve remobilization in combination with an impact on grain filling, starch biosynthesis, and ultimately, an optimization in starch composition and structure in the endosperm. Furthermore, these variations were shown to be associated with a protein-coding gene: GF14f (encoding GF14f isoform of 14-3-3 proteins) and such gene negatively impacts oxidative and environmental resistance in ratoon rice.
Conclusion: Our findings suggested that this genetic regulation by GF14f gene was the main cause leading to changes in rice yield and grain chalkiness improvement of ratoon rice, irrespective of seasonal or environmental effects. A further significance was to see how yield performance and grain quality of ratoon rice were able to be achieved at higher levels via suppression of GF14f.
Introduction
China is the largest global producer and consumer of rice (FAO, 2020). This is especially true regarding Southern China, which makes the greatest contribution to both China's rice industry and global rice production (Lin et al., 2022). However, Southern China has now been forced to significantly change its practices because light and temperature resources in this region are enough for one seasonal crop but insufficient for two seasons (Lin et al., 2015). To counteract this challenge, ratoon rice cropping was introduced for greater rice production and has become increasingly popular in Southern China (Xu et al., 2021). Rice ratooning refers to a regenerative phenomenon of rice where new tissues can develop from dormant buds of stem nodes on residual stubbles after harvesting the main crop (Plucknett et al., 1970), and is the hallmark of more green and economic rice production (Shen et al., 2021; Yu et al., 2021; Xu et al., 2022). Modern forms of ratoon rice cropping originate from Texas and southern Louisiana, USA, having had a long history there beginning in approximately 1927 and then expanding to Asian countries in 1970 (Wang et al., 2021). In the past decade in China, ratoon rice cropping has been widely disseminated due to the Chinese government actively promoting the practice and introducing several policies, such as the Project of High-Yield Cultivation Techniques for Ratoon Rice (2009-2010), the Project of Comprehensive Cultivation Techniques for Ratoon Rice (2015-2016), and the Project of High-Yield and Highly Efficient Cultivation Techniques for the Mechanized Harvesting of Ratoon Rice (2017-2018). Through the use of this system, an average increase in yield was achieved, with the yield advantages of ratoon rice cropping jumping from 26.1% to 71.43%, as compared to the traditional cropping system (Firouzi et al., 2018; Yuan et al., 2019). Not only that, ratoon rice was able to meet the fast-growing market demand for grain quality in the global rice industry (Fitzgerald et al., 2009). It has been documented that ratoon rice cropping significantly contributes to a distinct improvement in rice quality. For example, the physicochemical properties and textural characteristics of cooked ratoon rice were highly improved when compared to the main crop (Deng et al., 2021). Zhou (2006) and Wu (2005) claimed a significant decrease in chalky grain percentage in ratoon rice as compared to the main crop. Recently, it has been documented that ratoon rice significantly reduced grain chalkiness as compared to late-season rice, indicating that such an improvement in the chalkiness of ratoon rice might be irrespective of the seasonal effect (Huang et al., 2020). However, the potential molecular mechanism remains unclear. Despite that, there is compelling evidence to claim the significance of ratoon rice cropping for higher yield and better quality rice.
Grain filling is the final stage of growth for cereals with a duration that continues into maturity. Grain filling will initiate source-to-sink transport for delivering the carbon and nitrogen substances into caryopsis (Cruz-Aguado et al., 1999) and, as a result, determine the yield and quality of cereals (Zhang et al., 2021; Wang et al., 2008; Shimoyanagi et al., 2021). However, both source-to-sink transport and grain-filling properties are distinctly different in the comparisons between ratoon rice and traditionally-cultivated rice. Rice ratooning can regenerate new spikes from auxiiary buds on the residual stubbles and break into the grain-filling stage instead of undergoing a long-day vegetative growth period (Lin et al., 2015). This means that there is an inherent pre-start and speed-up for senescence occurring in ratoon rice. It was previously pointed out that a whole senescence sequence of cereal is required to remobilize and transfer assimilates pre-stored in vegetative tissues to grains (Yang and Zhang, 2006). It has been widely accepted that the role of plant hormones, especially regarding abscisic acid (ABA), determines the intimate relationship between senescence and carbon reserve remobilization (Yang et al., 2002; Yang et al., 2006; Wu et al., 2008). For example, ABA promotes the transference of carbohydrates from stem to grain by regulating the relative gene expression in carbon reserve remobilization (Wang and Zhang, 2020; Wang et al., 2020). Meanwhile, the sucrose and abscisic acid interaction affected the activities of key enzymes in sucrose-to-starch conversion (Tang et al., 2009; Wang et al., 2015), thereby regulating the grain-filling process. Abscisic acid, a kind of dominant hormone in rice senescence (Mao et al., 2017; Sakuraba et al., 2020), was documented to be enriched in the source tissues of ratoon rice at post-anthesis while displaying low levels in their counterparts of the single-cropping late rice (Zhou, 2020). These results indicated a potential ABA-dominated senescence with additional effects of carbon reserve remobilization and grain filling process underlying rice ratooning. Except for ABA-associated senescence, environmental stimuli are also a major contributing factor inducing the senescence process in rice (Yang et al., 2001a; Yang et al., 2001b; Yang et al., 2002; Kim et al., 2011; Prathap et al., 2019; Ren et al., 2021). Indeed, controlled environmental stress helps in initiating the remobilization of carbon reserves and thereby greatly contributes to an increase in rice yield and grain quality (Yang et al., 2003; Yang et al., 2004). However, ratoon rice might also exhibit poor grain filling as traditionally-cultivated rice, particularly in the inferior spikelets (Yang et al., 2000; Ishimaru et al., 2003), which often display a stagnant status from 5 to 15 days after flowering (Zhang et al., 2019). Furthermore, Yang and Zhang (2010) demonstrated that the grain average weight and the average filling rate of inferior spikelets were 20.9% and 20.7% lower than those of superior spikelets, respectively. Besides, the poor activity of the involved enzymes in sucrose-to-starch metabolism (Mohapatra et al., 2009; Zhang et al., 2012) and lower hormone levels of ABA (Nonhebel and Griffin, 2020) are the major factors that contribute to poor grain filling. It has been previously documented that microRNA expression in seed development may cause poor grain filling of rice (Peng et al., 2014). Teng et al. (2022) demonstrated that starch synthesis and phytohormone biosynthesis were affected by differentially expressed microRNA leading to a decrease in rice yield. However, this standpoint currently lacks further support based on genetic evidence. In contrast, our research documented that the GF14f isoform of 14-3-3 proteins could be considered the hub of the regulatory network responsible for the poor grain filling in rice (Zhang et al., 2014; Zhang et al., 2015b; Zhang et al., 2017). Recently, the molecular mechanism has revealed that GF14f negatively affects rice grain filling in addition to interacting with key enzymes in sucrose-to-starch conversion (Zhang et al., 2019). Interestingly, the GF14f gene (encoding GF14f isoform of 14-3-3 proteins) was weakly expressed in the grains of ratoon rice during the time course of 7 to 28 days after flowering while taking a turn for highly up-regulated expression at 35 days, as compared to its counterparts in both early-season rice and late-season rice (Supplementary Figure S1). These results indicated the potential associations between differentially expressed GF14f and the grain filling process underlying rice ratooning.
Therefore, in this study, we analyzed the rice yield and grain quality comparing early-season rice, ratoon rice, and late-season rice with additional evaluation of carbon reserves remobilization. A further aim was to elucidate the key roles the GF14f gene has on carbon reserves remobilization, grain filling properties, starch composition, and structure. Finally, our aim was to understand whether gene-dependent regulation by GF14f had an impact on rice yield and quality improvement under rice ratooning, irrespective of seasonal or environmental effects.
Materials and methods
Experimental design and materials
GF14f-RNAi line, GF14f mutant, and their WT (Jinhui-809) were grown at the Experiment Station of Fujian Agriculture and Forestry University, Fuzhou, Fujian, China (119.280E, 26.080N). The GF14f-RNAi line was obtained by transgenic technology as previously described (Zhang et al., 2019), displaying a specific suppression of GF14f gene expression. The GF14f mutant was obtained from the T-DNA-inserted japonica line Dongjin, displaying the loss in expression of the GF14f gene (http://signal.salk.edu/cgi-bin/RiceGE). The suppression degree of the GF14f-RNAi line and GF14f mutant was investigated using qRT-PCR (Figure S2). Field trials were performed from March 2019 to October 2019 and repeated from March 2020 to October 2020. Weather data from 2019 and weather data from 2020 are provided in Supplementary Figures S3–S4. To provide evidence for revealing the key role of the GF14f gene in ratoon rice, the GF14f-RNAi line, GF14f Mutant, and the corresponding WT were specifically grown as ratoon rice and late-season rice, having the same or different genetic background and having a synchronized heading time. Planting and fertilization were conducted as in our previous research (Lin et al., 2022). These plant materials were provided by Fujian Provincial Key Laboratory of Agroecological Processing & Safety Monitoring, Fujian Agriculture and Forestry University, Fuzhou 350002, China.
Evaluation of the ability of carbon reserve remobilization
The harvested rice plants were sampled at the heading stage and at maturity, respectively. Then, the plant materials were further divided into four tissue parts, which contained roots, stems, leaves, and grains. Here, the whole root, whole stem, whole leaf, and full-filled grains were respectively collected with their three replications to form a composite sample. Three composite samples were used as the three biological replications in the following experiments. Then, the samples were immediately dried in a forced-air dryer at 80 °C to constant weight for the estimation of dry matter weight (Ying et al., 1998). Stems and leaves were freeze-dried and ground to a fine powder for the estimation of non-structural carbohydrates (NSC). Total soluble sugar content was measured by a colorimetric method of anthracenone determination (Yang et al., 2001b; Wang et al., 2020). Total starch content was measured spectrophotometrically (Li and Peng, 2018). The absorbance was measured at 630 nm using a spectrophotometer (Model 340, Sequoia-Turner Co., Taiwan). Total NSC content was calculated as the sum of total soluble sugar and total starch. Other calculation formulas are as follows. Here, the transferable dry matter and transferable NSC were respectively referred to as the reduction of dry matter and the reduction of NSC in source tissues between the heading stage and the mature stage.
