- 1Agricultural and Bio Resource Development Department, Innovation Division, KAGOME CO., LTD., Nasushiobara, Japan
- 2Institute of Vegetable and Floriculture Science, National Agriculture and Food Research Organization, Tsu, Japan
Glucosinolates (GSLs), precursors of isothiocyanates (ITCs), are present in Brassicaceae plants have been found to have health benefits. Sulforaphane (4-(methylsulfinyl)butyl ITC) is an ITC stored in the form of 4-(methylsulfinyl)butyl GSL (glucoraphanin, 4MSOB) in Brassica vegetables, such as broccoli and kale. Sulforaphane activates Nrf2 expression, a transcription factor responsible for inducing physiological activities such as detoxification in the human body, and it represents a functional component unique to cruciferous vegetables. Raphanobrassica is an inter-generic hybrid between radish and kale, and it contains a high amount of 4MSOB. However, Raphanobrassica contains as much 4-methylsulfinyl-3-butenyl GSL (glucoraphenin, 4MSO3B) as it does 4MSOB. GLUCORAPHASATIN SYNTHASE 1 (GRS1) is an enzyme present in radish that synthesizes 4-methylthio-3-butenyl GSL (glucoraphasatin, 4MT3B), a precursor of 4MSO3B, using 4-(methylthio)butyl GSL (glucoerucin, 4MTB) as a substrate. Since the precursor of 4MSOB is also 4MTB, it was considered that both 4MSOB and 4MSO3B accumulate owing to competition in Raphanobrassica. We hypothesized that owing to the impaired function of GRS1 in Raphanobrassica, it may be possible to breed Raphanobrassica cultivars containing a high 4MSOB content. In this study, we generated Raphanobrassica populations with functional and defective GRS1 and compared the GSL composition in the two populations using high-performance liquid chromatography. The mean 4MSOB content in leaves of the defective-type populations was higher than that in the functional-type population, and the defective/functional ratio ranged from 2.02 to 2.51-fold, supporting this hypothesis. Furthermore, leaves, flower buds, stems, and roots contained higher amounts of 4MSOB in the defective population than in the functional population. The leaf 4MSOB content of defective Raphanobrassica grown in this study was comparable to that of previously studied vegetables (such as broccoli sprouts) with high 4MSOB content. Raphanobrassica with defective GRS1 represents a new leafy vegetable with high 4MSOB content which exhibits anti-cancerous and anti-inflammatory potentials.
1 Introduction
More than 500 plant species, primarily cruciferous plants, have been found to contain glucosinolates (GSLs). The common structure of GSLs consists of a β-D-glucopyranose residue linked by a sulfur atom to a (Z)-N-hydroximinosulfate ester, plus a variable side chain derived from precursor amino acids (Fahey et al., 2001; Halkier and Gershenzon, 2006). Based on the type of amino acid from which they are produced, GSLs can be categorized as aliphatic, benzenic, and indolic GSLs. Methionine (or alanine, isoleucine, leucine, and valine as alternatives), phenylalanine, and tryptophan are precursors of aliphatic, benzenic, and indolic GSLs, respectively. When plant tissues are damaged by herbivores and/or during infection, GSLs are hydrolyzed by myrosinases to isothiocyanates, thiocyanates, nitriles, or epithionitriles, depending on pH and the presence of epithiospecifier protein (Bones and Rossiter, 1996; Rask et al., 2000).
4-(Methylsulfinyl)butyl GSL (glucoraphanin, 4MSOB), which is a precursor of sulforaphane, is an aliphatic GSL. Sulforaphane has several physiological activities, one of which involves the activation of the transcription factor NF-E2–related factor 2 (Nrf2) (Kensler et al., 2013; Yuanfeng et al., 2021). Nrf2 is a master regulator of detoxification and antioxidants, and it controls the expression of downstream antioxidant genes and phase II detoxification enzyme genes by activating the oxidation response element (Chen and Maltagliati, 2018). The health benefits of sulforaphane have been widely studied in humans; sulforaphane intake reduces the level of urinary 8-hydroxyguanosine, an oxidative stress marker, and it decreases gamma-glutamyl transpeptidase and alanine transaminase levels, which are indicators of liver dysfunction (Kikuchi et al., 2015). Cognitive function is improved by the simultaneous intervention of sulforaphane intake and brain training (Nouchi et al., 2021). In addition, sulforaphane intake mediates the excretion of mycotoxins and air pollutants and improves mild asthma symptoms (Kensler et al., 2005; Riedl et al., 2009; Egner et al., 2014).
