- Institute of Food and Agricultural Technology-CIDSAV-XaRTA, University of Girona, Girona, Spain
The activity of Eucalyptus essential oil against eleven strains pertaining to six species of plant pathogenic bacteria was studied using growth inhibition and contact assays. All strains were susceptible to the formulation EGL2, and Xylella fastidiosa subspecies and Xanthomonas fragariae were the most sensitive. The bactericidal effect was strong causing 4.5 to 6.0 log reductions in survival in 30 min at concentrations in the range of 0.75 to 15.0 μl/ml depending on the bacteria tested. Transmission electron microscopy of the formulation EGL2 against the three X. fastidiosa subspecies studied allowed the observation of a strong lytic effect on bacterial cells. In addition, the preventive spray application of EGL2 to potted pear plants subsequently inoculated with Erwinia amylovora significantly decreased the severity of infections. Almond plants treated by endotherapy or soil drenching, and then inoculated with X. fastidiosa showed a significant decrease in disease severity as well as in the levels of the pathogen, depending on the strategy used (endotherapy/soil drenching, preventive/curative). The treatment by endotherapy in almond plants induced the expression of several genes involved in plant defense. It was concluded that the reduction of infections by the Eucalyptus oil treatments was due to the combination of its bactericidal and plant defense induction activities.
1 Introduction
Worldwide losses in crop yields due to diseases, and their implications for global food demands, create the need for improving disease management. IPPC Secretariat (2021a) estimated annual loses up to 40% of global crop production caused by pests, where plant diseases represent a global economy cost over $220 billion, and international standards for phytosanitary measures include protecting sustainable agriculture and improving global food security, as well as protecting the environment (IPPC Secretariat, 2021b). European Commission aim to reduce by 50% the currently homologated chemical pesticides by 2030 (EC, Plant protection in EU agriculture 2022), but in fact, control of most bacterial diseases is only reasonably accomplished by using copper compounds. However, these compounds are not sufficiently effective in most devastating diseases such as those caused by Pseudomonas syringae pv. syringae (bacterial canker of pear), P. syringae pv. actinidiae (bacterial canker of kiwi fruit), Xanthomonas arboricola pv. pruni (bacterial spot of almond) or Erwinia amylovora (fire blight of pear, apple) (Iacobellis et al., 2005; Vanneste et al., 2008; Palacio-Bielsa et al., 2010).
Even more difficult is managing diseases caused by the fastidious phytopathogenic bacteria Xylella fastidiosa or Candidatus Liberibacter (Blaustein et al., 2019; Moll et al., 2022). X. fastidiosa is responsible for emerging diseases in Europe affecting mainly olive and almond, and there are still no effective methods to cure infected plants, due to the lack of effective bactericides and the difficulty to access to the vascular system, where the pathogen establishes. This pathogen is exclusively transmitted by xylem fluid-feeding insects, by grafting or budding and can infect more than 600 plant species (EFSA, 2022). This wide spectrum of hosts combined with genetic plasticity, favors the spread of the pathogen, and make its control extremely difficult (Baldi and La Porta, 2017; Denancé et al., 2019; EFSA, 2021). Since the first detection of X. fastidiosa subsp. pauca in olive plants in Apulia region, Italy in 2013, about 5 million trees has been infected or dead, representing losses of about 10% of Italian olive oil (White et al., 2020). In Spain, X. fastidiosa was first detected in Balearic Islands in 2016 and to date, more than 80% of almond trees are affected by almond leaf scorch disease (ALS) (Morelli et al., 2021; Olmo et al., 2021). Similarly, in 2017, X. fastidiosa subsp. multiplex was detected in diseased almond trees in the Valencian Community (Spain), and in 2021, the infected area reached 2292 ha, and more than 100,000 diseased almond trees have been destroyed (Morelli et al., 2021). Several strategies have been assessed to control X. fastidiosa diseases on plant hosts, including chemical control using oxytetracycline or Zn/Cu citric biocomplex foliar treatments (Amanifar et al., 2016; Dongiovanni et al., 2017; Scortichini et al., 2018; Bruno et al., 2021), stimulators of plant defense responses (Zhang et al., 2019; Moll et al., 2022), biological control using antagonistic endophytes (Baccari et al., 2019) or different agricultural practices. Some of these treatments can reduce symptoms, and in some few cases, even decrease the population levels in infected plants or trees. However, the main concern is that no cure has been found to be effective in the control of X. fastidiosa in infected trees.
Thus, there is a strong need for new products for bacterial disease control, and specially for the emerging and re-emerging diseases of tree crops (Sundin et al., 2016; Sharma et al., 2022). In recent years, the development of biopesticides and particularly non-microbial biopesticides based on natural products are experiencing an increasing growth, as they fulfill the regulations associated to plant protection, such as sustainability, biodegradability and minimal toxicity to humans and environment (Leahy et al., 2014). Many plant extracts or essential oils (EOs) appear as alternative control strategies, since their efficacy against a wide range of pathogens and pests has been confirmed in vitro and to a lesser extent, in planta (Raveau et al., 2020). Particularly, different studies reported in vitro antibacterial activity of EO such as EO from Satureja hortensis L., Cleistocalyx peraculatus or Eucalyptus globulus against E. amylovora, Xanthomonas campestris pv. vesicatoria or X. fastidiosa (Bajpai et al., 2010; Karami-Osboo et al., 2010; Brentini et al., 2018).
In addition, it is important to point out that the regulatory process for registration of bioactive natural products as pesticides may be faster than for conventional chemical products (Mohan et al., 2011).
Different EO-based products have been developed and screened for their fungicidal, herbicidal, and insecticidal activity or as growth regulators, and an increasing number of these products have been homologated for their use in agriculture and commercialized (Raveau et al., 2020; Pathma et al., 2021). Among commercially available botanical derived-biopesticides, there are some described as antimicrobial agents and/or inducers of plant defense, and are effective in controlling bacterial diseases in several crops such as tomato or wheat. In this context, tea tree oil has been reported to exhibit antifungal and antibacterial properties, inhibiting powdery mildew of barley (Terzi et al., 2007). Also, a salicylic acid (SA) analog or ethanolic extracts of Giant Knotweed can induce resistance in a wide range of hosts (Ramasamy et al., 2015; Margaritopoulou et al., 2020). However, there is a lack of EO-products against plant pathogenic bacteria and bacterial diseases. Eucalyptus EO was shown to have antibacterial activity (Tan et al., 2008; Bachir and Benali, 2012), and presents a low risk profile, as it is commercially available for human health and as flavoring agents in food (Batish et al., 2008). Interestingly, neither Eucalyptus EO nor active substance derived from it are approved for use as biopesticides, neither in Europe nor in the United States (https://food.ec.europa.eu; https://epa.gov) .
The aim of the present work was to evaluate the effect of a Eucalyptus EO (EGL2) in controlling X. fastidiosa infections in young potted almond plants as well as E. amylovora infections in pear plants. More specifically the objectives were to: (1) evaluate the antimicrobial activity of EGL2 against different plant pathogenic bacteria of economic importance, (2) assess the effect of spray treatment with EGL2 in controlling E. amylovora infections, (3) study the effect of different treatments with EGL2 in the reduction of ALS symptoms and levels of X. fastidiosa in almond plants, and (4), evaluate the effect of EGL2 treatment in the induction of defense responses in almond plants.
2 Materials and methods
2.1 Bacterial strains and growth conditions
The bacterial pathogens and growth conditions used in the study are listed in Supplementary Table 1. Bacterial strains were cultured at 28°C for 24 h-48 h (for X. fastidiosa strains, 7-10 days) and scrapped from surface to prepare suspensions adjusted to 108 CFU/ml.
2.2 Eucalyptus oil formulation
EGL2 Eucalyptus EO was obtained from leaves and branches of Eucalyptus globulus plants at harvest time in winter, from October to February. Qualitative and quantitative analyses were performed using gas chromatography coupled with a flame ionization detector (GC-FID). The main components identified were 1,8-cineole (>70), limonene (<10%) and α-Terpineol (<10%) (Supplementary Table 2). Dilutions of the product were made in double distilled water to obtain the desired final concentrations.
2.3 Antimicrobial activity
Agar incorporation test method was used for growth inhibition assays for EGL2 product. Briefly, the required agar growth medium was mixed with the corresponding product concentration (5, 10, 30, 60 and 120 µl/ml), and the 10 µl of the test plant pathogenic bacteria (at final concentration of 108 CFU/ml) was added at the center of the agar plate. Three replicates for product concentration were used. Positive controls containing water instead of product, and negative controls containing a bactericidal reference product were included (streptomycin and ampicillin at 100 mg/l). Plates were incubated at 28°C for 24-48 h or 5-10 days, depending on the plant pathogenic bacteria (Supplementary Table 1). Microbial growth was determined qualitatively, by determining the presence or absence of bacterial growth. The minimal inhibitory concentration (MIC) value was taken as the lowest product concentration with no growth at the end of the experiment.
2.4 Bactericidal activity
Bactericidal activity of EGL2 was determined by a contact test or killing assay, consisting of the exposure of the target microorganism (selected from) to an antimicrobial compound for a given time and determining the surviving cells (Montesinos et al., 2021). 100 µl of the corresponding product concentration were mixed in a microtiter plate with 100 µl of bacterial suspension (at final concentration of 5x107 CFU/ml), to a total volume of 200 µl. Three replicates for each concentration and pathogen were used. Controls containing water instead of EGL2 (negative control) or a bactericidal reference product (positive control) were included. Microplates were incubated at 28°C for 30 and 120 minutes under constant shaking. The plate counting method was used to quantify the culturable cells and to assess the bactericidal activity of EGL2. Decimal dilutions of each EGL2 concentration were prepared and plated (20 µl) onto the surface of agar plates and colony forming units (CFU) were quantified at 24-48 h or 5-10 days after the incubation at 28°C.
2.5 Transmission electron microscopy
Alterations in bacterial membrane morphology and integrity of X. fastidiosa subsp. fastidiosa, X. fastidiosa subsp. multiplex and X. fastidiosa subsp. pauca cells after EGL2 treatment was observed by transmission electron microscope (TEM). Cells were exposed to EGL2 (6 µl/ml) for 120 min and harvest by centrifugation at 10,000 g for 10 min. The Microscopy Unit (Research Technical Services) of the University of Girona fixed pelleted cells, included in epoxy resin, and prepared the ultra-thin sections of 60 to 80 nm as described in Baró et al., 2020a. After contrasting sections with uranium acetate 2%, the samples were observed with a JEOL JEEM1400 transmission electron microscope at the Microscopy Unit of the Autonomous University of Barcelona.