Evaluation of yield performance and grain quality
At maturity, rice plants with uniform morphological features were harvested. Each panicle that had a seed setting percentage of over 50% was considered a productive panicle and grain weight was calculated based on 200 grains and was converted to 1,000-grain weight (Li et al., 2014). The seed-setting percentage, per-unit yield, and harvest index were calculated as previously described (Huang et al., 2020; Lin et al., 2022). Seed-setting percentage (%) = filled grains/(filled grains + partially filled grains + unfilled grains) × 100%, per-unit yield (t·ha-1) = total rice yield/whole-field area and harvest index (%) = grain dry weight/biological yield of overground parts × 100%. The panicles that were headed on the same day were tagged and then used to calculate the grain number per spike. The annual rice yield of ratoon rice was composed of the yield of early-season rice and the yield of ratoon rice. Daily average yield = per-unit yield/duration of the growing period.
Brown rice yield, milled rice yield, and head rice yield were counted according to the “Chinese agricultural standard: NY/T 83-2017” (Ministry of Agriculture of the PRC, 2017). Chalky grain percentage and chalkiness degree were measured using a grain scanner (ScanMaker i800 plus, Microtek, Shanghai) according to the manufacturer’s instructions. Chalky grain percentage = (numbers of chalky grains/numbers of total grains) × 100%. Chalkiness degree = (sum of chalky area/sum of whole grain area) × 100%.
Assay of starch composition in the endosperm
Amylose content was measured using a continuous flow analytical system (Skalar San++ System, Netherlands). Standards were prepared using potato amylose (Solarbio, China) with a concentration gradient of 2%, 4%, 8%, 12%, 16%, 20%, and 32%. The absorbance of amylose content was determined at 600 nm. Total starch content was measured spectrophotometrically based on the determination of the absorbance of glucose concentration at 540 nm (Li and Peng, 2018). According to the transformational equation between starch and glucose, total starch content was calculated by the following formula: Total starch content (mg·g-1) = glucose concentration × 10 × 9.11 ml × 0.9/(50 × 1000). Amylopectin content = total starch content - amylose content (Prathap et al., 2019).
Scanning electron microscopy analysis of the endosperm fine structure
According to the method described by Li et al., 2014, milled rice grains were cut in the middle and coated with gold under vacuum conditions. The starch morphology in the belly part of the endosperm was examined using a scanning electron microscope (Phenom ProX, FEI NanoPorts, America) at an accelerating voltage of 10 kV and a spot size of 30 nm.
Transcriptomic and bioinformatics analysis
The GF14f mutant should be more genetically stable in the disturbing function of this gene than the GF14f-RNAi line. Therefore, we used the rice grains which were sampled from the GF14f mutant and its WT Dongjin for the following RNA sequencing. Total RNA was extracted from these two rice lines on the 7th day after flowering. Grains from ten tagged panicles were collected and combined into an independent biological replication for each sample (at least 3 replicates). Total RNA was extracted using a TRIzol reagent (TransGen Biotech, China) and double-stranded cDNA was synthesized and purified using a QIAquick PCR extraction Kit (QIAGEN Inc., USA). The size-selected DNA of PCR products was ligated by pulse-field gel electrophoresis. We constructed cDNA libraries and completed Illumina sequencing (Illumina Hiseq™ 2500, America) at Majorbio company, China.
For further bioinformatic analysis, the reads with > 50% low-quality bases (Q-value of ≤30) or >10% of unknown nucleotides were removed (Lin et al., 2022). Transcriptome assembly was performed using the short-read assembly program Trinity (Grabherr et al., 2011). The unigene expression abundance was normalized to reads per kb per million reads (RPKM) using the following formula: RPKM = (1,000,000 × C)/(N × L/1000). Here, C is the number of reads that are uniquely mapped to one unigene, N is the total number of reads that are uniquely mapped to all unigenes and L is the length (base number) of one unigene (Fang et al., 2017). Further analysis and graphing were performed according to our previous research (Lin et al., 2021; Lin et al., 2022). More specifically, the edgeR package (http://www.r-project.org/) was used to identify differentially expressed genes (DEGs). Target proteins of DEGs were collectively annotated according to their numeric order in the GO database (http://www.geneontology.org/). GO terms with a p-value< 0.05 and an FDR< 0.05 were defined as the significant terms. GO enrichment analysis displayed the key DEGs that were clustered into their corresponding GO terms (https://www.omicshare.com).
Assay of H2O2 concentration, production ratio, and MDA content
The crude proteins of grain samples were extracted at 4°C, and then H2O2 concentration, production rate, and MDA concentration were determined according to previously published methods (Ke et al., 2007; Chakrabarty and Datta, 2008).
Data processing and statistical analysis
SPSS v. 25.0 (IBM Corp., Armonk, NY, USA) and Origin pro 2021 (OriginLab Corp., Northampton, MA, USA) were used for data processing and analysis of variance (ANOVA). Significant differences were evaluated with a p-value< 0.05.
Results
An extensive carbon reserve remobilization induced by rice ratooning
The ability to remobilize the carbon reserves significantly contributes to rice yield and grain quality. Here, both dry matter translocation efficiency and contribution of dry matter assimilation to grains in the different source tissues of ratoon rice were higher than in their counterparts in early-season rice and those in late-season rice. In 2019, the dry matter translocation efficiency in the root, stem, and leaf of the ratoon rice significantly (P< 0.05) increased by 7.9%, 24.4%, and 40.2%, respectively, as compared to early-season rice. Whereas, those parameters significantly (P< 0.05) increased by 9.0%, 37.5%, and 41.5% when compared to late-season rice. The contribution of dry matter assimilation to grains in the root, stem, and leaf of the ratoon rice significantly (P< 0.05) increased by 1.3%, 2.8%, and 2.4%, respectively, as compared to early-season rice. Furthermore, those parameters significantly (P< 0.05) increased by 1.1%, 2.9%, and 1.9% when compared to late-season rice. In 2020, a similar scenario was observed in the dry matter translocation efficiency and contribution of dry matter assimilation to grains, which were higher in the ratoon rice than those of early-season rice and of late-season rice. Consistently, both NSC translocation efficiency and contribution of NSC to grains in the different source tissues of the ratoon rice were also higher than in their counterparts in early-season rice and those in late-season rice. In 2019, the NSC translocation efficiency in the root, stem, and leaf of the ratoon rice significantly (P< 0.05) increased by 11.4%, 15.0%, and 16.5%, respectively, as compared to early-season rice. In contrast, those parameters significantly (P< 0.05) increased by 6.4%, 22.0%, and 13.0% when compared to late-season rice. In addition, the contribution of NSC to grains in the root, stem, and leaf of the ratoon rice significantly (P< 0.05) increased by 4.7%, 1.5%, and 2.0% when compared to early-season rice, while significantly (P< 0.05) increased by 4.1%, 1.7%, and 1.6% when compared to late-season rice. In 2020, the NSC translocation efficiency and the contribution of NSC to grains significantly (P< 0.05) increased in the root, stem, and leaf of the ratoon rice as compared to their counterparts in early-season rice and late-season rice (Table 1).
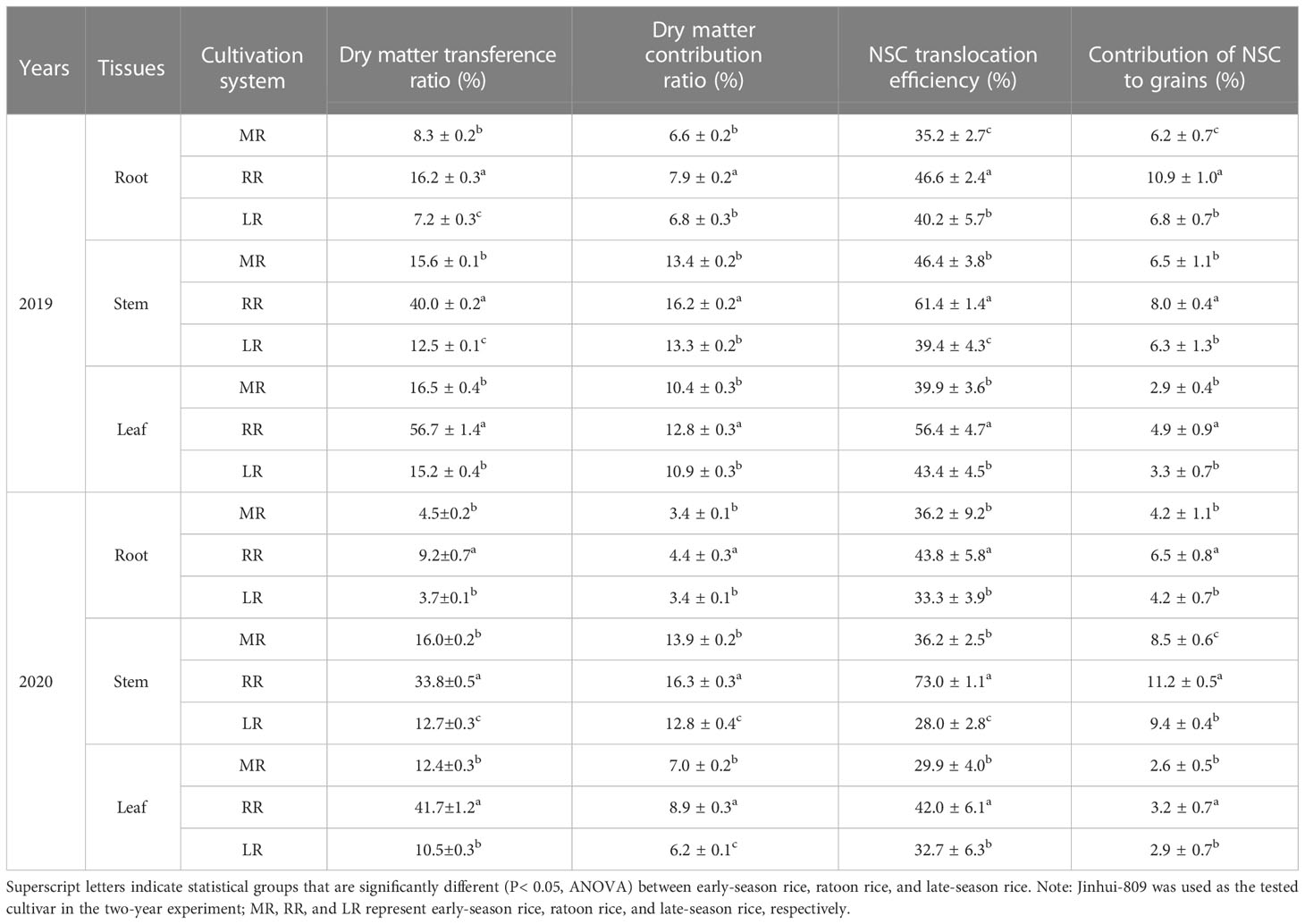
Table 1 Comparison of carbon reserve remobilization between early-season rice, ratoon rice, and late-season rice.