4MSOB accumulates in certain Brassica oleracea vegetables (2n = 2x = 18, CC genome; Figure 1A) (Cartea and Velasco, 2007). Broccoli (B. oleracea var. italica) contains the highest amount of 4MSOB among the currently evaluated Brassica vegetables. Farnham et al., (2004) reported that the average of 4MSOB concentration in 32 broccoli variety was 0.36 µmol·g-1 fresh weight and the range of 4MSOB was 0.24 to 1.85 µmol·g-1 fresh weight. It is known that 4MSOB accumulates in all tissues of broccoli and its content is particularly high in mature seeds and seedlings (Yagishita et al., 2019). 4MSOB is also known to be contained in red cabbage (Wermter et al., 2020). Several studies have attempted to increase the 4MSOB content in broccoli, and high 4MSOB-containing broccoli has been bred from crosses between a closely related wild species, Brassica villosa, and broccoli (Traka et al., 2013). Recently, it was suggested that MYB28 derived from B. villosa increases the transcription of genes that encode GSL biosynthesis enzymes in broccoli (Neequaye et al., 2022). Another 4MSOB-rich vegetable is allopolyploid Raphanobrassica (2n = 4x = 36, RRCC), an intergeneric hybrid of the genera Raphanus and Brassica. Initially, it was primarily used in cytogenetic studies to achieve the introduction of valuable traits (Karpechenko, 1924; McNaughton, 1973). Raphanobrassica contains 4MSOB, which is present in high concentrations in B. oleracea, and it contains 4-methylsulfinyl-3-butenyl GSL (glucoraphenin, 4MSO3B), which is in the leaves of Raphanus plants (Schutze et al., 1999; Niimi et al., 2015). The ratio of 4MSOB to 4MSO3B content in Raphanobrassica is lower; therefore, the development of a cultivar with a relatively higher 4MSOB content is desirable.
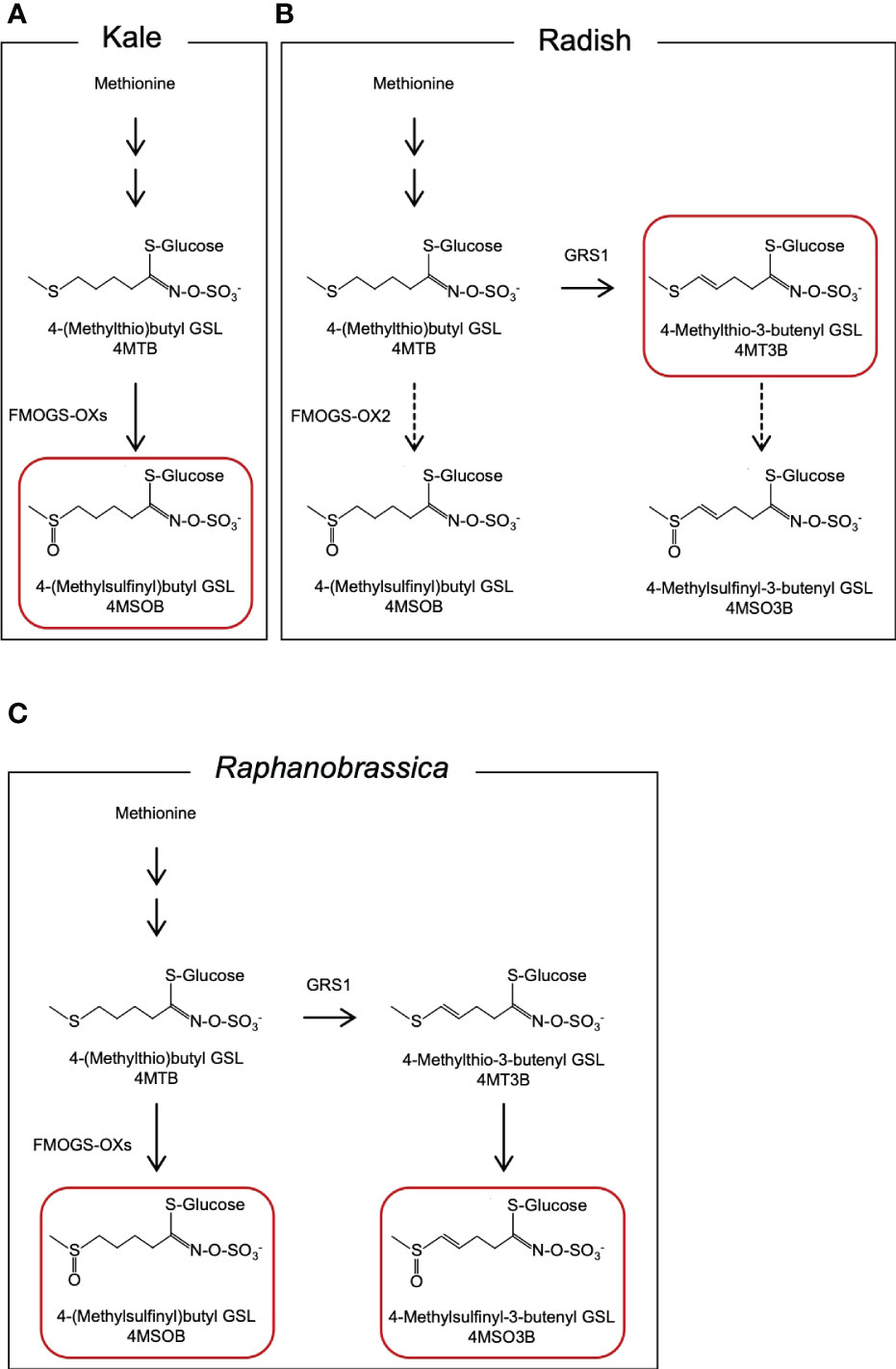
Figure 1 Presumed metabolic pathway of aliphatic GSLs in (A) kale, (B) radish, and (C) Raphanobrassica leaves. GSL, glucosinolate. The GSLs indicated by red boxes are the major GSLs in each species.