2.6 Effect of EGL2 treatment on bacterial infections in plants
The efficacy of EGL2 in controlling infections by E. amylovora and X. fastidiosa was evaluated in potted pear and almond plants, respectively, and under greenhouse conditions.
2.6.1 Erwinia amylovora assays
Self-rooted pear plants cv. Conference (3-year-old) were used. Plants were pruned to leave 3-4 shoots per plant, forced to sprout in the greenhouse and used when shoots contained 5 to 6 young leaves per shoot. Plants were fertilized once a week with a 200 ppm of water soluble NPK solution (20:10:20). Disease was evaluated in leaves of plants that have been sprayed until drop-off with aqueous solution of EGL2 at 20 and 40 µl/ml (10 ml per plant). Streptomycin (0.10 mg/ml) was used as a reference control product, and water-sprayed plants were used as non-treated controls (NTC). Before treating the plants with different EGL2 doses, a double transverse incision (ca. 1mm) was made perpendicular to the midrib of the three youngest fully expanded leaves (most susceptible to infection) of each shoot. After 24 h, treated plants were inoculated with the pathogen by delivering 10 µl of bacterial suspension (at 5x107 CFU/ml) to the center of the two incisions previously made in the midrib. Plants were incubated in the controlled environment greenhouse at 23 ± 2 °C and a photoperiod of for 16 h of light and 8 h dark and 60% relative humidity. The experimental design consisted of three biological replicates of three plants per each treatment and pathogen. Two independent experiments were performed.
After incubation, disease symptoms were allowing to develop and the intensity of the infections was scored 8 days after pathogen inoculation, using a severity index ranging from 0 to a maximum of 4 (0, no symptoms; 1, localized necrosis around the wound; 2: complete necrosis of the midrib; 3, progression of the necrosis across the petiole and 4, progression of necrosis towards shoot). In every plant, each of 3 leaves belonging to each shoot was rated according to the index, and it was used to calculate a disease severity index per plant according to the formula:
where S is the severity of the infections per plant, Ii is the severity index for each leaf, n is the number of leaves measured, which is multiplied by the maximum severity index (i.e. 4). Then, the mean of the three plants for each biological replicate was used for the statistical analysis.
2.6.2 Xylella fastidiosa assays
The effect of EGL2 on disease severity caused by X. fastidiosa subsp. fastidiosa 5387.2 and subsp. multiplex 5901.2 in inoculated almond plants was assessed. Almond plants were inoculated as detailed in Baró et al. (2021). Briefly, three inoculations of 10 µl each (30 µl of inoculum per plant, total inoculum of 3x106 CFU) were applied at the same side of the stem in a section of 3 cm at around 15 cm above the substrate level, with a high-precision microinjector (NanoJet; Chemyx, Stafford, TX) provided with a Hamilton 250-µl syringae with a thin needle with a beveled tip (Bonaduz, Switzerland).
In a first experiment, 20 or 40 µl/ml of EGL2 was applied preventively by endotherapy following the protocol described in Baró et al. (2021) for pathogen inoculation. In a second experiment, four independent strategies of 60 µl/ml EGL2 treatment were explored and consisted of (1) preventive application by endotherapy 1 day before the inoculation (1dbi) of X. fastidiosa (2) combination of preventive (1 dbi) and curative application by endotherapy 7- and 43-days post-inoculation (dpi) (3) preventive application (1 dbi) by soil drench, and (4) combination of preventive (1 dbi) and curative application by soil drench 7 and 43 dpi.
At the end of the period of treatment the plants that have been treated by endotherapy using the preventive strategy received a total of 1.8 µl/plant, whereas the plants treated by endotherapy using the combined strategy (preventive and curative) received a total of 5.4 µl/plant (corresponding to three applications of 1.8 µl/plant, one preventive application and two additional curative applications). The experimental design consisted of nine plants per each treatment, and two independent experiments were performed. Symptoms were evaluated according to the severity scale previously described (Baró et al., 2021). For both pathosystems, data set was subjected to analysis of variance (one-way ANOVA) to determine if there were significant differences between treatments in bacterial disease control. Efficacy of each treatment was calculated based on the severity of the treatment in relation to severity observed in plants NTC group, according to the formula:
where E is the efficacy of the treatment (in percentage), SNTC is the severity observed in the plants of the NTC group and ST is the severity observed in the treatment group.
Infected plants were cultivated in a Biosafety level II+ quarantine greenhouse authorized by the Plant Health Services, according to EPPO recommended containment conditions (EPPO, 2006), taking into consideration X. fastidiosa quarantine status in the EU (EFSA PLH Panel et al., 2018).
2.7 Quantitative PCR
The levels of X. fastidiosa in inoculated plants were analyzed by quantitative PCR as described by Baró et al. (2021). To determine the movement and growth of X. fastidiosa, 16 cm of shoot material consisting of two sampling zones located above (Upwards zone 1, U1; Upwards zone 2, U2; 8 cm each zone) and below the inoculation point (Zone, D; 8 cm). X. fastidiosa levels were analyzed in each of nine plants per treatment and in each zone. Briefly, after removing the bark from each zone and processing the plant material, DNA extraction and purification was performed using the GeneJET Genomic DNA purification kit (Thermo Fisher Scientific, USA) following the manufacturer’s instructions. The number of total X. fastidiosa cells in the xylem tissue was quantified using a quantitative PCR and expressed as CFU/g, by interpolating the CT values of each sample in the standard curve, CT values vs. CFU, described previously (Baró et al., 2020b).
2.8 Transcriptomic analysis of almond plants challenged with EGL2
The effect of EGL2 in almond plant defense response was evaluated using two preventive application approaches, endotherapy or soil drenching, as detailed in section 2.6 (X. fastidiosa section, preventive application). Control plants were treated with distilled water. For RT-qPCR analyses, treated leaves were collected 24 h after treatment and immediately frozen in liquid nitrogen for subsequent RNA extraction. For total RNA extraction, the plant material was ground to a fine powder in liquid nitrogen with the Tissuelyzer II system (Qiagen) and total RNA was extracted using TriZol® (Invitrogen, Life Technologies) followed by DNAse treatment (Ambion® Turbo DNA-free™, Life Technologies) to remove any contaminant DNA, as described in Montesinos et al. (2021). RNA samples of 3 plants were pooled in the same Eppendorf tube, and three biological replicates per treatment were analyzed (9 plants/treatment, 3 tubes per treatment). cDNA was synthesized from RNA samples using High-Capacity cDNA Reverse Transcription Kits (Thermo Fisher Scientific Inc.) and was assayed for quantify the expression levels of eight Prunus genes related to plant defense and previously described in Ruiz et al. (2017); Tong et al. (2009); Foix et al. (2021). These genes codify a basic 7S globulin-like protein, glutamate receptor, a WRKY transcription factor, G-type lectin S-receptor-like serine/threonine-protein kinase, a pathogenesis-related transcriptional activator PTI5, a PR9 (a peroxidase 44), a RING-H2 finger protein and a pathogenesis-related protein 4 (PR4). Primers are detailed in Supplementary Table 3.
Quantitative Real Time-PCR was carried out in a fluorometric thermal cycler (qPCR Quant Studio 5, Applied Biosystems) using the Mix SYBR®Green PCR Master Mix (Applied Biosystems) as described in Badosa et al. (2017). The total reaction volume was 20 µl containing 1x Sybr Green Master Mix (Applied Biosystems), the appropriate concentration of primers (Sigma) and 2 µL of RT reaction (cDNA). The reaction conditions were as follows: (1) initial denaturation step (10 min at 95°C); (2) amplification and quantification (50 cycles of 15 s at 95°C and 1 min at 60°C); and a final melting program (60-95°C with a heating rate of 0.5°C/s) as described in Badosa and co-workers (2017). Reactions were carried out in duplicate in 96-well plates. Controls from no cDNA template were included as negative controls. The relative quantification of each individual gene expression was performed using the 2-ΔΔCt method (Livak and Schmittgen, 2001) and the REST2009 Software (Pfaffl et al., 2002). Relative expression values of each plant defense were calculated normalizing against the UBQ-gene as an internal control.
2.9 Data analysis
To test the effect of EGL2 on the population levels of X. fastidiosa on almond plants and disease severity in almond and pear plants, an analysis of variance (one-way ANOVA) was performed. Means were separated according to the Tukey’s test at a P value of ≤ 0.05. The statistical significance of the gene expression data was determined using the REST2009 Software (Pfaffl et al., 2002).
3 Results
3.1 Antibacterial activity
Antibacterial activity of EGL2 against the strains of plant pathogenic bacteria is shown in Table 1. All bacteria were susceptible to EGL2, but there was a differential susceptibility among strains, being the three subspecies of X. fastidiosa and Xanthomonas fragariae the most sensitive and E. amylovora the most tolerant. Specifically, EGL2 showed a MIC value of <5 µl/ml in X. fastidiosa and X. fragariae, and between 60 and 120 µl/ml in E. amylovora.
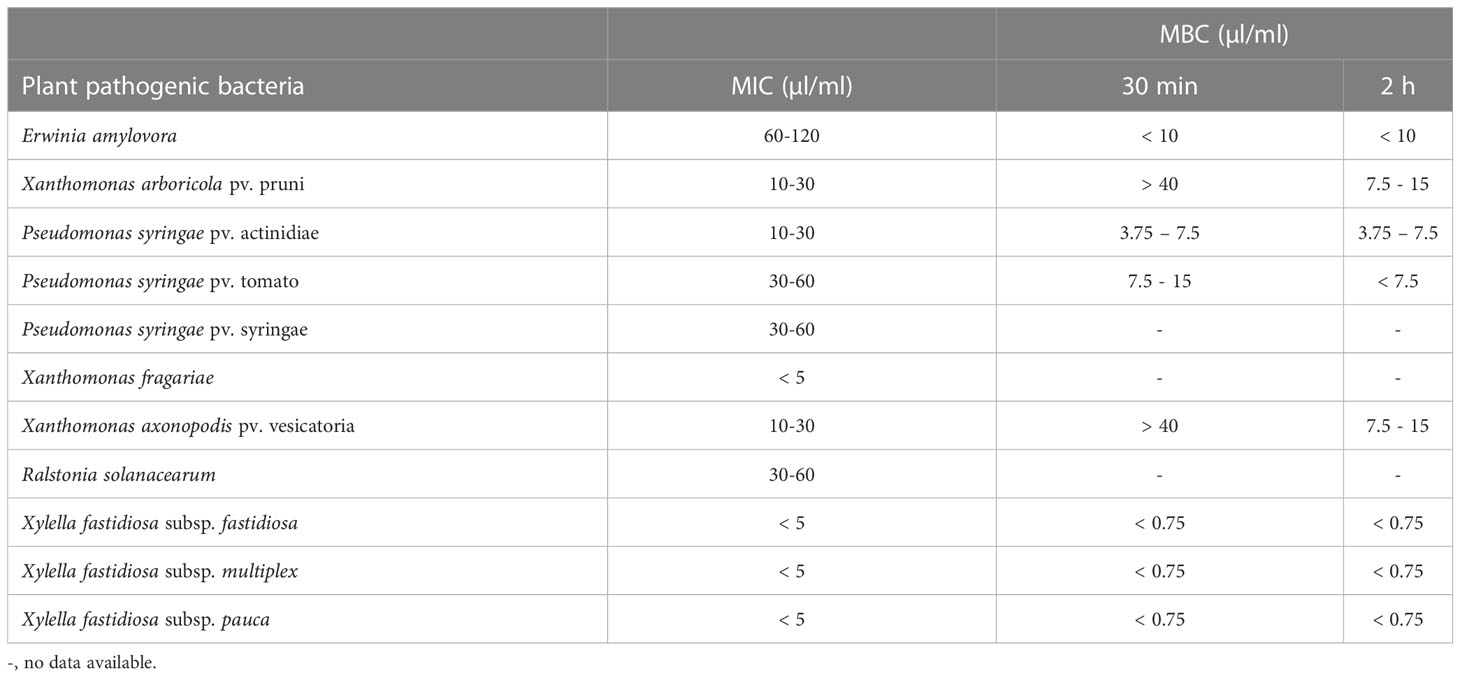
Table 1 Minimal inhibitory concentration (MIC) and bactericidal concentration (MBC) of Eucalyptus essential oil against plant pathogenic bacteria.