These results suggested that rice ratooning could induce an extensive carbon reserve remobilization, thereby providing the grains with more carbon substances.
Yield performance and grain quality improvement underlying rice ratooning
In our work, yield performance varied significantly between early-season rice, ratoon rice, and late-season rice. Between these treatments, panicle number, grain number per spike, 1000-grain weight, and per-unit yield all decreased in the ratoon rice. In contrast, the seed-setting percentage and harvest index of ratoon rice turned out to be much higher than those of early-season rice and of late-season rice. Furthermore, the results showed that the daily average yield of the ratoon rice was significantly (P< 0.05) higher than the daily average yield of early-season rice and of late-season rice. In 2019, the daily average yield of ratoon rice significantly (P< 0.05) increased by 28.1% and 28.6% when compared to early-season rice and late-season rice, respectively. And in 2020, the daily average yield of ratoon rice significantly (P< 0.05) increased by 16.6% and 22.6% when compared to main and late crops. It has also been found that the annual rice yield of ratoon rice (composed of main + ratooning crops) was significantly (P< 0.05) higher than the yield of late-season rice. In detail, the annual rice yield of ratoon rice significantly (P< 0.05) increased by 60.5% in 2019 and increased by 62.4% in 2020 (Table 2).
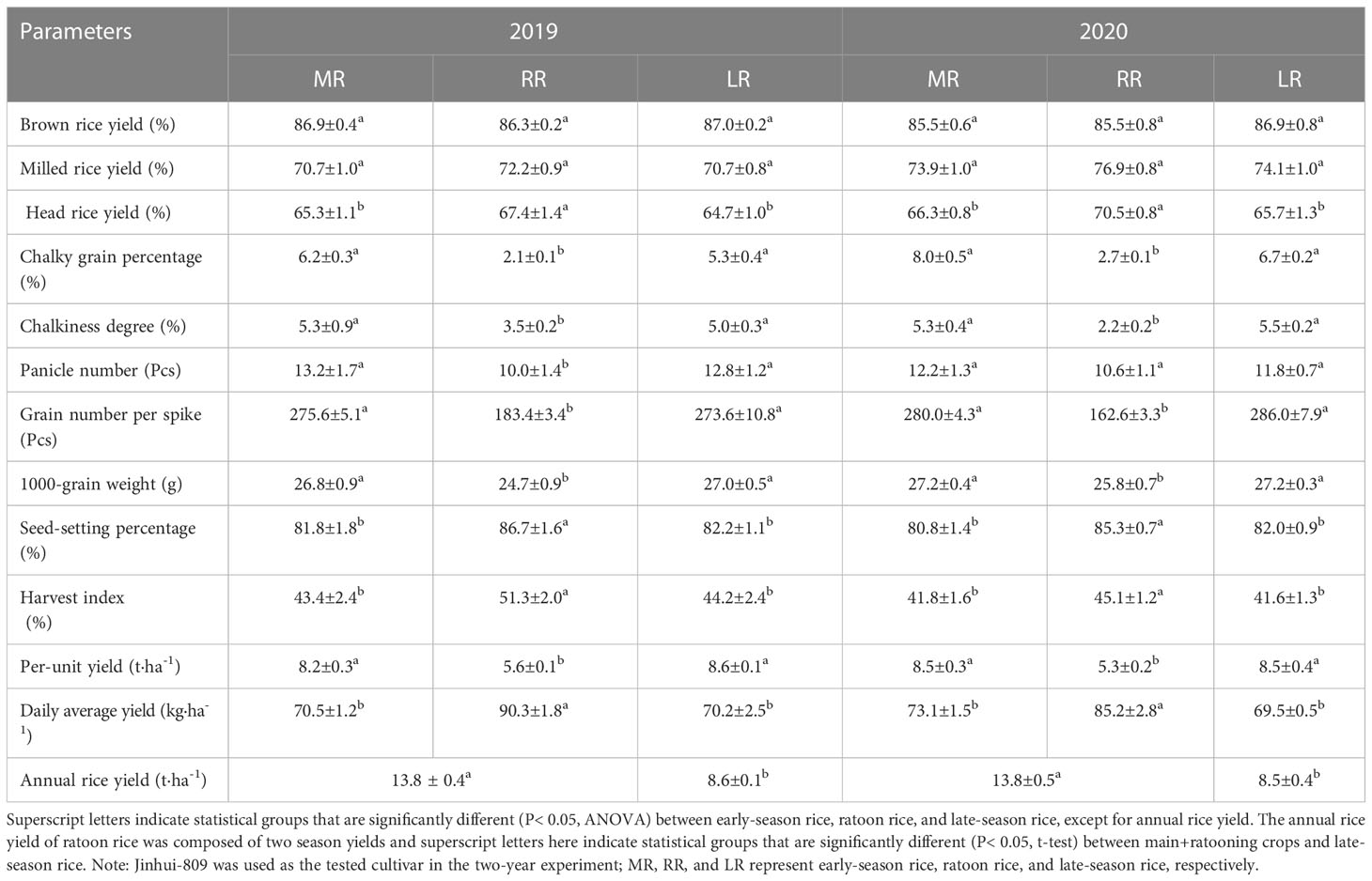
Table 2 Comparison of grain quality and yield performance between early-season rice, ratoon rice, and late-season rice.
In addition, the key parameters of rice quality, such as brown rice yield, milled rice yield, head rice yield, chalky grain percentage, and chalkiness degree, were thoroughly investigated. In 2019, the head rice yield of the ratoon rice significantly (P< 0.05) increased by 2.1% and 2.3% when compared to early-season rice and late-season rice, respectively. However, the chalky grain percentage of the ratoon rice significantly (P< 0.05) reduced by 4.1% and 3.2% when compared to its counterparts of main and late crops. Likewise, the chalkiness degree of the ratoon rice significantly (P< 0.05) reduced by 1.8% and 1.5% when compared to early-cropping season and late-season rice, respectively. Consistently, in 2020, the head rice yield of the ratoon rice was significantly (P< 0.05) higher than the head rice yield of early-season rice and of late-season rice. In contrast, the chalky grain percentage and the chalkiness degree of ratoon rice were significantly (P< 0.05) lower than in their counterparts of early-season rice and late-season rice. Finally, the data did not display any statistical or biological differences in either brown rice yield or milled rice yield between early-season rice, ratoon rice, and late-season rice (Table 2).
In general, our results suggested distinct progress in improving head rice yield and grain chalkiness under rice ratooning. Moreover, the daily average yield of the ratoon rice was improved significantly by depending on the shorter growing period with the additional advantage of increasing the annual rice yield of ratoon rice.
GF14f gene impacts on yield performance and grain chalkiness improvement underlying rice ratooning
As was previously described above, per-unit yield in combination with panicle number, grain number per spike, and 1000-grain weight all performed at lower levels in the ratoon rice than their counterparts in late-season rice. However, these yield-related parameters strongly increased in either the GF14f-RNAi line or the GF14f mutant, respectively, as compared to their corresponding WT. In detail, panicle number, grain number per spike, 1000-grain weight as well as seed-setting percentage, harvest index daily average yield, and per-unit yield in ratoon rice of the GF14f-RNAi line significantly (P< 0.05) increased by 42.0%, 24.9%, 5.3% 6.3%, 4.3%, 7.3%, and 28.6% when compared to those in ratoon rice of the WT (Jinhui-809). Likewise, those yield-related parameters significantly (P< 0.05) increased by 25.0%, 92.5%, 7.6%, 3.1%, 4.0%, 21.7%, and 27.2% in ratoon rice of the GF14f mutant as compared to its WT (Dongjin) (Figures 1A–G).
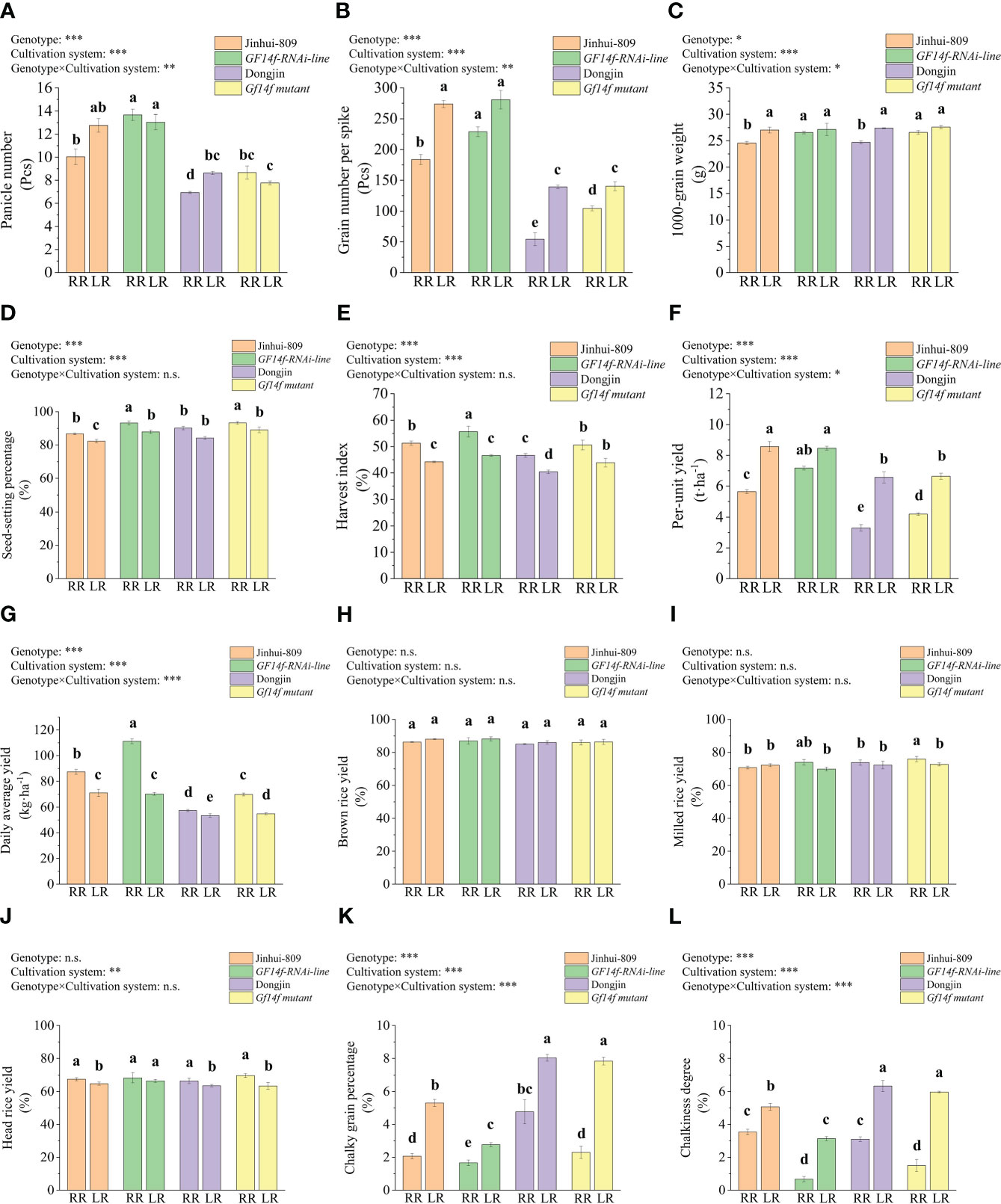
Figure 1 Comparisons in grain quality and yield performance between GF14f-RNAi line, GF14f mutant and their WT. (A): Panicle number; (B): Grain number per spike: (C): 1000-grain weight; (D): Seed-setting percentage: (E): Harvest index; (F): Per-unit yield; (G): Daily average yield; (H): Brown rice yield: (I): Milled rice yield: (J): Head rice yield: (K): Chalky grain percentage: (L): Chalkiness degree. Note: MR. RR and LR represent early-season rice, ratoon rice and late-season rice, respectively. Superscript letters indicate statistical groups that are significantly different (P< 0.05, ANOVA). Asterisks indicate factors contributing to the differences between samples (n.s., not significant; *P< 0.05; **P< 0.01; ***P< 0.001).