The radish GLUCORAPHASATIN SYNTHASE 1 (GRS1) gene encodes a 2-oxoglutarate-dependent dioxygenase that presumably desaturates the 4-(methylthio)butyl GSL (glucoerucin, 4MTB) side chain (Figure 1B). A GRS1 insertional mutants (grs1) are known for their high 4MTB accumulation (Kakizaki et al., 2017). Based on these facts, we hypothesized that metabolism would shift towards 4MSOB synthesis in radish lines containing the grs1 allele as a parent of Raphanobrassica (Figure 1C). This would enable the breeding of Raphanobrassica cultivars with high 4MSOB content in the leaves. In this study, allodiploid Raphanobrassica (2n = 2x = 18, RC) plants were produced from a cross between kale and radish with heterozygous GRS1, and the correlation between GRS1 genotype and 4MSOB content was analyzed.
2 Methods
2.1 Plant material and growth conditions
A previous study reported a Japanese radish (Raphanus sativus L.) landrace, ‘cv. Nishimachi-Riso’, which contains a GRS1 mutation (Ishida et al., 2015). As the genotype of GRS1 was not fixed within the ‘cv. Nishimachi-Riso’ population, the genotype of GRS1 was determined using DNA markers (see 2.2 Genotyping) and plants carrying heterozygouse GRS1 (GRS1/grs1) were designated as AKO lines. Kale (Brassica oleracea var. acephala) inbred line ‘KK45-2’ is a collard-type kale whose leaves contain 4MSOB used as the paternal parent. All the plants were grown in plastic pots (diameter of 210 mm) in a greenhouse. A Nippi-engei-baido (Nihon Hiryo Co.,Ltd, Gunma, Japan) based soil was used and 1/1000 HYPONeX (HYPONeX JAPAN CORP., LTD., Osaka, Japan) solution was applied once a week. To obtain hybrids, the anthers were removed from radish buds 1–3 d before flowering and were pollinated with kale pollen on the day of flowering. Raphanobrassica seeds were sown on September 5, 2020, and genomic DNA was purified from the true leaves two weeks later and used for genotyping. For genomic DNA purification, DNeasy 96 Plant Kit (QIAGEN, Venlo, Netherlands) was used. The plants were then planted in a field on August 31, 2020, with a gap of 35 cm between plants and 60 cm between rows, at the Institute of Vegetable and Floriculture Science (34°46′N, 136°25′E; Tsu, Mie, Japan).