The bactericidal activity (killing assay) was determined against 8 plant pathogenic bacteria selected based on the MIC values (Figure 1). Reduction of bacterial populations (survival) varied according to the strain, EGL2 concentration and the exposure time. EGL2 exhibited rapid and potent bactericidal effect and, after 30 min of exposure, a maximum of > 5.7 to 6.0 log reduction (N0/N) in survival of E. amylovora, P. syringae pv. actinidiae, P. syringae pv. tomato, and X. campestris pv. vesicatoria was observed after incubation at 10, 7.5 and 15 µl/ml, respectively. EGL2 showed also bactericidal activity against X. arboricola pv. pruni, although we observed a lower survival reduction of 4.5 logs. Interestingly, a reduction of 5.6 to 6.4 logs in any of the three subspecies of X. fastidiosa was observed at 0.75 µl/ml. After increasing the exposure time to 120 min, the reduction of X. arboricola pv. pruni survival increased to 6.1 logs after incubation at 15 µl/ml. MBC values are detailed in Table 1.
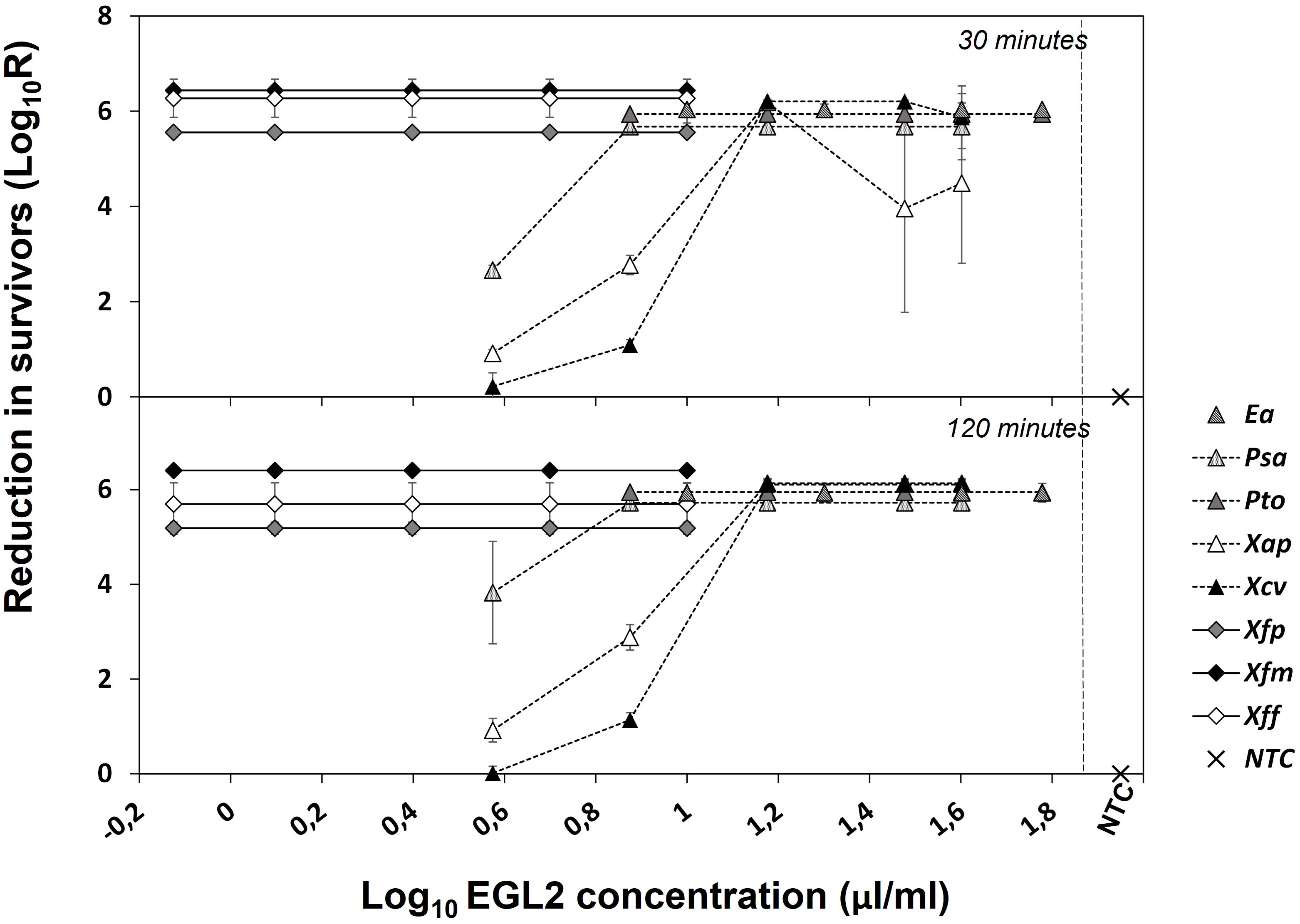
Figure 1 Effect of EGL2 in cell survival of different plant pathogenic bacteria after exposure to 30 or 120 minutes. Ea, Erwinia amylovora; Psa, Pseudomonas syringae pv. actinidiae; Pto, Pseudomonas syringae pv. tomato; Xap, Xanthomonas arboricola pv. pruni; Xcv, Xanthomonas campestris pv. vesicatoria; Xfp, Xylella fastidiosa subsp. pauca; Xfm, Xylella fastidiosa subsp. multiplex; Xff, Xylella fastidiosa subsp. fastidiosa. Non-treated controls (NTC) were included. Values are the means of three replicates, and error bars represent the standard deviation of the mean. Most bacteria were highly susceptible to EGL2 with high reduction in survivors even at the lowest concentration of 0.75 µl/ml. Psa, Xap and Xcv were the less susceptible.
3.2 Lytic effect of EGL2 on X. fastidiosa cells
TEM imaging revealed a potent lytic activity of EGL2 (at 6 µl/ml) against the three X. fastidiosa subspecies (Figure 2). The vast majority of X. fastidiosa cells lost the structural integrity and morphology of the membrane and the cell wall, compared to the non-treated control cells that maintained the cell wall integrity after 2 h exposure.
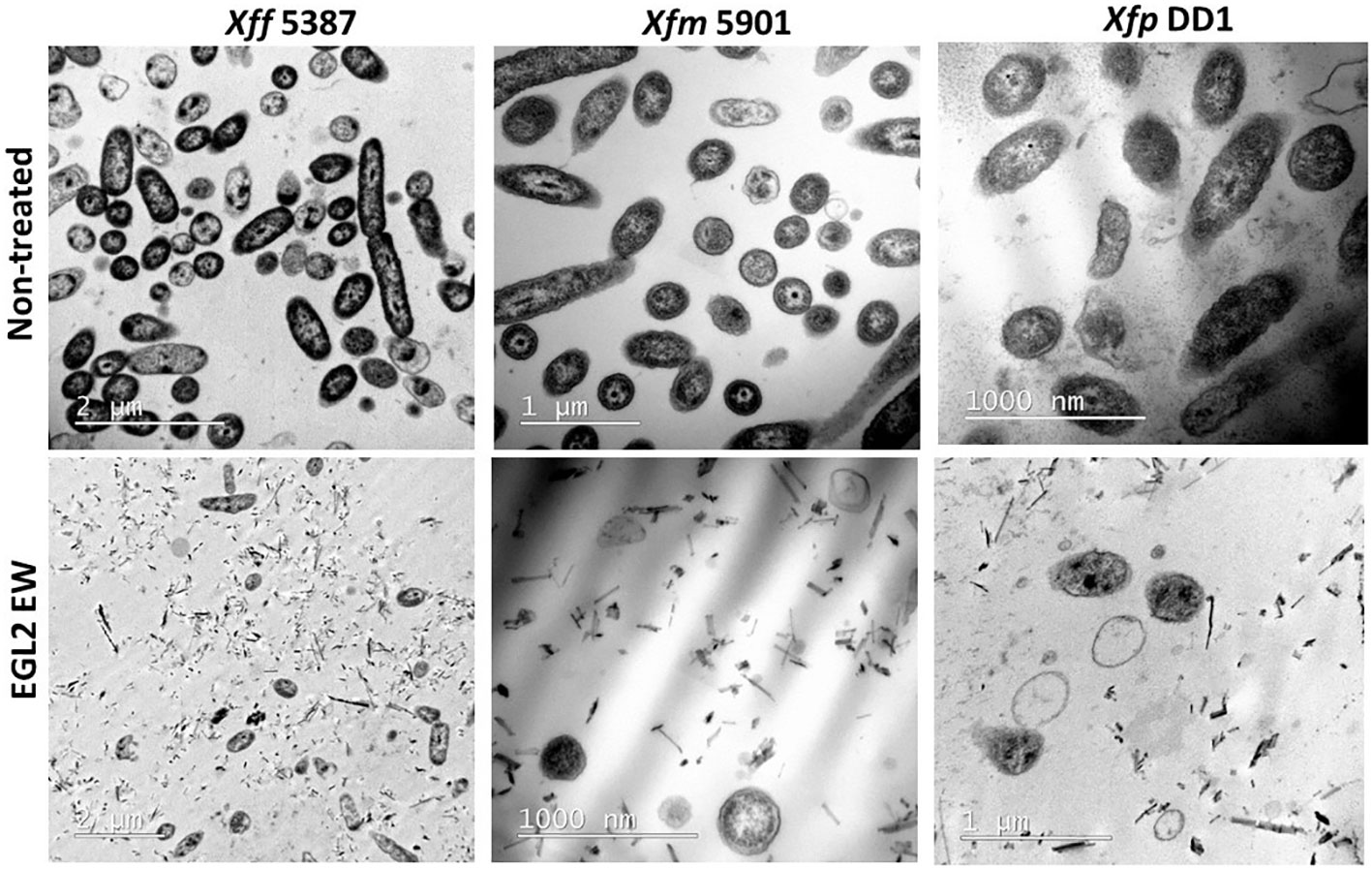
Figure 2 TEM microscopy of samples of of X. fastidiosa subsp. fastidiosa IVIA 5387.2, subsp. multiplex IVIA 5901.2 and subsp. pauca De Donno after exposure to EGL2 at 6 µl/ml for 120 min. Notice that very few cells remain intact after the treatment, and extensive lysis and debris material is observed (lower panels).