In addition, the data did not display statistical and biological differences in brown rice yield, milled rice yield, and head rice yield between the GF14f-RNAi line, GF14f mutant, and their WT when grown as ratoon rice (Figures 1H–J). However, the low levels of chalky grain percentage and chalkiness degree in ratoon rice of the WT were significantly (P< 0.05) reduced by 0.4% and 2.8% in ratoon rice of the GF14f-RNAi line. Consistently, chalky grain percentage and chalkiness degree were significantly (P< 0.05) reduced by 2.5% and 1.6% in ratoon rice of the GF14f mutant as compared to those in ratoon rice of its WT. Chalky grain percentage and chalkiness degree in late-season rice of the WT also significantly improved (P< 0.05) when the GF14f gene function was suppressed (Figures 1K–L). Furthermore, ANOVA analysis indicated that most of the evaluative parameters (except brown rice yield, milled rice yield, and head rice yield) were simultaneously significantly influenced by two factors, namely cultivation system and genotype. The changes occurring in panicle number, grain number per spike, 1000-grain weight, per-unit yield, daily average yield, chalky grain percentage, and chalkiness were even affected by the cultivation system, genotype, and interaction effects between cultivation system and genotype (Figure 1).
Thus, these results suggested that yield performance and grain quality improvement underlying rice ratooning were strongly dependent on the GF14f, which negatively affected yield performance and grain chalkiness.
GF14f gene impacts on carbon reserve remobilization and sucrose-to-starch biosynthesis underlying rice ratooning
As previously described, dry matter translocation efficiency and dry matter assimilation from root to grains were displayed at higher levels in the ratoon rice as compared to their low levels in late-season rice. A significant (P< 0.05) increase in dry matter translocation efficiency and dry matter assimilation to grains were further achieved in the root of the GF14f-RNAi line and the GF14f mutant, whether grown as ratoon rice or late-season rice, as compared to those in their counterparts of the WT. Moreover, NSC translocation efficiency and contribution of NSC from either stem or leaf to grains also displayed distinct improvements in the ratoon rice of the GF14f-RNAi line and the GF14f mutant, respectively (Figure 2A). During the grain-filling stage, the key enzymes in the sucrose-to-starch biosynthesis, which contained ADPGase, soluble starch synthase, and starch branching enzyme, reached greatly higher levels in not only ratoon rice but also in late-season rice when the GF14f gene was suppressed (Figure 2B).
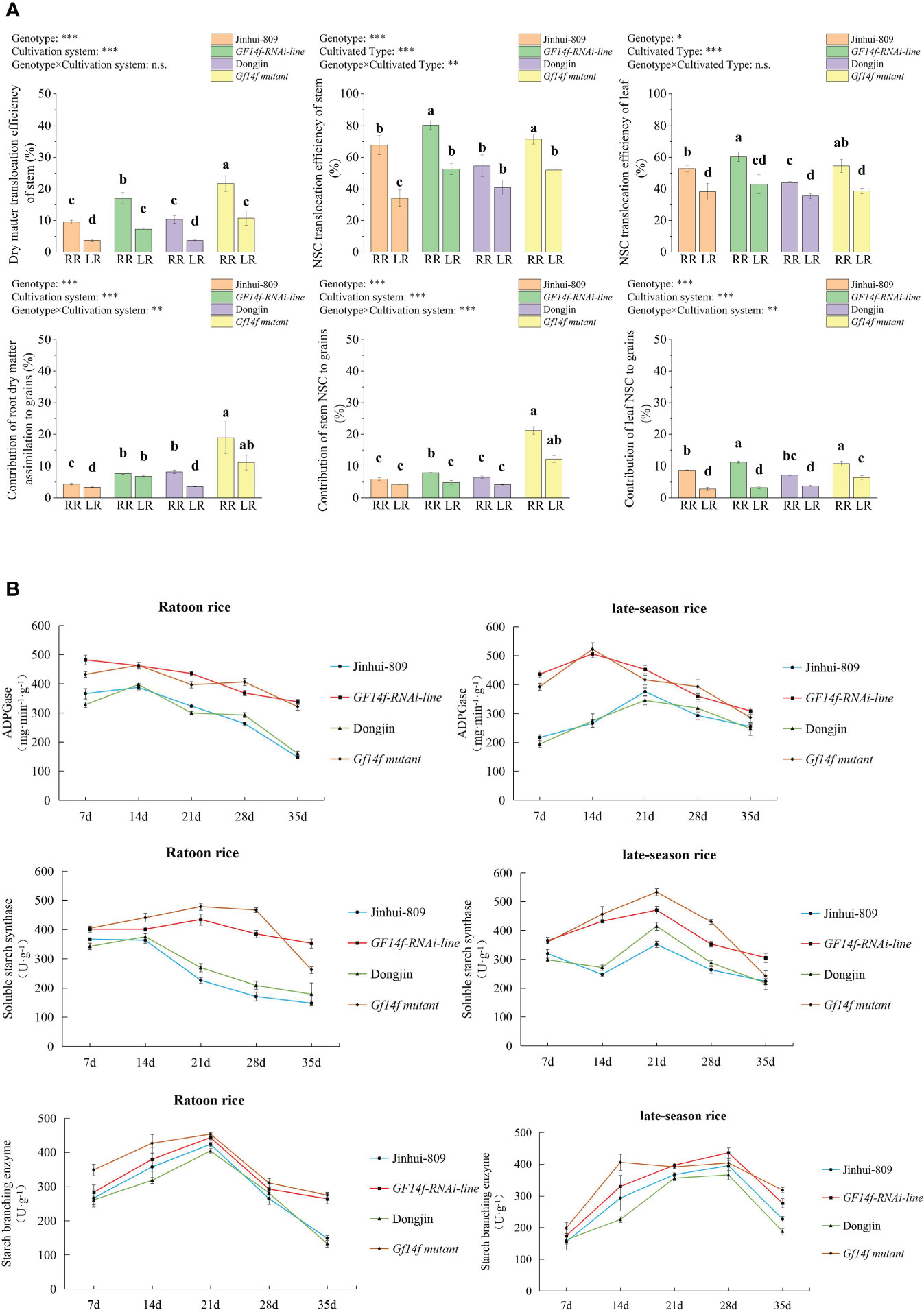
Figure 2 Comparisons in carbon reserve remobilization and sucrose-to-starch biosynthesis between GF14f-RNAi line, GF14f mutant and their WT. (A): Carbon reserve remobilization in source tissues was mediated by the presence of the GF14f gene. Note: MR, RR and LR represent early-season rice, ratoon rice and late-season rice, respectively. Superscript letters indicate statistical groups that are significantly different (P< 0.05, ANOVA). Asterisks indicate factors contributing to the differences between samples (n.s., not significant; *P< 0.05; **P< 0.01; ***P< 0.001). (B): Starch biosynthesis were enhanced by suppression of the GF14f gene. ADPGase, soluble starch enzyme and starch branching enzyme were essential for sucrose-to-starch biosynthesis.
Starch is the main substance for rice grain and scattered starch granules are the main contributing factor leading to poor grain quality. Here, amylose content, amylopectin content, and amylose/amylopectin ratio in the ratoon rice of the GF14f-RNAi line significantly (P< 0.05) increased by 11.3%, 8.3%, and 17.4%, respectively, when compared to those in the ratoon rice of the WT. Amylose content, amylopectin content, and amylose/amylopectin ratio in the ratoon rice of the GF14f mutant significantly (P< 0.05) increased by 9.0%, 8.1%, and 16.2% as compared to its WT (Figures 3A–C). Further analysis revealed that the ratoon rice of the GF14f mutant contained lower numbers and surface areas of scattered starch granules in the endosperm, displaying a complete and compact starch structure in contrast to the WT (Figures 3D–K).