2.2 Genotyping
Previous studies have shown that insertion into the first exon of GRS1 causes complete functional defects (Kakizaki et al., 2017). To detect the insertion into GRS1, primers were designed at the genomic positions shown in Figure 2A, and polymerase chain reaction (PCR) was used to amplify the region between Rs270 (5′-GCAGGAGAGGATGCTTGAAGG-3′) and Rs271 (5′-TGAAACCTTACCCCAAAACG-3′) for the functional type, Rs270 and Rs272 (5′-TCCAGGTTGGGATAGCTTGT-3′) for the defective type. PCR was performed under the following conditions: initial denaturation at 94°C for 1 min, cycling at 94°C for 15 s for heat denaturation, annealing at 60°C for 15 s, and extension at 72°C for 50 s for 32 cycles. The amplified PCR products were separated on a 2% agarose gel, and the functional and defective types were distinguished based on differences in fragment length (Figure 2B). To confirm that the kale genome was inherited by the hybrid, PCR was performed using primers Bo-Fw (5′-CTAGTATGAGGACTCGTTCAGTTACCTCCCTTAGCAGC-3′) and Bo-Rv (5′-GTTTCTTAGAATATGGTGATTGCTGGCTT-3′) to amplify UDP-sulfoquinovose synthase (LOC106306866), which is located on chromosome C1.
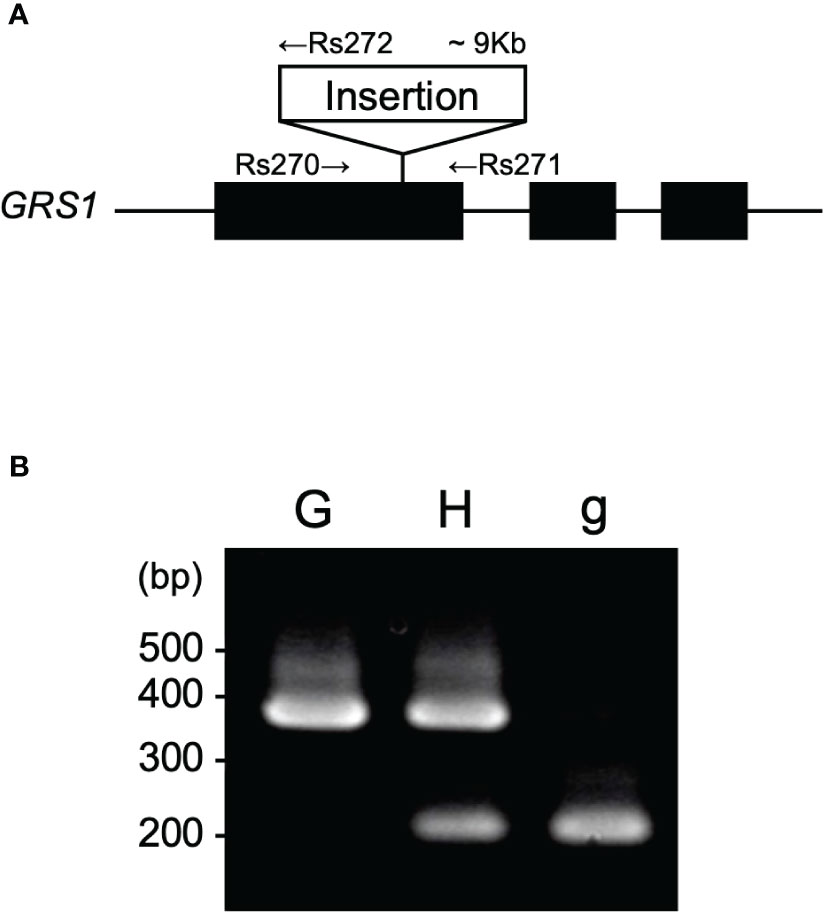
Figure 2 (A) Gene structure of GRS1. Black boxes represent exons. Arrows represent the position of primers for PCR. (B) Representative agarose gel pattern of amplified PCR product. “G”, “H”, and “g” indicate homozygote for GRS1, heterozygote, and homozygote for grs1, respectively. GRS1, GLUCORAPHASATIN SYNTHASE 1; PCR, polymerase chain reaction.