3.3 Effect of EGL2 treatment on bacterial infections in potted plants
3.3.1 Erwinia amylovora assays
The preventive spray of EGL2 on pear plants was effective in reducing severity of infections caused by E. amylovora (Figure 3). Although a significant difference between the two experiments conducted was observed (F=102.4; P<0.0001), the reduction effect was consistent (F=50.5; P<0.0001). More in detail, after treatment with 20 µl/ml, disease severity was 37.9% for experiment 1 and 50.6% for experiment 2 (37.0 and 36,7% efficacy, respectively), and after treatment with 40 µl/ml it was of 23.0% and 55.5% (39.4 and 30.2% efficacy), compared to non-treated controls (60.2% in experiment 1 and 79.5% in experiment 2). No significant differences were observed among EGL2 doses. In the second experiment no significant differences were observed between streptomycin and EGL2 treatment. No phytotoxic effects were observed on the treated pear plants.
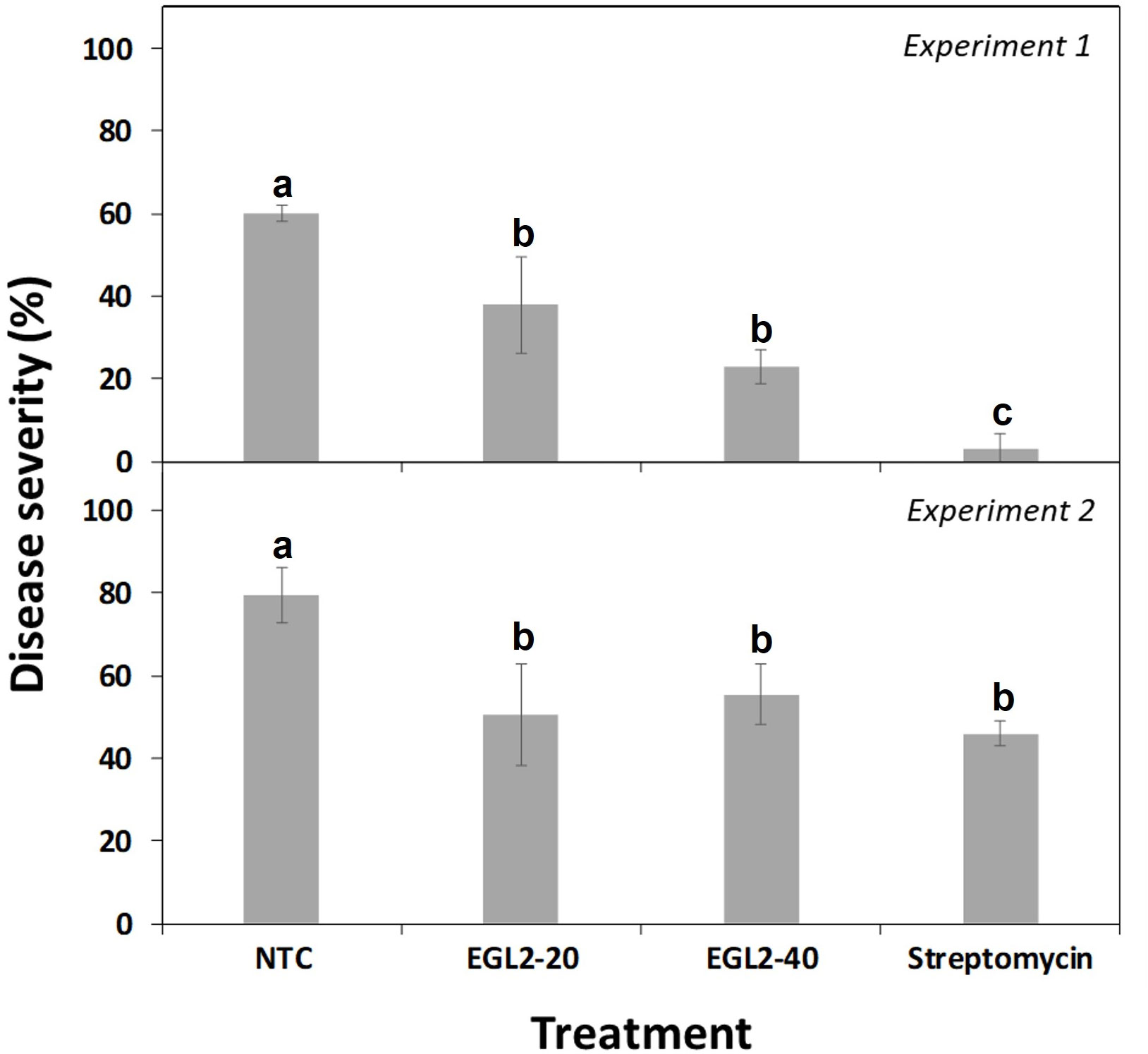
Figure 3 Effect of spray application of EGL2 on infection severity by E. amylovora on pear plant leaves. Two independent assays were performed, and EGL2 was applied by spraying 24 h before pathogen inoculation. Disease severity was evaluated on pear plants after 8 days from pathogen inoculation (107 CFU/ml). Values correspond to the mean disease severity of three replicates of three plants per treatment. Standard errors are indicated on bars. The effect of treatments was significant according to ANOVA (p<0.001 for experiment 1 and p=0.002 for experiment 2). Different letters between treatments indicate significant differences between disease severities according to Tukey’s test (P ≤ 0.05).
3.3.2 Xylella fastidiosa assays
Plants treated with water showed the typical symptoms of ALS disease, earlier than EGL2 treated plants. Consistently, EGL2 decreased the intensity of infections compared to NTC. In the first experiment (Figure 4), the preventive application of EGL2 by endotherapy controlled infections caused by both X. fastidiosa subsp. fastidiosa as well as X. fastidiosa subsp. multiplex in almond plants, with efficacies of 68.6% and 52%, respectively. In the second experiment (Figure 5), different application strategies were studied. EGL2 preventive application, either by endotherapy or soil drenching resulted in efficacies of 66.7%. The combined strategy (combination of preventive and curative application) using soil drenching or endotherapy allowed an efficacy of 63% and 48.1%, respectively.
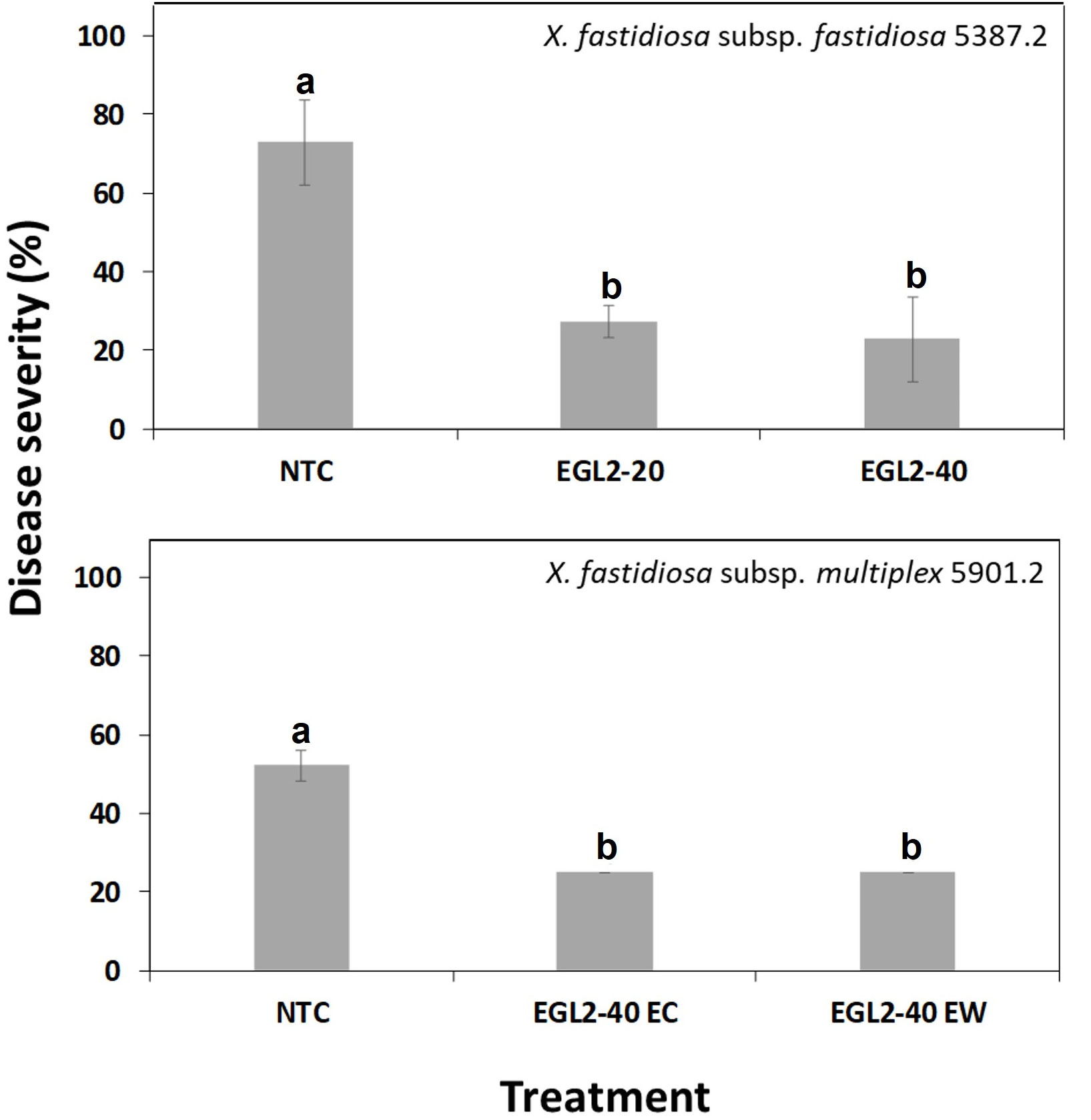
Figure 4 Disease severity of ALS in almond plants treated with EGL2 by endotherapy and infected by X. fastidiosa subsp. fastidiosa IVIA 5387.2 or X. fastidiosa subsp. multiplex IVIA 5901.2. Values are the means of 3 replicates of 3 plants, and error bars represent the standard deviation of the mean. The effect of treatments was significant according to ANOVA (p=0.006 for experiment 1 and p<0.001 for experiment 2). Different letters between treatments indicate significant differences between disease severities according to Tukey’s test (P ≤ 0.05).