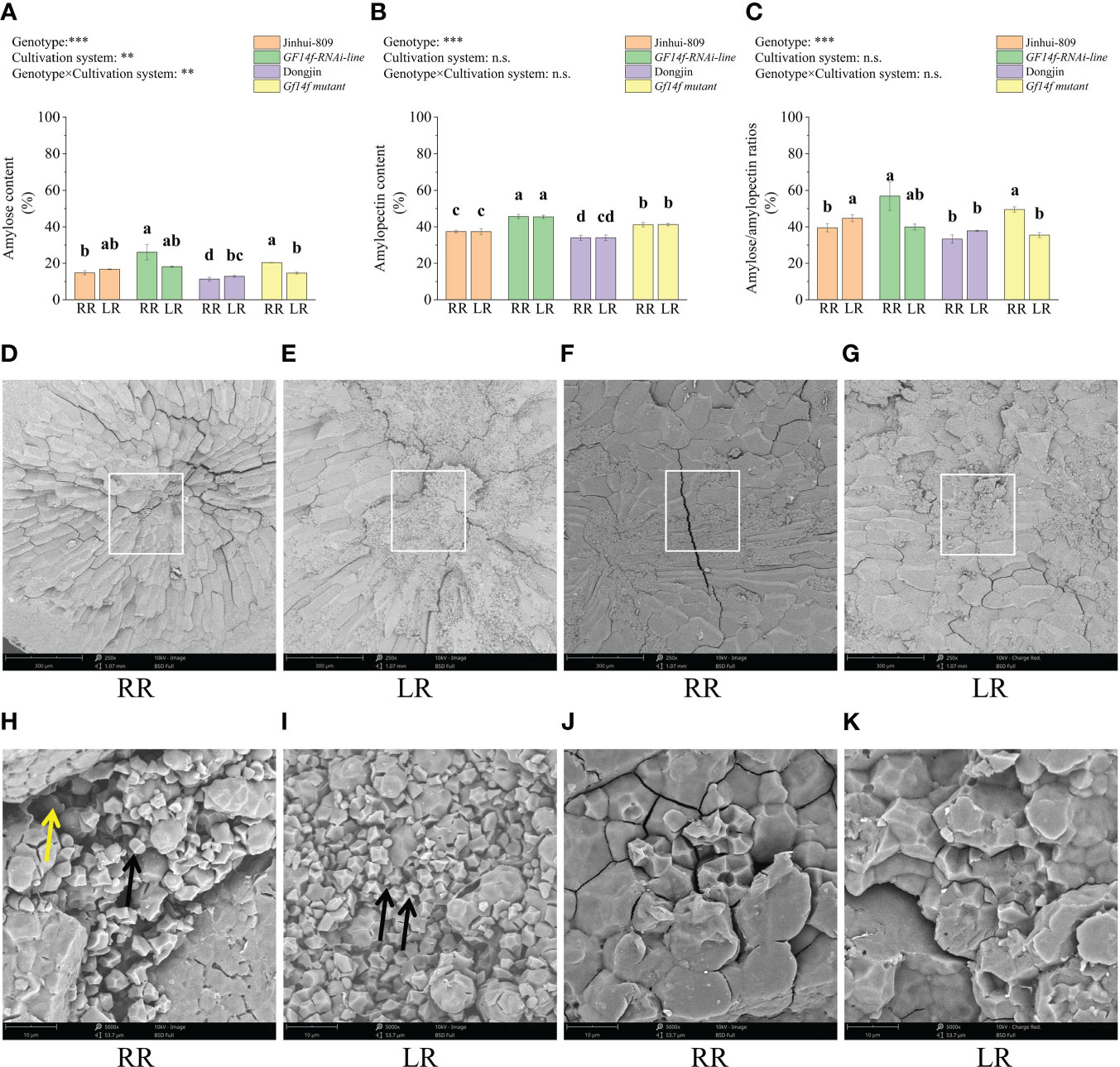
Figure 3 Comparisons in starch composition and scanning electron microscope observation in endosperm structure. (A–C): Amylose, amylopectin and amylose/amylopectin ratio that varied between GF14f-RNAi line, GF14f mutant and their WT; (D–K): Scanning electron microscopy images of the starch granules in endosperm. Positions marked by rectangles in the middle bellies of the endosperm. Scale bars: 250 times magnificention (top); 5000 times magnificention (bottom). Black arrows point loosened starch granules and yellow arrows point the loss of compositional parts of starch. MR, RR and LR represent early-season rice, ratoon rice and late-season rice, respectively.
Thus, these results indicated the regulatory roles of the GF14f gene negatively impacting carbon reserve remobilization and starch accumulation in the ratoon rice.
GF14f gene modulates the gene-dependent plant resistance under rice ratooning
We further investigated the regulatory roles for the GF14f encoded regulator underlying rice ratooning using transcriptome analysis. As a result, there were 948 differentially expressed genes (DEGs) significantly (|Fold change| > 2, P< 0.05) varying between ratoon rice and late-season rice in the WT (Dongjin). However, 340 DEGs that were significantly (|Fold change| > 2, P< 0.05) different could be detected when comparing ratoon rice and late-season rice using the GF14F Mutant. Among these, 252 DEGs were highly differentially expressed in both the GF14F Mutant and the WT (Figure 4A). Furthermore, the 252 DEGs were clustered into their corresponding GO terms (Top 10) using GO enrichment analysis. Among these, all the GO terms were involved in the processes of response to environmental stimuli and stress (Figure 4B). Thus, the differential expression of these functional genes could be considered as the potential cause leading to differentiation in stimuli-related plant resistance.
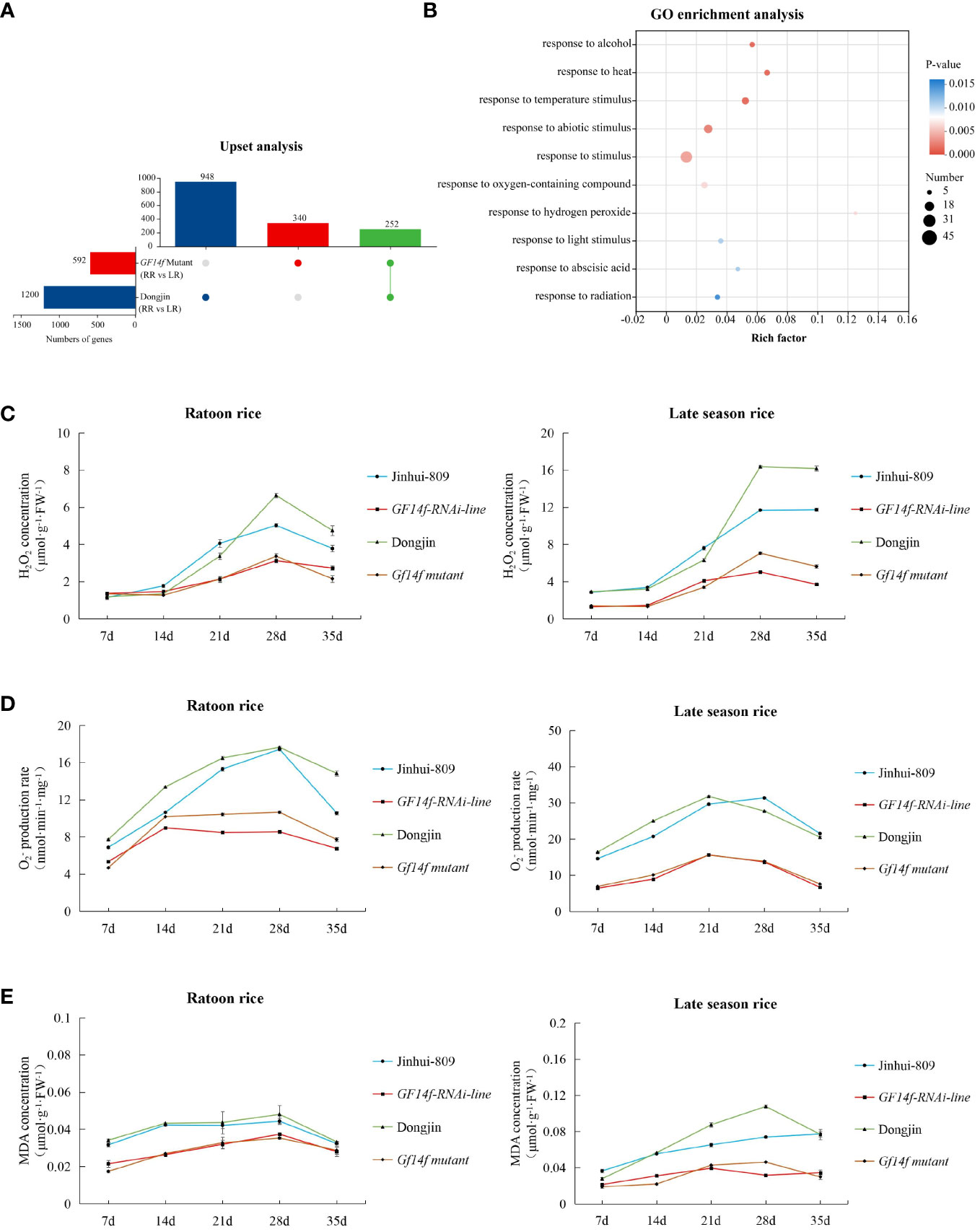
Figure 4 GF14f gene modulates the gene-dependent response to environmental stimuli underlying rice ratooning (A) Up-set analysis of GF14 mutant and its WT. (B): Go enrichment analysis (TOP 10) of the 252 key DEGS which were differentially expressed in the pathways of both the GF14f mutant and the corresponding WT. GO database (http://www.geneontology.org/) was used to annotate the DEGs. The data showing the differentially expressed genes were visualized using Circos (Version 0.69, http://circos.ca/). Each gene was classified as belonging to their sample groups and was assigned to one special color in chordal diagram. (C) Temporal pattern of H2O2 concentration during grain-filling stage. (D) Temporal pattem of production rate during grain-filling stage. (E) Temporal pattern of MDA concentration during grain-filling stage.
To further test the impact of the presence of the GF14f on plant resistance, the H2O2 concentration, production rate, and MDA concentration in rice grains were investigated. It could be shown that either the GF14f-RANi line or the GF14f mutant accumulated ROS and MDA during the grain-filling stage at very low levels. Interestingly, H2O2 concentration, production ratio, and MDA concentration in the late-season rice displayed lower levels when the GF14f gene was suppressed but showed much higher levels when monitoring their levels in late-season rice of the WT (Figures 4C–E). Furthermore, we performed qRT-PCR to investigate the differential gene expression of key genes regarding transcriptome analysis as described above. The results displayed that CAT-A, CAT-B, CAT-B (which encode catalase), OsAPX1, OsAPX2, OsAPX4 (which encode ascorbate peroxidase), and OsGPX1, OsGPX2, OsGPX4 (which encode glutathione peroxidase) greatly increased in the ratoon rice of the GF14f mutant when compared to those in the ratoon rice of the WT (Figures S5A–I).
Thus, these results indicated the essential roles of the GF14f gene in impacting plant resistance and ROS removal in the ratoon rice.
Discussion
Ratoon rice cropping contributes to high rice yield and higher quality that can satisfy the present market demands (Xu et al., 2021), thereby increasingly becoming popular in worldwide rice production (Nakano et al., 2020; Wang et al., 2021; Xu et al., 2022). However, the potential mechanism affecting yield performance and grain quality underlying rice ratooning needs to be further investigated. In this study, a key gene, GF14f, impacting rice yield and quality as the regulatory hub in ratoon rice was explored for the first time. Furthermore, we discussed the essential roles and implications of how the GF14f gene impacts rice yield and grain quality under rice ratooning, irrespective of seasonal or environmental effects.