2.3 GSL analysis
Leaf, root, stem, and flower bud are used for GSL analysis (Figure 3). For leaf analysis, 10 cm from the tip of the 20 cm long leaf was collected, and the central vein was removed. Three leaves per plant were collected and analyzed as one bulk sample. For root analysis, a section was cut 5 cm below the stem/hypocotyl border in the form of a disk (thickness, 0.5–1.0 cm) and collected. For bud analysis, apical flower buds were collected. For stem analysis, a 10 cm section below the apical flower bud of the main stem, which was elongated after bolting, was collected.
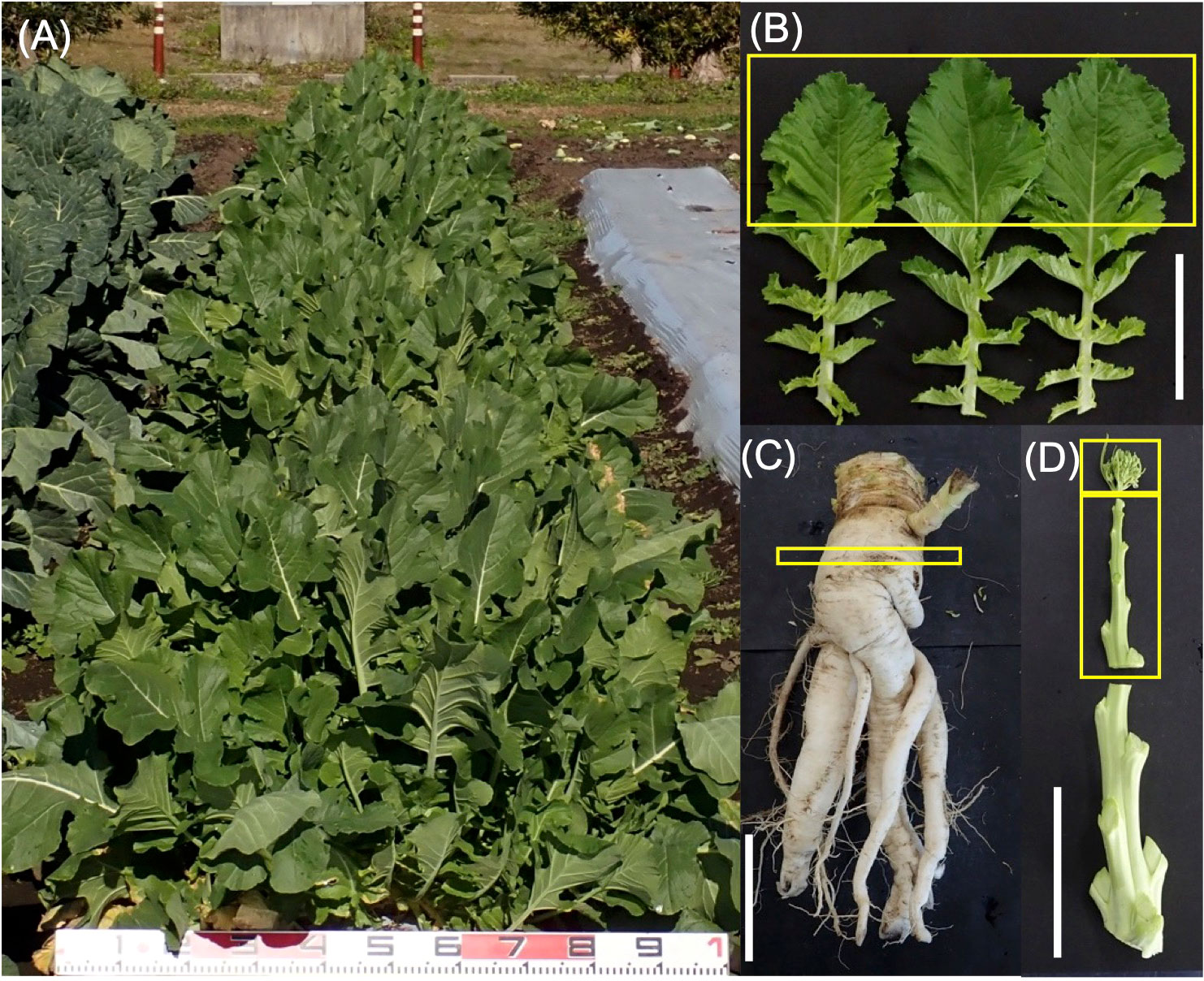
Figure 3 (A) Typical phenotypic characteristics of Raphanobrassica (A). Plant parts used for glucosinolate analysis: (B) leaf, (C) root, (D) flower bud, and stem. Yellow boxes show a part used for high-performance liquid chromatography analysis. White bars indicate 10 cm.