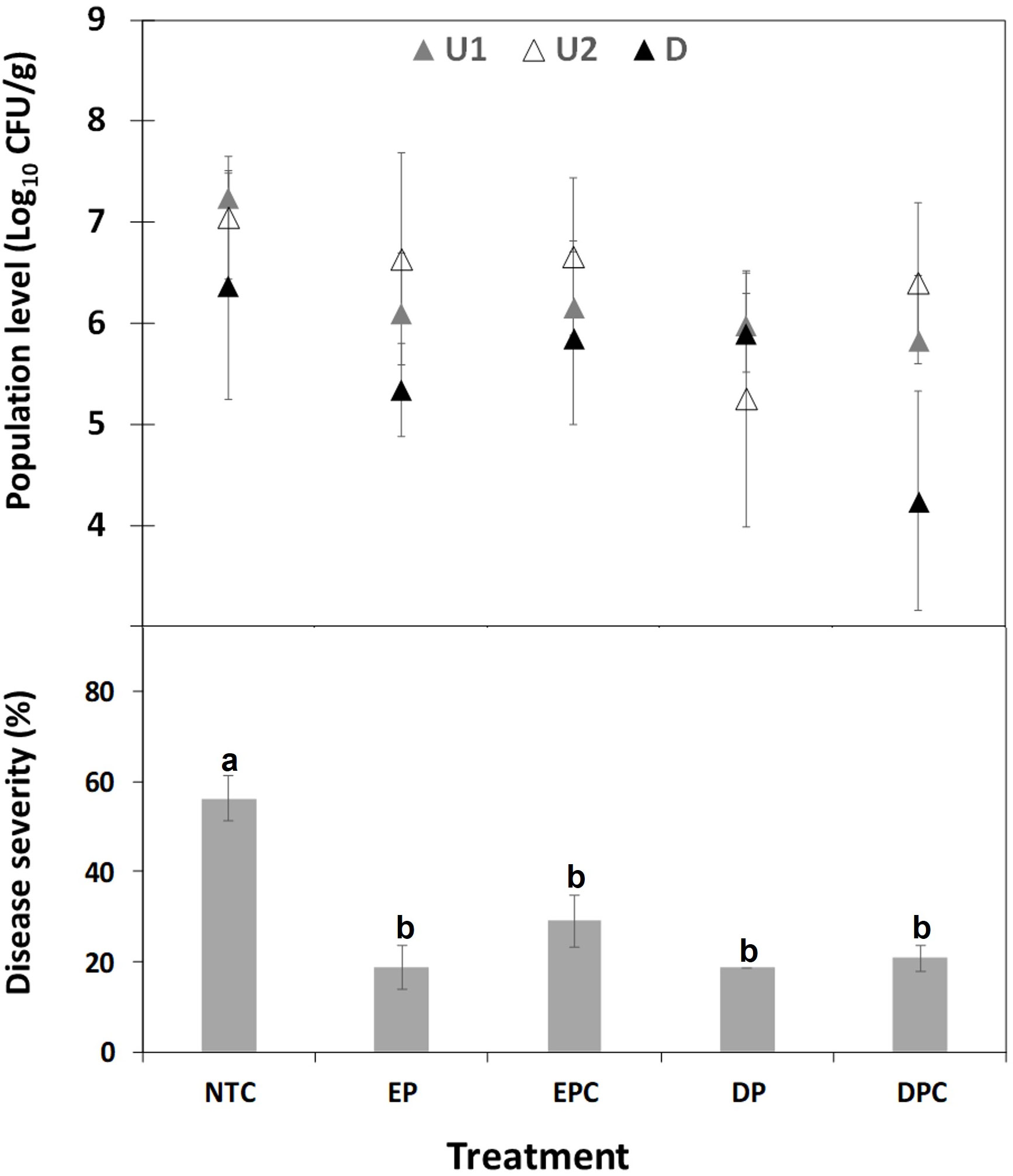
Figure 5 Effect of EGL2 treatment strategies on X. fastidiosa subsp. fastidiosa IVIA 5387.2 population levels in almond plants after 70 days post inoculation. Four independent strategies of EGL2 treatment (60 µl/ml) were used and consisted of: (1) preventive application by endotherapy 1 day before the pathogen inoculation (1dbi, EP); (2) combination of preventive (1 dbi) and curative application by endotherapy 7 and 43 days post-inoculation (dpi, EPC); (3) preventive application (1 dbi, DP) by soil drench, and (4) combination of preventive (1 dbi) and curative application by soil drench 7 and 43 dpi (DPC). Sampled zones are also indicated as upwards zones (U1 and U2) and downwards zone (D), in relation to the point of inoculation and product injection. Values are the means of 6 plants, and error bars represent the standard deviation of the mean. The effect of treatments was significant according to ANOVA (p<0.0001). Different letters between treatments indicate significant differences between disease severities according to Tukey’s test (P ≤ 0.05).
Throughout the second experiment, the levels of X. fastidiosa in almond plants were quantified after the treatment with EGL2, and compared to non-treated control plants after 30 and 70 dpi. At 30 dpi, no significant differences were observed between treatments. The levels in different plant zones (U1, U2, D) after 70 dpi are shown in Figure 5 and Supplementary Figure 1. At 70 dpi, after EGL2 application, a consistent decrease in X. fastidiosa levels was observed in U1, compared to non-treated control plants (reduction of 1.1 to 1.4 logs) by soil drench or endotherapy, in preventive or combined strategies (preventive and curative) (Figure 5 and Supplementary Figure 1). No significant differences were observed between strategies used. The combination of preventive and curative application by endotherapy reduced X. fastidiosa cells in U2 zone, with a decrease of 1.8 logs. The combined treatment using soil drenching was the optimal one in reducing X. fastidiosa cells in the basal zone with a decrease of 2.1 logs.
No phytotoxic effects were observed on the treated almond plants.
3.4 Effect of EGL2 treatment on the expression of defense-related genes in almond
The almond response to EGL2 was determined in almond plants preventively treated by soil drenching or endotherapy, and using a selection of genes related to plant defenses (Foix et al., 2021). The expression of 8 plant defense-related genes was analyzed after 24 h, and transcriptomic changes were only observed when plants were treated by endotherapy (Table 2). Specifically, after endotherapy, transcript levels increased for the genes 7S globulin-like, glutamate receptor, G-type lectin S-receptor-like serine/threonine-protein kinase, pathogenesis-related transcriptional activator PTI5, PR9, RING-H2 finger and pathogenesis-related protein 4, in comparison to non-treated control plants.
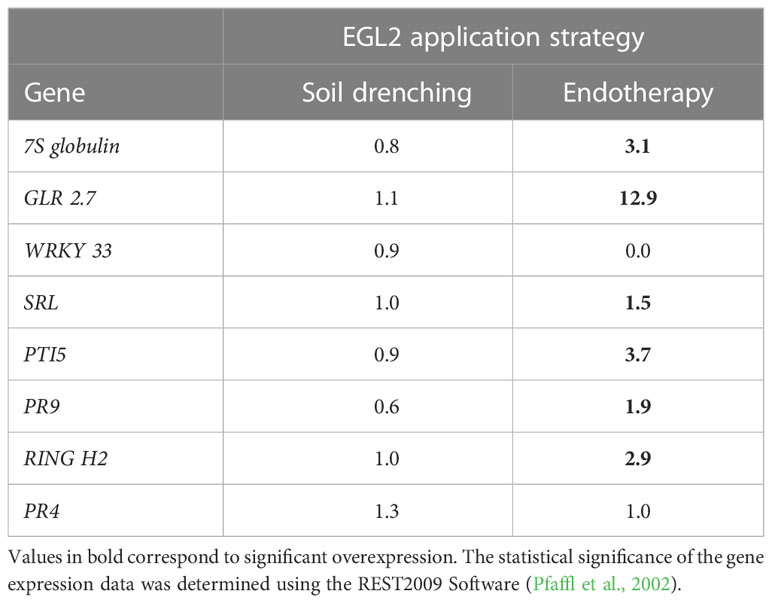
Table 2 Expression of genes related to plant defense response in Prunus dulcis after Eucalyptus essential oil treatments, applied by two different strategies.
4 Discussion
In the present study we investigated the antibacterial properties of a Eucalyptus EO, the ELG2 formulation, against representative plant pathogenic bacteria, and its effect to enhance defense priming responses in a plant host, that finally improved infection control in two bacterial pathosystems.
EGL2 showed a potent in vitro bactericidal activity against E. amylovora, P. syringae pv. syringae, P. syringae pv. tomato, X. campestris pv. vesicatoria, X. arboricola pv. pruni, X. fastidiosa subsp. fastidiosa, X. fastidiosa subsp. pauca and X. fastidiosa subsp. multiplex. The bactericidal activity was dependent on the EO concentration, bacterial strain, and the exposure time, and the most susceptible bacteria were the three subspecies of X. fastidiosa with an MBC of 0.75 µl/ml (750 ppm). Globally, these results agree with reports of other EO showing antibacterial activity against phytopathogenic bacteria such as X. fastidiosa, Agrobacterium tumefaciens or Clavibacter michiganensis (Alonso-Gato et al., 2021). However, it is difficult to compare our data with other studies because of the differences in methods, units of concentration, and bacterial strains used, and in most cases focused food preservation and the medical field. Also, the chemical composition and consequently the antimicrobial activity of EOs, even from a same plant species, can differ due to the method of extraction, its origin, plant organ, geographical location, or climatic conditions (Razzouk et al., 2022). In addition, there are only few reports on Eucalyptus EO (Pandey et al., 2017; Raveau et al., 2020).