Carbon reserves remobilization is the basis for the grain filling of cereals leading to source-to-sink transport (Yang et al., 2006). Our finding demonstrated that rice ratooning was able to initiate extensive remobilization of carbon reserves to provide the grains with more carbon substances. Therefore, current knowledge (Yang et al., 2001a; Yang et al., 2001b; Wang et al., 2020) allows us to infer the following scenario—yield performance and grain quality in the ratoon rice are predicted to achieve higher levels. In our work, chalky grain percentage and chalkiness degrees were highly improved when rice plants were grown as ratoon rice. This result indicated a distinct improvement in rice appearance quality under rice ratooning. However, per-unit yield in combination with panicle number, grain number per spike, and 1000-grain weight in the ratoon rice were significantly reduced when compared to those in the early-season rice and those in the late-season rice. It seems that rice ratooning in particular contributes to obtaining higher grain quality rather than higher yield. However, this is only true if applied universally without considering the growing period. Under normal circumstances, the duration of the growing phase of traditionally-cultivated rice was longer than the duration of the growing phase of ratoon rice (Wang et al., 2020; Xu et al., 2021). Rice ratooning initiated grain filling once the new spikes were regenerated from auxiliary buds on the residual stubbles (Lin et al., 2015; Lin, 2019), thereby reducing the duration of the vegetative growth period. We, therefore, determined the daily average yield of ratoon rice. Consequently, the daily average yield was highly improved when monitoring ratoon rice. In addition, seed-setting percentage and harvest index were both displayed at higher levels in the grains of ratoon rice than their levels in either early-season rice or late-season rice. Not only that, the annual rice yield of ratoon rice (composed of main + ratooning crops) was much higher than the yield of single-cropping late rice. Thus, ratoon rice cropping is especially applicable for greater rice production in Southern China because light and temperature resources are enough for one seasonal crop but insufficient for two seasons (Lin et al., 2015; Huang et al., 2020; Xu et al., 2022). Southern China, which makes the greatest contribution to China’s rice industry, has currently been constrained by labor shortage (Deng et al., 2019), water scarcity (Zhuang et al., 2021), negative environmental impacts (Zhang et al., 2015a; Shen et al., 2021), and a lower economic return (Yu et al., 2021). Therefore, our finding provides compelling evidence to highlight the significance of ratoon rice cropping in Southern China. Importantly, such reductions involving per-unit yield, panicle number, grain number per spike, and 1000-grain weight of ratoon rice were highly improved when the GF14f gene was thoroughly suppressed. Additionally, the original high-quality of ratoon rice was further improved when investigating the GF14f-RNAi line and the GF14f mutant. A further significance was to observe how it was possible to achieve higher levels of yield performance and grain quality of ratoon rice via suppression of the GF14f gene. Furthermore, all analyses were conducted based on the comparison between ratoon rice and late-season rice, having the same or a different genetic background and the synchronized rice heading time so as to remove the seasonal and environmental effects. Therefore, our finding provided the basis for a hypothesis that the gene-dependent effect by the GF14f determines the yield performance and grain quality underlying rice ratooning.
Grain filling of cereals is an essential process catalyzed by a series of enzymes from sucrose-to-starch biosynthesis (Yang et al., 2003; Yang et al., 2004; Tang et al., 2009). Carbon substances were quickly transferred from source tissues into grains (Yang et al., 2001a; Yang et al., 2001b). In our work, the carbon reserves remobilization in the ratoon rice of the GF14f-RNAi line and the GF14f mutant were also further improved when compared to their WT. Meanwhile, the key enzymes in sucrose-to-starch biosynthesis were kept at high levels during the whole grain-filling stage when the GF14f gene was suppressed. It should be noted that high yield and rice quality are positive results ensuing from a continued high enzymatic activity of the key enzymes in starch biosynthesis (Yang et al., 2003; Yang et al., 2004; Yang et al., 2006). In addition, it was previously documented that starch is the most abundant and essential element in rice grain and that it greatly affects grain quality (Burrell, 2003; Bao, 2019), and this trait was influenced by starch composition, structure, and properties (Gu et al., 2019; Peng et al., 2021; Zhu et al., 2021). Furthermore, the currently available data in our work suggested that starch compositions varied significantly between the GF14f-RNAi line, the GF14f mutant, and their corresponding WT and, ultimately, displayed lower numbers and surface areas of scattered starch granules in the endosperm of both the GF14f-RNAi line and the GF14f mutant. It has been documented that lower numbers and surface areas of scattered starch granules in combination with a lower amylose/amylopectin ratio significantly contributed to reduced grain chalkiness (Wani et al., 2012; Schirmer et al., 2013; Li et al., 2014; Lin et al., 2018). This is consistent with our findings, thereby indicating a negative regulatory role of the GF14f gene, which impacted starch composition and structure in the endosperm. Our findings, therefore, indicated that the GF14f negatively affected several essential processes in ratoon rice, namely carbon reserve remobilization, grain filling process, and starch biosynthesis. Nonetheless, the in-depth molecular mechanism associated with these processes needs to be further investigated. In this study, a transcriptome analysis of the GF14f mutant and its WT would provide a more comprehensive understanding of how the GF14f regulates the grain filling of ratoon rice at the transcriptional level. Consequently, the data was suggested to be involved in the process of stimuli-related plant resistance. Environmental stimuli are the main cause of poor grain filling (Lawas et al., 2018; Yao et al., 2020), and oxidative and environmental stress were easily induced by many environmental stimuli, which led to excessive accumulation of ROS and MDA (Kaneko et al., 2016; Sharma and Sharma, 2018). In addition, carbon reserve remobilization and grain filling are long-term processes with accompanying cell metabolism, thereby producing several toxic compounds such as ROS. These were especially true when examining the loss of rice yield and grain quality (Xing and Zhang, 2010; Hakata et al., 2012; Lo et al., 2016; Suriyasak et al., 2017). However, both the GF14f-RNAi line and the GF14f mutant were better at increasing oxidative and environmental stress resistance as compared to their WT via reducing ROS accumulation and gene expression of the involved gene. Our findings, therefore, indicated that the GF14f gene can modulate the gene-dependent plant resistance and ROS removal in grains underlying rice ratooning. In addition, we also investigated the key genes that were documented to be essential for the chalky grain process and yield-related process. As a result, these key genes were also highly expressed in the GF14f mutant using a qRT-PCR analysis.
In summary, this study revealed the yield performance and grain chalkiness improvement underlying rice ratooning. Such processes were further shown to be associated with a key gene, GF14f. The GF14f had a negative impact on many aspects ranging from carbon reserve remobilization, starch biosynthesis, stimuli-related plant resistance, and ROS removal. The yield performance and grain quality were, therefore, dependent on the GF14f gene, irrespective of seasonal or environmental effects.
Data availability statement
The original contributions presented in the study are included in the article/Supplementary Material. Further inquiries can be directed to the corresponding author.
Author contributions
FL and WL contributed to the conception of the study; FL performed the experiment; SL and ZZ contributed to the manuscript preparation; FL performed the data analyses and wrote the manuscript; CR and DX helped to work out the conception and analysis with constructive discussions. They also helped revise the initial draft. All authors contributed to the article and approved the submitted version
Funding
This work was supported by the National Key Research and Development Program of China (2016YFD0300508, 2017YF-D0301602, 2018YFD0301105) and the National Natural Science Foundation of China (No. 31871542).
Conflict of interest
The authors declare that the research was conducted in the absence of any commercial or financial relationships that could be construed as a potential conflict of interest.
Publisher’s note
All claims expressed in this article are solely those of the authors and do not necessarily represent those of their affiliated organizations, or those of the publisher, the editors and the reviewers. Any product that may be evaluated in this article, or claim that may be made by its manufacturer, is not guaranteed or endorsed by the publisher.
Supplementary material
The Supplementary Material for this article can be found online at: https://www.frontiersin.org/articles/10.3389/fpls.2023.1112146/full#supplementary-material
References
Burrell, M. M. (2003). Starch: the need for improved quality or quantity–an overview. J. Exp. Bot. 54, 451–456. doi: 10.1093/jxb/erg049
Chakrabarty, D., Datta, S. K. (2008). Micropropagation of gerbera: lipid peroxidation and antioxidant enzyme activities during acclimatization process. Acta Physiol. Plant. 30, 325–331. doi: 10.1007/s11738-007-0125-3
Cruz-Aguado, J. A., Reyes, F., Rodes, R., Perez, I., Dorado, M. (1999). Effect of source-to-sink ratio on partitioning of dry matter and 14 c-photoassimilates in wheat during grain filling. Ann. Bot. 83, 655–665. doi: 10.1006/anbo.1999.0869
Deng, N. Y., Grassini, P., Yang, H. S., Huang, J., Cassman, K. G., Peng, S. (2019). Closing yield gaps for rice self-sufficiency in China. Nat. Commun. 10, 1725. doi: 10.1038/s41467-019-09447-9
Deng, F., Yang, F., Li, Q., Zeng, Y., Li, B., Zhong, X, et al. (2021). Differences in starch structural and physicochemical properties and texture characteristics of cooked rice between the main crop and ratoon rice. Food Hydrocolloids 116, 106643. doi: 10.1016/j.foodhyd.2021.106643
Fang, C., Zhang, P., Jian, X., Chen, W., Lin, H., Li, Y, et al. (2017). Overexpression of Lsi1 in cold-sensitive rice mediates transcriptional regulatory networks and enhances resistance to chilling stress. Plant Sci. 262, 115–126. doi: 10.1016/j.plantsci.2017.06.002
FAO (2020) Food and agriculture organization of the united nations. crop production. Available at: http://www.fao.org/faostat/en/#data (Accessed 3 October 2021).