After sampling, each part was frozen in liquid nitrogen and dried using a lyophilizer (Labconco, Kansas City, MO, USA). The dried samples were crushed using a multi-bead shocker (Yasui Machinery, Miyazaki, Japan); 0.1 g of ground samples were weighed, mixed with 4.8 mL of 80% methanol and 0.2 mL of 5 mM 2-propenyl GSL (Sigma-Aldrich, St. Louis, MO, USA) as an internal standard and shaken for 30 min at 25°C. After centrifugation at 3,000 rpm for 10 min, the supernatant was collected, GSLs were adsorbed onto DEAE-Sephadex A-25 (Sigma-Aldrich, St. Louis, MO, USA) and desulfonated using arylsulfatase (Type H-1, EC 3.1.6.1, Sigma-Aldrich, St. Louis, MO, USA) at 25°C for 18 h. Desulfo-GSL solutions eluted ion-exchange water were used as samples for high-performance liquid chromatography (HPLC) analysis (LC-20A; Shimadzu Corp., Kyoto, Japan). A reverse-phase column (COSMOSIL 5C18-II, 150 × 4.6 mm; Nacalai Tesque Inc., Kyoto, Japan) was used at 30°C and a flow rate of 1.5 mL/min. The mobile phase comprised 20% acetonitrile, and detection was performed using UV light with a wavelength of 229 nm. The GSL molecular species were estimated based on the retention time of the peaks according to our previous report (Ishida et al., 2015). The individual GSL contents were calculated by the ratios of the individual desulfo-GSL peak areas to the peak areas of an internal standard, 2-propenyl GSL (Sigma-Aldrich, St. Louis, MO, USA), and a response factor (The International Organization for Standardization, 1992). Table 1 shows the list of GSLs analyzed, and Figure 4 shows representative chromatograms of each desulfo-GSL molecular species detected using HPLC.
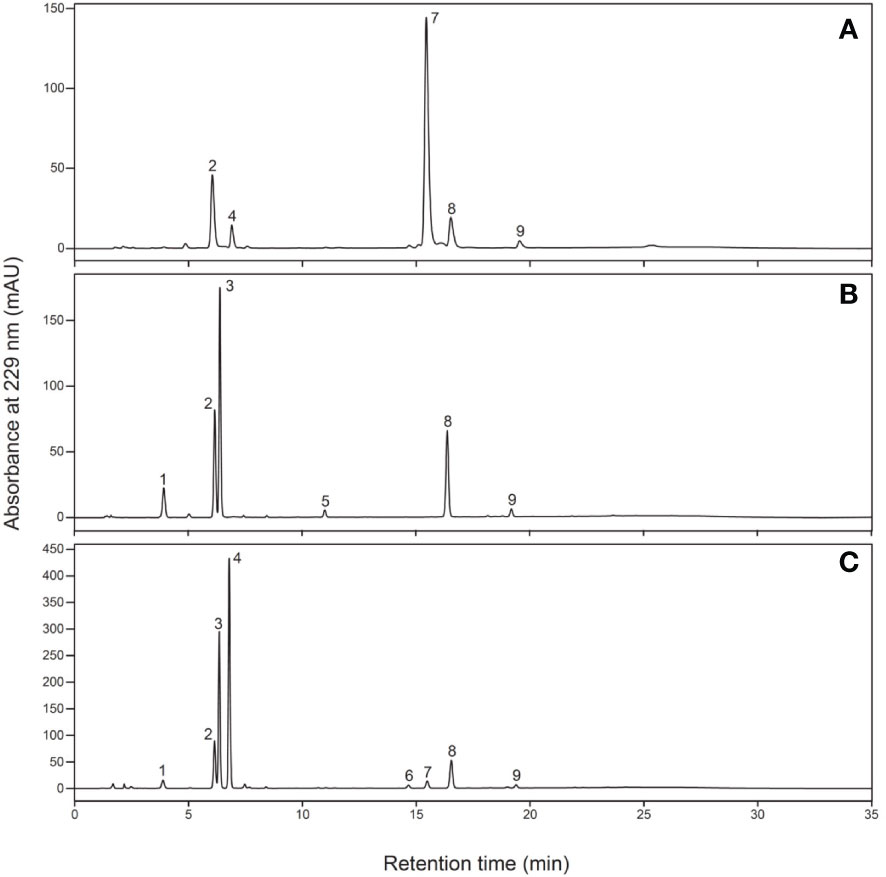
Figure 4 Typical chromatograms of desulfo-glucosinolates in (A) radish, (B) kale, and (C) Raphanobrassica. Peak 1, 3-(methylthio)propyl; peak 2, 2-propenyl (internal standard); peak 3, 4-(methylsulfinyl)butyl; peak 4, 4-methylsulfinyl-3-butenyl; peak 5, 4-methoxyindol-3-ylmethyl; peak 6, 4-(methylthio)butyl; peak 7, 4-methylthio-3-butenyl; peak 8, indol-3-ylmethyl; peak 9, 4-methoxyindol-3-ylmethyl.