Despite these limitations, some comparisons can be done. For example, it has been reported that the MIC of an essential oil of Eucalyptus globulus fruits ranged from 3-4 mg/ml (3000-4000 ppm) against Pseudomonas aeruginosa, Staphylococcus aureus, Bacillus subtilis or Escherichia coli, while the MBC varied between 3.6 to 9.0 mg/ml (3600 to 9000 ppm) (Bakkali et al., 2008; Said et al., 2016). Globulol, the main component of E. globulus fruit petroleum ether fraction of the ethanol crude extract, showed IC50 values on Xanthomonas vesicatoria and Bacillus subtilis of 158.0 µg/ml and 737.2 µg/ml, respectively (Tan et al., 2008). EL-Hefny et al. (2017) find, by disc diffusion susceptibility test, that MICs of extracts of Eucalyptus camaldulensis ranged from 16 to 500 ppm against Pectobacterium carotovorum, Ralstonia solanacearum, Dickeya spp., and Agrobacterium tumefaciens. Similar results were reported with EO extracts from different plants like Rosa damascene or Thymbra spicata against Erwinia amylovora, (MBC 1.4 mg/ml (1400 ppm) and 0.5 mg/ml (500 ppm), respectively), Russowia sogdiana against A. tumefaciens (MIC 0.2-to 0.8 mg/ml (200-800 ppm) and Cleistocalyx peraculatus against X. campestris pv. vesicatoria (MBC 62.5 to 250 µg/ml (62.5 to 250 ppm) (Basim and Basim, 2004; Tan et al., 2007; Bajpai et al., 2010). In the case of Xylella fastidiosa 9a5c, Brentini et al. (2018) determined for a Eucalyptus oil a MIC of 1000 µg/ml, much higher than we observed after exposure to ELG2 of X. fastidiosa subsp. fastidiosa 5387.2, X. fastidiosa subsp. multiplex 5901.2 or X. fastidiosa subsp. pauca De Donno (MICs <5 μl/ml).
The bactericidal activity of EGL2 against the three subspecies of X. fastidiosa was based on a lytic effect, according to TEM ultrastructural imaging. This observation agrees with the fact that terpenes, the main component of essential oils, can disrupt the bacterial cell membrane structure by releasing the lipopolysaccharides and resulting in bacterial cell permeabilization (Sharifi-Rad et al., 2017).
In the present study, apart from the bactericidal effect of EGL2, induced disease tolerance has been associated to overexpression of plant defense genes. To the best of our knowledge, there are no reports on the stimulation of plant defense by Eucalyptus EO. However, our findings agree with the fact that many EOs are involved in host defense mechanisms against plant pathogens, resulting in reduction of disease development (Batish et al., 2008). For example, the foliar application of ethanolic extracts from Giant knotweed induced SA-dependent defense responses in cucumber plants, and reduce powdery mildew severity (Margaritopoulou et al., 2020). Likewise, lavender EO induced overexpression of genes related to SA and ethylene/jasmonic acid pathways in sorghum plants (Rashad et al., 2022). More specifically, we have identified that treatment of almond by endotherapy with EGL2 overexpressed genes coding a vicilin protein (also known as 7S globulin), a glutamate receptor, a G-type lectin S-receptor-like serine/threonine protein kinase, a pathogenesis-related transcriptional activator PTI5, a PR9 (Per44), a RING-H2 finger and a pathogenesis-related protein 4 (PR4).
Glutamate receptors, G-type lectin S-receptor-like serine/threonine protein kinases (LecRLKs) and pathogenesis-related transcriptional activators PTI5 are described as positive regulators of PAMP triggered immunity (PTI) (Luo et al., 2017). In addition, glutamate receptor also responds to an attack from pathogens (and wounding) by mediating PTI by acting as Ca2+ channels (Forde and Roberts, 2014). LecRLK has been also described as a positive regulator of plant tolerance to salt stress (Sun et al., 2013) and plays crucial roles in plant development and responses to biotic and abiotic stresses (Han et al., 2021). In case of LecRLK overexpression, it has been reported that pathogen infection due to P. syringae DC3000 activates not only the transcription of LecRLK in Arabidopsis, but also plants overexpressing LecRLK show not only resistant phenotype to P. syringae DC3000 but also reactive oxygen species (ROS)-production and SA accumulation (Luo et al., 2017). In relation to PTI5 it is known that belongs to the ethylene-response factor (ERF) family and binds to promoters of many pathogenesis-related (PR) genes (Yong-Qiang et al., 2002), and so overexpression of PTI5 in tomato enhanced protection to P. syringae pv. tomato (He et al., 2001) while overexpression in Arabidopsis activated the expression of SA-regulated genes (PR1 and PR2 genes) (Gu et al., 2002).
Additionally, the pathogenesis-related proteins PR4 and PR9, and vicilin, also seem to contribute to highest tolerance to X. fastidiosa infection. Specifically, the induction of PR9 (Per44) is related with plant defense against pathogen attack and environmental stresses, being a key component of ROS production during plant defense responses (Soylu et al., 2005). Moreover, PR9 plays a key role in lignin biosynthesis and biodegradation, contributing to strengthening plant cell walls by catalyzing lignin deposition (Taheri and Tarighi, 2012). Likewise, PR4 is crucial against a fungal attack, but also increased its synthesis by other biotic factors such as bacteria, virus, insects as well as abiotic stresses, SA, or ethylene (Sharma et al., 2011; Grove, 2012), and vicilin is a multifunctional protein related to stress responses, antibacterial activity, and hormone receptor-like activity, and in some plants are described as a precursor of antimicrobial peptides (Hirano, 2021).
We have demonstrated here that the preventive application of EGL2 applied by spraying to pear plants reduced E. amylovora infections with an efficacy close to 40%. Since the first report on the antibacterial activity of the active substances derived from EO (i.e., the terpenoids geraniol and citronellol) against E. amylovora (Scortichini and Rossi, 1991), several studies have been conducted using detached organs (Măruţescu et al., 2009; Akhlaghi et al., 2020), but none demonstrating the effectiveness of EO treatment in fire blight control using in planta assays. In addition, EGL2 applied to almond plants by different strategies (endotherapy and soil drenching, preventive, curative or a combination of both), reduced severity of infections caused by X. fastidiosa, under greenhouse conditions, with efficacies varying from 50 to 70%. The reduction in ALS symptoms was associated to a reduction in levels of X. fastidiosa in treated plants, although pathogen was not eliminated. Our results of efficacy of Eucalyptus EO, agree with the field reports of different compounds such as a biocomplex of zinc, copper and citric acid, N-acetylcysteine, fosetyl-aluminum, bioactive detergent from plants, diffusible signal factor, as well as endophytic microorganisms, avirulent/weakly virulent X. fastidiosa strains or bacteriophages, that in some cases reduced symptoms in infected plants, but were not able to eliminate X. fastidiosa from diseased plants (EFSA PLH Panel et al., 2019; Morelli et al., 2021).
In conclusion, our study demonstrates that a formulation of Eucalyptus EO has a strong bactericidal effect and can protect almond plants from X. fastidiosa and pear plants from E. amylovora infections, on plants under greenhouse conditions. The EGL2 acts by a dual mechanism, directly against the target plant pathogenic bacteria and indirectly by eliciting defense responses in the host plant. This bifunctional mechanism of action and the fact that can be applied using different methods (irrigation, endotherapy, spray) demonstrates its potential for controlling bacterial diseases of plants.
Data availability statement
The original contributions presented in the study are included in the article/Supplementary Materials, further inquiries can be directed to the corresponding author/s.
Author contributions
EM obtained the financial support. EM and LM designed research and wrote the paper. LM, AB, and BG conducted, performed the experiments, and analyzed de data. All authors contributed to the article and approved the submitted version.
Funding
This work was supported by grants from the European Union (BeXyL Ref. 101060593), and from Ministerio de Ciencia, Innovación y Universidades of Spain (XF-Peps Ref. TED2021-130110B-C43). AB was recipient of a research grant from the Secretaria d’Universitats i Recerca, Departament d’Economia i Coneixement, Generalitat de Catalunya (Ref.2018 FI B00334).
Acknowledgments
We thank Lainco S.A. for providing the Eucalyptus essential oil formulation. We also thank Héctor Saravia and Gemma Roselló for participating in various tasks related to the preparation and maintenance of plant material.
Conflict of interest
The authors declare that the research was conducted in the absence of any commercial or financial relationships that could be construed as a potential conflict of interest.
Publisher’s note
All claims expressed in this article are solely those of the authors and do not necessarily represent those of their affiliated organizations, or those of the publisher, the editors and the reviewers. Any product that may be evaluated in this article, or claim that may be made by its manufacturer, is not guaranteed or endorsed by the publisher.
Supplementary material
The Supplementary Material for this article can be found online at: https://www.frontiersin.org/articles/10.3389/fpls.2023.1122218/full#supplementary-material
Supplementary Figure 1 | Effect of EGL2 treatment strategies on X. fastidiosa subsp. fastidiosa IVIA 5387.2 population levels in almond plants after 70 days post inoculation. Four independent strategies of EGL2 treatment (60 µl/ml) were used and consisted of: (1) preventive application by endotherapy 1 day before the pathogen inoculation (1dbi, EP); (2) combination of preventive (1 dbi) and curative application by endotherapy 7 and 43 days post-inoculation (dpi, EPC); (3) preventive application (1 dbi, DP) by soil drench, and (4) combination of preventive (1 dbi) and curative application by soil drench 7 and 43 dpi (DPC). Sampled zones are also indicated as upwards zones (U1 and U2) and downwards zone (D), in relation to the point of inoculation and product injection. Values are the means of 6 plants, and error bars represent the standard deviation of the mean. Different letters between zones indicate significant differences between population levels of X. fastidiosa according to Tukey’s test (P ≤ 0.05).