Firouzi, S., Nikkhah, A., Aminpanah, H. (2018). Rice single cropping or ratooning agro-system: which one is more environment-friendly? Environ. Sci. pollut. Res. 25, 32246–32256. doi: 10.1007/s11356-018-3076-x
Fitzgerald, M. A., McCouch, S. R., Hall, R. D. (2009). Not just a grain of rice: the quest for quality. Trends Plant Sci. 14, 133–139. doi: 10.1016/j.tplants.2008.12.004
Grabherr, M. G., Haas, B. J., Yassour, M., Levin, J. Z., Thompson, D. A., Amit, I., et al. (2011). Trinity: reconstructing a full-length transcriptome without a genome from RNA-seq data. Nat. Biotechnol. 29, 644. doi: 10.1038/nbt.1883
Gu, F., Gong, B., Gilbert, R. G., Yu, W., Li, E., Li, C, et al. (2019). Relations between changes in starch molecular fine structure and in thermal properties during rice grain storage. Food Chem. 295, 484–492. doi: 10.1016/j.foodchem.2019.05.168
Hakata, M., Kuroda, M., Miyashita, T., Yamaguchi, T., Kojima, M., Sakakibara, H, et al. (2012). Suppression of α-amylase genes improves quality of rice grain ripened under high temperature. Plant Biotechnol. J. 10, 1110–1117. doi: 10.1111/j.1467-7652.2012.00741.x
Huang, J., Pan, Y., Chen, H., Zhang, Z., Fang, C., Shao, C, et al. (2020). Physiochemical mechanisms involved in the improvement of grain-filling, rice quality mediated by related enzyme activities in the ratoon cultivation system. Field Crops Res. 258, 107962. doi: 10.1016/j.fcr.2020.107962
Ishimaru, T., Matsuda, T., Ohsugi, R., Yamagishi, T. (2003). Morphological development of rice caryopses located at the different positions in a panicle from early to middle stage of grain filling. Funct. Plant Biol. 30, 1139–1111. doi: 10.1071/FP03122
Kaneko, K., Sasaki, M., Kuribayashi, N., Suzuki, H., Sasuga, Y., Shiraya, T, et al. (2016). Proteomic and glycomic characterization of rice chalky grains produced under moderate and high-temperature conditions in field system. Rice 9, 1–16. doi: 10.1186/s12284-016-0100-y
Ke, D., Sun, G., Wang, Z. (2007). Effects of superoxide radicals on ACC synthase activity in chilling-stressed etiolated mungbean seedlings. Plant Growth Regul. 51, 83–91. doi: 10.1007/s10725-006-9150-2
Kim, J., Shon, J., Lee, C. K., Yang, W., Yoon, Y., Yang, W. H., et al. (2011). Relationship between grain filling duration and leaf senescence of temperate rice under high temperature. Field Crops Res. 122, 207–213. doi: 10.1016/j.fcr.2011.03.014
Lawas, L. M. F., Shi, W., Yoshimoto, M., Hasegawa, T., Hincha, D. K., Zuther, E., et al. (2018). Combined drought and heat stress impact during flowering and grain filling in contrasting rice cultivars grown under field conditions. Field Crops Res. 229, 66–77. doi: 10.1016/j.fcr.2018.09.009
Li, Y., Fan, C., Xing, Y., Yun, P., Luo, L., Yan, B., et al. (2014). Chalk5 encodes a vacuolar h+-translocating pyrophosphatase influencing grain chalkiness in rice. Nat. Genet. 46, 398. doi: 10.1038/ng.2923
Li, Y. B., Peng, B. (2018). Determination of the total starch content of rice seeds. Bio-101, e1010161. doi: 10.21769/Bioprotoc.1010161
Lin, W. X. (2019). Developmental status and problems of rice ratooning. J. Integr. Agric. 18, 246–247. doi: 10.1016/S2095-3119(19)62568-2
Lin, W., Chen, H., Zhang, Z., Xu, Q., Tu, N., Fang, C., et al. (2015). Research and prospect on physio-ecological properties of ratoon rice yield formation and its key cultivation technology. Chin. J. Eco-Agric. 23, 392–401. doi: 10.13930/j.cnki.cjea.150246
Lin, F., Letuma, P., Li, Z., Lin, S., Rensing, C., Lin, W, et al. (2021). Rhizospheric pathogen proliferation and ROS production are associated with premature senescence of the osvha-a1 rice mutant. J. Exp. Bot. 72, 7247–7263. doi: 10.1093/jxb/erab338
Lin, F., Rensing, C., Pang, Z., Zou, J., Lin, S., Letuma, P., et al. (2022). Metabolomic analysis reveals differential metabolites and pathways involved in grain chalkiness improvement under rice ratooning. Field Crops Res. 283, 108521. doi: 10.1016/j.fcr.2022.108521
Lin, L., Zhang, L., Cai, X., Liu, Q., Zhang, C., Wei, C, et al. (2018). The relationship between enzyme hydrolysis and the components of rice starches with the same genetic background and amylopectin structure but different amylose contents. Food Hydrocolloids 84, 406–413. doi: 10.1016/j.foodhyd.2018.06.029
Lo, P. C., Li, H., Kitano, H., Matsuoka, M. (2016). Starch metabolism and grain chalkiness under high temperature stress. Natl. Sci. Rev. 3, 36. doi: 10.1093/nsr/nww036
Mao, C., Lu, S., Lv, B., Zhang, B., Shen, J., He, J., et al. (2017). A rice NAC transcription factor promotes leaf senescence via ABA biosynthesis. Plant Physiol. 174, 1747–1763. doi: 10.1104/pp.17.00542
Ministry of Agriculture of the PRC (2017). Determination of rice quality: NY/T 83–2017 (BeiJing, China: China Agricultural Press).
Mohapatra, P. K., Sarkar, R. K., Kuanar, S. R. (2009). Starch synthesizing enzymes and sink strength of grains of contrasting rice cultivars. Plant Sci. 176, 256–263. doi: 10.1016/j.plantsci.2008.11.001
Nakano, H., Tanaka, R., Wada, H., Okami, M., Nakagomi, K., Hakata, M. (2020). Breaking rice yield barrier with the ratooning method under changing climatic conditions: A paradigm shift in rice cropping systems in southwestern Japan. Agron. J. 112, 3975–3992. doi: 10.1002/agj2.20309
Nonhebel, H. M., Griffin, K. (2020). Production and roles of IAA and ABA during development of superior and inferior rice grains. Funct. Plant Biol. 47, 716–726. doi: 10.1071/FP19291
Peng, Y., Mao, B., Zhang, C., Shao, Y., Wu, T., Hu, L., et al. (2021). Influence of physicochemical properties and starch fine structure on the eating quality of hybrid rice with similar apparent amylose content. Food Chem. 353, 129461. doi: 10.1016/j.foodchem.2021.129461
Peng, T., Sun, H., Qiao, M., Zhao, Y., Du, Y., Zhang, J., et al. (2014). Differentially expressed microRNA cohorts in seed development may contribute to poor grain filling of inferior spikelets in rice. BMC Plant Biol. 14, 1–17. doi: 10.1186/s12870-014-0196-4
Plucknett, D. L., Evenson, J. P., Sanford, W. G. (1970). Ratoon cropping. Adv. Agron. 22, 285–330. doi: 10.1016/S0065-2113(08)60271-0
Prathap, V., Ali, K., Singh, A., Vishwakarma, C., Krishnan, V., Chinnusamy, V., et al. (2019). Starch accumulation in rice grains subjected to drought during grain filling stage. Plant Physiol. Biochem. 142, 440–451. doi: 10.1016/j.plaphy.2019.07.027
Ren, Y., Huang, Z., Jiang, H., Wang, Z., Wu, F., Xiong, Y., et al. (2021). A heat stress responsive NAC transcription factor heterodimer plays key roles in rice grain filling. J. Exp. Bot. 72, 2947–2964. doi: 10.1093/jxb/erab027
Sakuraba, Y., Kim, D., Han, S. H., Lü, B., Liang, J. (2020). Multilayered regulation of membrane-bound ONAC054 is essential for abscisic acid-induced leaf senescence in rice. Plant Cell 32, 630–649. doi: 10.1105/tpc.19.00569
Schirmer, M., Höchstötter, A., Jekle, M., Arendt, E., Becker, T. (2013). Physicochemical and morphological characterization of different starches with variable amylose/amylopectin ratio. Food Hydrocolloids 32, 52–63. doi: 10.1016/j.foodhyd.2012.11.032
Sharma, K. P., Sharma, N. (2018). Influence of high temperature on sucrose metabolism in chalky and translucent rice genotypes. Proc. Natl. Acad. Sci. India Sect. B.: Biol. Sci. 88, 1275–1284. doi: 10.1007/s40011-017-0865-9
Shen, X., Zhang, L., Zhang, J. (2021). Ratoon rice production in central China: Environmental sustainability and food production. Sci. Total Environ. 764, 142850. doi: 10.1016/j.scitotenv.2020.142850
Shimoyanagi, R., Abo, M., Shiotsu, F. (2021). Higher temperatures during grain filling affect grain chalkiness and rice nutrient contents. Agronomy 11, 1360. doi: 10.3390/agronomy11071360
Suriyasak, C., Harano, K., Tanamachi, K., Matsuo, K., Tamada, A., Iwaya-Inoue, M., et al. (2017). Reactive oxygen species induced by heat stress during grain filling of rice (Oryza sativa l.) are involved in occurrence of grain chalkiness. J. Plant Physiol. 216, 52–57. doi: 10.1016/j.jplph.2017.05.015
Tang, T., Xie, H., Wang, Y., Lü, B., Liang, J. (2009). The effect of sucrose and abscisic acid interaction on sucrose synthase and its relationship to grain filling of rice (Oryza sativa l.). J. Exp. Bot. 60, 2641–2652. doi: 10.1093/jxb/erp114
Teng, Z., Chen, Y., Yuan, Y., Peng, Y., Yi, Y., Yu, H., et al. (2022). Identification of microRNAs regulating grain filling of rice inferior spikelets in response to moderatiosoil drying post-anthesis. Crop J 10(4):962–71.