References
Akhlaghi, M., Tarighi, S., Taheri, P. (2020). Effects of plant essential oils on growth and virulence factors of Erwinia amylovora. J. Plant Pathol. 102, 409–419. doi: 10.1007/s42161-019-00446-9
Alonso-Gato, M., Astray, G., Mejuto, J. C., Simal-Gandara, J. (2021). Essential oils as antimicrobials in crop protection. Antibiotics (Basel). 10, 34. doi: 10.3390/antibiotics10010034
Amanifar, N., Taghavi, M., Salehi, M. (2016). Xylella fastidiosa from almond in Iran: overwinter recovery and effects of antibiotics. Phytopathol. Mediterr. 55, 337–345.doi: 10.14601/Phytopathol_Mediterr-17682
Baccari, C., Antonova, E., Lindow, S. (2019). Biological control of pierce’s disease of grape by an endophytic bacterium. Phytopathol. 109, 248–256. doi: 10.1094/PHYTO-07-18-0245-FI
Bachir, R. G., Benali, M. (2012). Antibacterial activity of the essential oils from the leaves of Eucalyptus globulus against Escherichia coli and Staphylococcus aureus. Asian Pacific J. Trop. Biomed. 2, 739–742. doi: 10.1016/S2221-1691(12)60220-2
Badosa, E., Montesinos, L., Camó, C., Ruz, L., Cabrefiga, J., Francés, J., et al. (2017). Control of fire blight infections with synthetic peptides that elicit plant defense. J. of Plant Pathol. 99, 65–73.
Bajpai, V. K., Dung, N. T., Suh, H. J., Kang, S. C. (2010). Antibacterial activity of essential oil and extracts of Cleistocalyx operculatus buds against the bacteria of Xanthomonas spp. J. Am. Oil Chem. Soc. 87, 1341–1349. doi: 10.1007/s11746-010-1623-9
Bakkali, F., Averbeck, S., Averbeck, D., Idaomar, M. (2008). Biological effects of essential oils–a review. Food Chem. Toxicol. 46, 446–475. doi: 10.1016/j.fct.2007.09.106
Baldi, P., La Porta, N. (2017). Xylella fastidiosa: host range and advance in molecular identification techniques. Front. Plant Sci. 8. doi: 10.3389/fpls.2017.00944
Baró, A., Badosa, E., Montesinos, L., Feliu, L., Planas, M., Montesinos, E., et al. (2020b). Screening and identification of BP100 peptide conjugates active against xylella fastidiosa using a viability-qPCR method. BMC Microbiol. 20, 229. doi: 10.1186/s12866-020-01915-3
Baró, A., Montesinos, L., Badosa, E., Montesinos, E. (2021). Aggressiveness of Spanish isolates of Xylella fastidiosa to almond plants of different cultivars under greenhouse conditions. Phytopathol. 111, 1994–2001. doi: 10.1094/PHYTO-02-21-0049-R
Baró, A., Mora, I., Montesinos, L., Montesinos, E. (2020a). Differential susceptibility of Xylella fastidiosa strains to synthetic bactericidal peptides. Phytopathol. 110, 1018–1026. doi: 10.1094/PHYTO-12-19-0477-R
Basim, E., Basim, H. (2004). Evaluation of antibacterial activity of essential oil of Rosa damascena on Erwinia amylovora. Phytoparasitica. 32, 409–412. doi: 10.1007/BF02979853
Batish, D. R., Singh, H. P., Kohli, R. K., Kaur, S. (2008). Eucalyptus essential oil as a natural pesticide. For. Ecol. Manage. 256, 2166–2174. doi: 10.1016/j.foreco.2008.08.008
Blaustein, R. A., Lorca, G. L., Teplitski, M. (2019). Challenges for managing candidatus liberibacter spp. (Huanglongbing disease pathogen): Current control measures and future directions. Phytopathol. 108, 424–435. doi: 10.1094/PHYTO-07-17-0260-RVW
Brentini, M., da Silva, T., Estétele, J., Oliveira, L., Lucarini, R., Fernandes, D., et al. (2018). In vitro of essential oils for potentional antibacterial effects against Xylella fastidiosa. J. of Phytopathol. 166, 790–798. doi: 10.1111/jph.12762
Bruno, G. L., Cariddi, C., Botrugno, L. (2021). Exploring a sustainable solution to control Xylella fastidiosa subsp. pauca on olive in the salento peninsula, southern Italy. Crop Prot. 139, 105288. doi: 10.1016/j.cropro.2020.105288
Denancé, N., Briand, M., Gaborieau, R., Gaillard, S., Jacques, M.-A. (2019). Identification of genetic relationships and subspecies signatures in Xylella fastidiosa. BMC Genomics 20, 239. doi: 10.1186/s12864-019-5565-9
Dongiovanni, C., Di Carolo, M., Fumarola, G., Ciniero, A., Tauro, D., Palmisano, F., et al. (2017). Recenti sperimentazioni per il controllo di xylella. Olivo e Olio. 4, 25–29. doi: 10.5281/zenodo.833397
EC, Plant protection in EU agriculture (2022). Farm to fork: New rules to reduce the risk and use of pesticides in the EU (Brussels). Available at: https://ec.europa.eu/commission/presscorner/detail/en/qanda_22_3694.
EFSA (2021). Update of the Xylella spp. host plant database–systematic literature searches up to 31 December 2020. EFSA J. 19, 6674. doi: 10.2903/j.efsa.2021.6674
EFSA (2022). Update of the Xylella spp. host plant database - systematic literature search up to 31 December 2021. EFSA J. 20, 7356. doi: 10.2903/j.efsa.2022.7356
EFSA PLH Panel, Bragard, C., Dehnen-Schmutz, K., DiSerio, F., Gonthier, P., Jacques, M. A., et al. (2019). Scientific opinion on the effectiveness of in planta control measures for xylella fastidiosa. EFSA J. 17, 5666. doi: 10.2903/j.efsa.2019.5665
EFSA PLH Panel, Jeger, M., Caffier, D., Candresse, T., Chatzivassiliou, E., Dehnen-Schmutz, K., et al. (2018). Scientific opinion on the updated pest categorization of Xylella fastidiosa. EFSA J. 16, 5357. doi: 10.2903/j.efsa.2018.5357
EL-Hefny, M., Ashmawy, N. A., Salem, M. Z. M., Abdelfattah, Z. M. S. (2017). Antibacterial activities of the phytochemicals-characterized extracts of Callistemon viminalis, eucalyptus camaldulensis and conyza dioscoridis against the growth of some phytopathogenic bacteria. Microb. Pathog. 113, 348–356. doi: 10.1016/j.micpath.2017.11.004
EPPO (2006). PM 3/64 (1) intentional import of organisms that are plant pests or potential plant pests. EPPO Bull. 36, 191–194. doi: 10.1111/j.1365-2338.2006.00908.x
Foix, L., Nadal, A., Zagorščak, M., Ramšak, Ž., Esteve-Codina, A., Gruden, K., et al. (2021). Prunus persica plant endogenous peptides PpPep1 and PpPep2 cause PTI-like transcriptome reprogramming in peach and enhance resistance to Xanthomonas arboricola pv. pruni. BMC Genomics 22, 360. doi: 10.1186/s12864-021-07571-9
Forde, B. G., Roberts, M. R. (2014). Glutamate receptor-like channels in plants: a role as amino acid sensors in plant defence? F1000Prime Rep. 6, 37. doi: 10.12703/P6-37
Grove, A. (2012). Plant chitinases: Genetic diversity and physiological roles. CRC Crit. Rev. Plant Sci. 31, 57–73. doi: 10.1080/07352689.2011.616043
Gu, Y. Q., Wildermuth, M. C., Chakravarthy, S., Loh, Y. T., Yang, C., He, X., et al. (2002). Tomato transcription factors pti4, pti5, and pti6 activate defense responses when expressed in Arabidopsis. Plant Cell. 14, 817–831. doi: 10.1105/tpc.000794
Han, H., Mu, X., Wang, P., Wang, Z., Fu, H., Gao, Y. G., et al. (2021). Identification of LecRLK gene family in Cerasus humilis through genomic-transcriptomic data mining and expression analyses. PloS One 16, e0254535. doi: 10.1371/journal.pone.0254535
He, P., Warren, R. F., Zhao, T., Shan, L., Zhu, L., Tang, X., et al. (2001). Overexpression of Pti5 in tomato potentiates pathogen-induced defense gene expression and enhances disease resistance to Pseudomonas syringae pv. tomato. Mol. Plant Microbe Interact. 14, 1453–1457. doi: 10.1094/MPMI.2001.14.12.1453
Hirano, H. (2021). Basic 7S globulin in plants. J. Proteomics. 240, 104209. doi: 10.1016/j.jprot.2021.104209
Iacobellis, N. S., Lo Cantore, P., Capasso, F., Senatore, F. (2005). Antibacterial activity of cuminum cyminum l. and carum carvi l. essential oils. J. Agric. Food Chem. 53, 57–61. doi: 10.1021/jf0487351
IPPC Secretariat (2021a). Scientific review of the impact of climate change on plant pests – a global challenge to prevent and mitigate plant pest risks in agriculture, forestry and ecosystems (Rome: FAO on behalf of the IPPC Secretariat). Available at: https://www.fao.org/documents/card/en/c/cb4769en.
IPPC Secretariat (2021b). Strategic framework for the international plant protection convention (IPPC) 2020–2030. protecting global plant resources and facilitating safe trade (Rome: FAO on behalf of the Secretariat of the International Plant Protection Convention).
Karami-Osboo, R., Khodaverdi, M., Ali-Akbari, F. (2010). Antibacterial effect of effective compounds of Satureja hortensis and Thymus vulgaris essential oils against erwinia amylovora. J. Agr. Sci. Tech. 12, 35–45.
Leahy, J., Mendelsohn, M., Kough, J., Jones, R., Berckes, N. (2014). Biopesticides: states of the art and future opportunities. Eds. Gross, A., Coats, J. R., Duke, S. O., Seiber, J. N. (Washington, DC: American Chemical Society), 3–18. doi: 10.1021/jf504252n
Livak, K. J., Schmittgen, T. D. (2001). Analysis of relative gene expression data using real-time quantitative PCR and the 2-ΔΔCT method. Methods. 25, 402–408. doi: 10.1006/meth.2001.1262
Luo, X., Xu, N., Huang, J., Gao, F., Zou, H., Boudsocq, M., et al. (2017). A lectin receptor-like kinase mediates pattern-triggered salicylic acid signaling. Plant Physiol. 174, 2501–2514. doi: 10.1104/pp.17.00404
Margaritopoulou, T., Toufexi, E., Kizis, D., Balayiannis, G., Theocharis, A., Rempelos, L., et al. (2020). Reynoutria sachalinensis extract elicits SA-dependent defense responses in courgette genotypes against powdery mildew caused by Podosphaera xanthii. Sci. Rep. 10, 3354. doi: 10.1038/s41598-020-60148-6
Măruţescu, L., Saviuc, C., Oprea, E., Savu, B., Bucur, M., Stanciu, G., et al. (2009). In vitro susceptibility of Erwinia amylovora (Burrill) Winslow et. al. to Citrus maxima essential oil. Roum Arch. Microbiol. Immunol. 68, 223–227.
Mohan, M., Haider, S. Z., Andola, H. C., Purohit, V. K. (2011). Essential oils as green pesticides: for sustainable agriculture. Res. J. Pharm. Biol. Chem. Sci. 2, 100–106.
Moll, L., Baró, A., Montesinos, L., Badosa, E., Bonaterra, A., Montesinos, E. (2022). Induction of defense responses and protection of almond plants against Xylella fastidiosa by endotherapy with a bifunctional peptide. Phytopathol. 112, 1907–1916. doi: 10.1094/PHYTO-12-21-0525-R
Montesinos, L., Gascón, B., Ruz, L., Badosa, E., Planas, M., Feliu, L., et al. (2021). A bifunctional synthetic peptide with antimicrobial and plant elicitation properties that protect tomato plants from bacterial and fungal infections. Front. Plant Sci. 12. doi: 10.3389/fpls.2021.756357
Morelli, M., García-Madero, J. M., Jos, Á., Saldarelli, P., Dongiovanni, C., Kovacova, M., et al. (2021). Xylella fastidiosa in olive: A review of control attempts and current management. Microorganisms. 9, 1771. doi: 10.3390/microorganisms9081771
Olmo, D., Nieto, A., Borràs, D., Montesinos, M., Adrover, F., Pascual, A., et al. (2021). Landscape epidemiology of Xylella fastidiosa in the Balearic islands. Agronomy. 11, 473. doi: 10.3390/agronomy11030473
Palacio-Bielsa, A., Roselló, M., Cambra, M. A., López, M. M. (2010). First report on almond in Europe of bacterial spot disease of stone fruits caused by Xanthomonas arboricola pv. pruni. Plant Dis. 94, 786. doi: 10.1094/PDIS-94-6-0786B
Pandey, A. K., Kumar, P., Singh, P., Tripathi, N. N., Bajpai, V. K. (2017). Essential oils: sources of antimicrobials and food preservatives. Front. Microbiol. 16. doi: 10.3389/fmicb.2016.02161
Pathma, J., Kennedy, R. K., Bhushan, L. S., Shankar, B. K., Thakur, K. (2021). “Microbial biofertilizers and biopesticides: nature’s assets fostering sustainable agriculture,” in Recent developments in microbial technologies. environmental and microbial biotechnology. Eds. Prasad, R., Kumar, V., Singh, J., Upadhyaya, C. P. (Singapore: Springer). doi: 10.1007/978-981-15-4439-2_2
Pfaffl, M. W., Horgan, G. W., Dempfle, L. (2002). Relative expression software tool (REST) for group-wise comparison and statistical analysis of relative expression results in real-time PCR. Nucleic Acids Res. 30, e36. doi: 10.1093/nar/30.9.e36
Ramasamy, A. D., Bokshi, A. I., Phan-Thien, K., McConchie, R. M. (2015). Seed treatment with benzothiadiazole induces resistance against powdery mildew disease caused by Sphaerotheca fuliginea and increases the activities of pathogenesis-related enzymes in cucumber plants. J. Hortic. Sci. Biotechnol. 90, 63–70. doi: 10.1080/14620316.2015.11513154
Rashad, Y. M., Abdel Razik, E. S., Darwish, D. B. (2022). Essential oil from Lavandula angustifolia elicits expression of three SbWRKY transcription factors and defense-related genes against sorghum damping-off. Sci. Rep. 12, 857. doi: 10.1038/s41598-022-04903-x
Raveau, R., Fontaine, J., Lounès-Hadj Sahraoui, A. (2020). Essential oils as potential alternative biocontrol products against plant pathogens and weeds: a review. Foods. 9, 365. doi: 10.3390/foods9030365
Razzouk, S., Mazri, M. A., Jeldi, L., Mnasri, B., Ouahmane, L., Alfeddy, M. N. (2022). Chemical composition and antimicrobial activity of essential oils from three Mediterranean plants against eighteen pathogenic bacteria and fungi. Pharmaceutics. 14, 1608. doi: 10.3390/pharmaceutics14081608
Ruiz, C., Nadal, A., Montesinos, E., Pla, M. (2017). Novel Rosaceae plant elicitor peptides as sustainable tools to control Xanthomonas arboricola pv. pruni in prunus spp. Mol. Plant Pathol. 19, 418–431. doi: 10.1111/mpp.12534
Said, Z., Haddadi-Guemghar, H., Boulekbache-Makhlouf, L., Rigou, P., Remini, H., Adjaoud, A., et al. (2016). Essential oils composition, antibacterial and antioxidant activities of hydrodistillated extract of Eucalyptus globulus fruits. Ind. Crops Prod. 89, 167–175. doi: 10.1016/j.indcrop.2016.05.018
Scortichini, M., Chen, J., De Caroli, M., Delessandro, G., Pucci, N., Modesti, V., et al. (2018). A zinc, cooper and citric acid biocomplex shows promise for control of Xylella fastidiosa subsp. pauca in olive trees in apulia region (Southern Italy). Phytopato.l Mediterr. 57, 48–72. doi: 10.14601/Phytopathol_Mediterr-21985
Scortichini, M., Rossi, M. P. (1991). In vitro susceptibility of Erwinia amylovora (Burrill) Winslow et al. to geraniol and citronellol. J. Appl. Bacteriol. 71 (2), 113–118. doi: 10.1111/j.1365-2672.1991.tb02964.x
Sharifi-Rad, J., Salehi, B., Varoni, E. M., Sharopov, F., Yousaf, Z., Ayatollahi, S. A., et al. (2017). Plants of the Melaleuca genus as antimicrobial agents: from farm to pharmacy. Phytother. Res. 31, 1475–1494. doi: 10.1002/ptr.5880
Sharma, A., Abrahamian, P., Carvalho, R., Choudhary, M., Paret, M. L., Vallad, G. E., et al. (2022). Future of bacterial disease management in crop production. Annu. Rev. Phytopathol. 60, 259–282. doi: 10.1146/annurev-phyto-021621-121806
Sharma, N., Sharma, K. P., Gaur, R. K., Gupta, V. K. (2011). Role of chitinase in plant defense. Asian J. Biochem. 6, 29–37. doi: 10.3923/ajb.2011.29.37
Soylu, S., Brown, I., Mansfield, J. W. (2005). Cellular reactions in Arabidopsis following challenge by strains of Pseudomonas syringae: from basal resistance to compatibility. Physiol. Mol. Plant Pathol. 66, 232–243. doi: 10.1016/j.pmpp.2005.08.005
Sun, X. L., Yu, Q. Y., Tang, L. L., Ji, W., Bai, X., Cai, H., et al. (2013). GsSRK, a G-type lectin s-receptor-like serine/threonine protein kinase, is a positive regulator of plant tolerance to salt stress. J. Plant Physiol. 170, 505–515. doi: 10.1016/j.jplph.2012.11.017
Sundin, G. W., Castiblanco, L. F., Yuan, X., Zeng, Q., Yang, C.-H. (2016). Bacterial disease management: challenges, experience, innovation and future prospects. Mol. Plant Pathol. 17, 1506–1518. doi: 10.1111/mpp.12436
Taheri, P., Tarighi, S. (2012). The role of pathogenesis-related proteins in the tomato-Rhizoctonia solani interaction. J. Bot. 12, 6 doi: 10.1155/2012/137037
Tan, M., Zhou, L., Huang, Y., Wang, Y., Hao, X., Wang, J. (2008). Antimicrobial activity of globulol isolated from the fruits of Eucalyptus globulus labill. Natural Product Res. 22, 569–575. doi: 10.1080/14786410701592745
Tan, M., Zhou, L., Qin, M., Li, D., Jiang, W., Wang, Y., et al. (2007). Chemical composition and antimicrobial activity of the flower oil of Russowia sogdiana (Bunge) b. Fedtsch. (Asteraceae) from China. J. Essent. Oil Res. 19, 197–200. doi: 10.1080/10412905.2007.9699258
Terzi, V., Morcia, C., Faccioli, P., Valè, G., Tacconi, G., Malnati, M. (2007). In vitro antifungal activity of the tea tree (Melaleuca alternifolia) essential oil and its major components against plant pathogens. Lett. Appl. Microbiol. 44, 613–618. doi: 10.1111/j.1472-765X.2007.02128.x
Tong, Z., Gao, Z., Wang, F., Zhou, J., Zhang, Z. (2009). Selection of reliable reference genes for gene expression studies in peach using real-time PCR. BMC Mol. Biol. 10, 1–3. doi: 10.1186/1471-2199-10-71
Vanneste, J. L., Voyle, M. D., Yu, J., Cornish, D. A., Boyd, R. J., Mclaren, G. F. (2008). “Copper and streptomycin resistance in pseudomonas strains isolated from pipfruit and stone fruit orchards in new Zealand,” in Pseudomonas syringae pathovars and related pathogens – identification, epidemiology and genomics (Dordrecht: Springer). doi: 10.1007/978-1-4020-6901-7_9
White, S. M., Navas-Cortés, J. A., Bullock, J. M., Boscia, D., Chapman, D. S. (2020). Estimating the epidemiology of emerging Xylella fastidiosa outbreaks in olives. Plant Pathol. 69, 1403–1413. doi: 10.1111/ppa.13238
Yong-Qiang, G., Wildermuth, M., Chakravarthy, S., Loh, Y., Yang, C., He, X., et al. (2002). Tomato transcription factors Pti4, Pti5, and Pti6 activate defense responses when expressed in arabidopsis. The Plant Cell. 14, 817–831. doi: 10.1105/tpc.000794
Keywords: eucalyptus oil, bactericidal, plant defense elicitor, plant infections, Erwinia amylovora, Xylella fastidiosa
Citation: Montesinos L, Baró A, Gascón B and Montesinos E (2023) Bactericidal and plant defense elicitation activities of Eucalyptus oil decrease the severity of infections by Xylella fastidiosa on almond plants. Front. Plant Sci. 14:1122218. doi: 10.3389/fpls.2023.1122218
Received: 12 December 2022; Accepted: 27 February 2023;
Published: 15 March 2023.
Edited by:
Yanan Wang, Hebei Agricultural University, ChinaReviewed by:
Kubilay Kurtulus Bastas, Selçuk University, TürkiyeManoj Choudhary, University of Florida, United States
Copyright © 2023 Montesinos, Baró, Gascón and Montesinos. This is an open-access article distributed under the terms of the Creative Commons Attribution License (CC BY). The use, distribution or reproduction in other forums is permitted, provided the original author(s) and the copyright owner(s) are credited and that the original publication in this journal is cited, in accordance with accepted academic practice. No use, distribution or reproduction is permitted which does not comply with these terms.
*Correspondence: Emilio Montesinos, ZW1pbGlvLm1vbnRlc2lub3NAdWRnLmVkdQ==