Wang, Y. C., Li, X. F., Lee, T., Peng, S. B., Dou, F. G. (2021). Effects of nitrogen management on the ratoon crop yield and head rice yield in south USA. J. Integr. Agric. 20, 1457–1464. doi: 10.1016/S2095-3119(20)63452-9
Wang, G., Li, H., Wang, K., Yang, J., Duan, M., Zhang, J., et al. (2020). Regulation of gene expression involved in the remobilization of rice straw carbon reserves results from moderatiosoil drying during grain filling. Plant J. 101, 6034. doi: 10.1111/tpj.14565
Wang, E., Wang, J., Zhu, X., Hao, W., Wang, L., Li, Q., et al. (2008). Control of rice grain-filling and yield by a gene with a potential signature of domestication. Nat. Genet. 40, 1370–1374. doi: 10.1038/ng.220
Wang, Z., Xu, Y., Chen, T., Zhang, H., Yang, J., Zhang, J., et al. (2015). Abscisic acid and the key enzymes and genes in sucrose-to-starch conversion in rice spikelets in response to soil drying during grain filling. Planta 241, 1091–1107. doi: 10.1007/s00425-015-2245-0
Wang, G., Zhang, J. (2020). Carbohydrate, hormone and enzyme regulations of rice grain filling under post-anthesis soil drying. Environ. Exp. Bot. 178, 104165. doi: 10.1016/j.envexpbot.2020.104165
Wang, N., Zhao, M., Li, Q., Liu, X., Song, H., Peng, X., et al. (2020). Effects of defoliation modalities on plant growth, leaf traits, and carbohydrate allocation in amorpha fruticosa l. and robinia pseudoacacia l. seedlings. Ann. For. Sci. 77, 1–15. doi: 10.1007/s13595-020-00953-1
Wani, A. A., Singh, P., Shah, M. A., Schweiggert‐Weisz, U., Gul, K., Wani, I. A., et al. (2012). Rice starch diversity: Effects on structural, morphological, thermal, and physicochemical properties–a review. Compr. Rev. Food Sci. Food Saf. 11, 417–436. doi: 10.1111/j.1541-4337.2012.00193.x
Wu, G. S. (2005). Performance and high-yielding cultivation techniques of ratooning rice from II youhang 1 in wuyi mountain region. Fujian Sci. Technol. Rice Wheat 23, 45–46.
Wu, C. Y., Trieu, A., Radhakrishnan, P., Kwok, S. F., Harris, S., Zhang, K., et al. (2008). Brassinosteroids regulate grain filling in rice. Plant Cell 20, 2130–2145. doi: 10.1105/tpc.107.055087
Xing, Y., Zhang, Q. (2010). Genetic and molecular bases of rice yield. Annu. Rev. Plant Biol. 61, 421–442. doi: 10.1146/annurev-arplant-042809-112209
Xu, Y., Liang, L., Wang, B., Xiang, J., Gao, M., Fu, Z., et al. (2022). Conversion from double-season rice to ratoon rice paddy fields reduces carbon footprint and enhances net ecosystem economic benefit. Sci. Total Environ. 813, 152550. doi: 10.1016/j.scitotenv.2021.152550
Xu, F., Zhang, L., Zhou, X., Guo, X., Zhu, Y., Liu, M., et al. (2021). The ratoon rice system with high yield and high efficiency in China: Progress, trend of theory and technology. Field Crops Res. 272, 108282. doi: 10.1016/j.fcr.2021.108282
Yang, J., Peng, S., Visperas, R., Sanico, A. L., Zhu, Q., Gu, S., et al. (2000). Grain filling pattern and cytokinin content in the grains and roots of rice plants. Plant Growth Regul. 30, 261–270. doi: 10.1023/A:1006356125418
Yang, J., Zhang, J. (2006). Grain filling of cereals under soil drying. New Phytol. 169, 223–236. doi: 10.1111/j.1469-8137.2005.01597.x
Yang, J., Zhang, J. (2010). Grain-filling problem in ‘super’rice. J. Exp. Bot. 61, 1–5. doi: 10.1093/jxb/erp348
Yang, J., Zhang, J., Liu, K., Wang, Z., Liu, L. (2006). Abscisic acid and ethylene interact in wheat grains in response to soil drying during grain filling. New Phytol. 171, 293–303. doi: 10.1111/j.1469-8137.2006.01753.x
Yang, J., Zhang, J., Wang, Z., Zhu, Q., Liu, L. (2001a). Water deficit–induced senescence and its relationship to the remobilization of pre-stored carbon in wheat during grain filling. Agron. J. 93 (1), 196–206. doi: 10.2134/agronj2001.931196x
Yang, J., Zhang, J., Wang, Z., Zhu, Q., Wang, W. (2001b). Remobilization of carbon reserves in response to water deficit during grain filling of rice. Field Crops Res. 71 (1), 47–55. doi: 10.1016/S0378-4290(01)00147-2
Yang, J., Zhang, J., Wang, Z., Zhu, Q., Liu, L. (2002). Abscisic acid and cytokinins in the root exudates and leaves and their relationship to senescence and remobilization of carbon reserves in rice subjected to water stress during grain filling. Planta 215, 645–652. doi: 10.1007/s00425-002-0789-2
Yang, J., Zhang, J., Wang, Z., Zhu, Q., Liu, L. (2003). Activities of enzymes involved in sucrose-to-starch metabolism in rice grains subjected to water stress during filling. Field Crops Res. 81, 69–81. doi: 10.1016/S0378-4290(02)00214-9
Yang, J., Zhang, J., Wang, Z., Zhu, Q., Liu, L. (2004). Activities of fructan-and sucrose-metabolizing enzymes in wheat stems subjected to water stress during grain filling. Planta 220, 331–343. doi: 10.1007/s00425-004-1338-y
Yao, D., Wu, J., Luo, Q., Li, J., Zhuang, W., Xiao, G., et al. (2020). Influence of high natural field temperature during grain filling stage on the morphological structure and physicochemical properties of rice (Oryza sativa l.) starch. Food Chem. 310, 125817. doi: 10.1016/j.foodchem.2019.125817
Ying, J., Peng, S., He, Q., Yang, H., Yang, C., Visperas, R. M., et al. (1998). Comparison of high-yield rice in tropical and subtropical environments: I. Determinants grain dry matter yields Field Crops Res. 57, 71–84. doi: 10.1016/S0378-4290(98)00077-X
Yuan, S., Cassman, K. G., Huang, J., Peng, S., Grassini, P. (2019). Can ratoon cropping improve resource use efficiencies and profitability of rice in central China? Field Crops Res. 234, 66–72. doi: 10.1016/j.fcr.2019.02.004
Yu, X., Yuan, S., Tao, X., Huang, J., Yang, G., Deng, Z., et al. (2021). Comparisons between main and ratoon crops in resource use efficiencies, environmental impacts, and economic profits of rice ratooning system in central China. Sci. Total Environ. 799, 149246. doi: 10.1016/j.scitotenv.2021.149246
Zhang, Z., Chen, J., Li, S., Li, Z., Cheng, R., Fang, C., et al. (2012). Proteomic and phosphoproteomic determination of ABA's effects on grain-filling of oryza sativa l. inferior spikelets. Plant Sci. 185, 259–273. doi: 10.1016/j.plantsci.2011.11.012
Zhang, H., Jing, W., Zhao, B., Wang, W., Xu, Y., Zhang, W., et al. (2021). Alternative fertilizer and irrigation practices improve rice yield and resource use efficiency by regulating source-sink relationships. Field Crops Res. 265, 108124. doi: 10.1016/j.fcr.2021.108124
Zhang, H., Li, H. W., Yuan, L. M., Wang, Z., Yang, J., Zhang, J., et al. (2012). Post-anthesis alternate wetting and moderate soil drying enhances activities of key enzymes in sucrose-to-starch conversion in inferior spikelets of rice. J. Exp. Bot. 63, 215–227. doi: 10.1093/jxb/err263
Zhang, X., Pan, H., Cao, J., Li, J. (2015a). Energy consumption of china’s crop production system and the related emissions. Renewable Sustain. Energy Rev. 43, 111–125. doi: 10.1016/j.rser.2014.11.034
Zhang, Z. X., Tang, J., Du, T. W., Zhao, H., Li, Z., Li, Z., et al. (2015b). Mechanism of developmental stagnancy of rice inferior spikelets at early grain-filling stage as revealed by proteomic analysis. Plant Mol. Biol. Rep. 33, 1844–1863. doi: 10.1007/s11105-015-0880-z
Zhang, Z. X., Zhang, Y. P., Zhao, H., Huang, F., Zhang, Z., Lin, W., et al. (2017). The important functionality of 14-3-3 isoforms in rice roots revealed by affinity chromatography. J. Proteomics 158, 20–30. doi: 10.1016/j.jprot.2017.02.008
Zhang, Z., Zhao, H., Huang, F., Long, J., Song, G., Lin, W., et al. (2019). The 14-3-3 protein GF14f negatively affects grain filling of inferior spikelets of rice (Oryza sativa l.). Plant J. 99, 344–358. doi: 10.1111/tpj.14329
Zhang, Z. X., Zhao, H., Tang, J., Li, Z., Li, Z., Chen, D., et al. (2014). A proteomic study on molecular mechanism of poor grain-filling of rice (Oryza sativa l.) inferior spikelets. PloS One 9, e89140. doi: 10.1371/journal.pone.0089140
Zhou, W. X. (2006). Comparative studies on growth characters and source-sink relationships of different types of ratooning rice. (Hunan, China: PhD Dissertation of Hunan Agricultural University in Chinese with English abstract).
Zhou, L. (2020). Study on physio-ecological mechanism of yield formation of ratooning rice generated from low stubbles of main crop. (Fuzhou, China: PhD Dissertation of Hunan Agricultural University in Chinese with English abstract).
Zhuang, J., Sun, H., Sayler, G., Kline, K. L., Dale, V. H., Jin, M., et al. (2021). Food–energy–water crises in the united states and China: commonalities and asynchronous experiences support integration of global efforts. Environ. Sci. Technol. 55, 1446–1455. doi: 10.1021/acs.est.0c06607
Keywords: ratooning season rice, GF14f gene, carbon reserve remobilization, starch composition, oxidative and environmental resistance
Citation: Lin F, Lin S, Zhang Z, Lin W, Rensing C and Xie D (2023) GF14f gene is negatively associated with yield and grain chalkiness under rice ratooning. Front. Plant Sci. 14:1112146. doi: 10.3389/fpls.2023.1112146
Received: 30 November 2022; Accepted: 23 January 2023;
Published: 10 February 2023.
Edited by:
Prashant Vikram, Shriram Bioseed Genetics, IndiaReviewed by:
Kurniawan Rudi Trijatmiko, International Rice Research Institute (IRRI), PhilippinesYifeng Wang, China National Rice Research Institute (CAAS), China
Karthikeyan Thiyagarajan, International Maize and Wheat Improvement Center, Mexico
Copyright © 2023 Lin, Lin, Zhang, Lin, Rensing and Xie. This is an open-access article distributed under the terms of the Creative Commons Attribution License (CC BY). The use, distribution or reproduction in other forums is permitted, provided the original author(s) and the copyright owner(s) are credited and that the original publication in this journal is cited, in accordance with accepted academic practice. No use, distribution or reproduction is permitted which does not comply with these terms.
*Correspondence: Daoxin Xie, ZGFveGlubGFiQG1haWwudHNpbmdodWEuZWR1LmNu; Christopher Rensing, cmVuc2luZ0BpdWUuYWMuY24=; Wenxiong Lin, bHd4QGZhZnUuZWR1LmNu