3 Results
3.1 Intergeneric cross between radish and kale
To generate intergeneric hybrids that harbored the grs1 allele, we used a radish AKO line that was heterozygous for the GRS1 gene (GRS1/grs1) as a seed parent. The kale KK45-2 line, containing 4MSOB in mature leaves, was used as the pollen parent. At the time of crossing, all stamens were removed from radish buds 1–2 d before flowering to avoid self-fertilization, and pollination was performed using kale pollen on the day of flowering. Twenty-one AKO plants were pollinated using KK45-2 pollen, and the pod formation rate varied from 0 to 0.24 among radish plants (Table 2). Similarly, there was a difference in the number of seeds per pod among the AKO plants. Among the 21 combinations, the seeds of four radish plants (AKO103, AKO108, AKO110, and AKO118) that yielded a large number of seeds were sown in petri dishes, and their germination rates and genotypes were investigated (Table 3). The germination percentage ranged from 71.4–97.1%, and 47–87 plants were obtained from each combination. To confirm that the obtained plants were hybrids, PCR was performed using primers specific for kale UDP-sulfoquinovose synthase (LOC106306866). This confirmed the inheritance of the kale genome. No amplification of the kale genome was detected in only four plants obtained from AKO110, and these plants were inbred radish plants. Genotyping using the GRS1 marker (Figure 2) resulted in a 1:1 match for GRS1 segregation in all combinations. No plants heterozygous for the GRS1 marker were identified. Based on these results, we obtained hybrids of radish and kale, and GRS1 segregation followed theoretical values. These data allowed us to evaluate the relationship between the GRS1 genotype and 4MSOB quantity in the Raphanobrassica population.
3.2 Suppression of GRS1 function increased 4MSOB content in the intergeneric hybrid
The obtained hybrids were planted in a field on September 5, 2020, and the GSL composition in the true leaves (20 cm length) was analyzed 72 d after planting. Growth was vigorous, and leaf shape was similar to that of radish (Figures 3A, B). No plants with pollen fertility were observed for any of the cross combinations. The root shape was snarled and the main root branched into several branches (Figure 3C). The timing of bolting was earlier than that of the parents, and the shape of the flower buds was similar to that of kale (Figure 3D). The 4MSOB content in the true leaves of intergeneric hybrids and parent plants is shown in Figure 5. Notably, in all cross combinations, the grs1-type had a 4MSOB content approximately twice as high as that of functional GRS1 hybrids (Table 4). Furthermore, these grs1-type plants had higher 4MSOB concentrations than those in kale KK45-2 and radish AKO103 (grs1/grs1) plants. There were significant differences in the concentration of 4MSOB between GRS1 genotypes, but even within the same GRS1 genotype the concentration of 4MSOB varied widely (Figure 5). The average of 4MSOB in four lines in GRS1-type and grs1-type were 15.5 ± 0.5 and 34.1 ± 1.0 µmol·g-1 dry weight in leaves, respectively. The highest 4MSOB concentration was 69.4 µmol·g-1 dry weight. In grs1-type Raphanobrassica, 4MSO3B was almost undetectable and the accumulation of 4MTB was detected (Figure 6). The contents of indolic GSLs, such as Indol-3-ylmethyl GSL (glucobrassicin, I3M), were not affected by the GRS1 mutation.
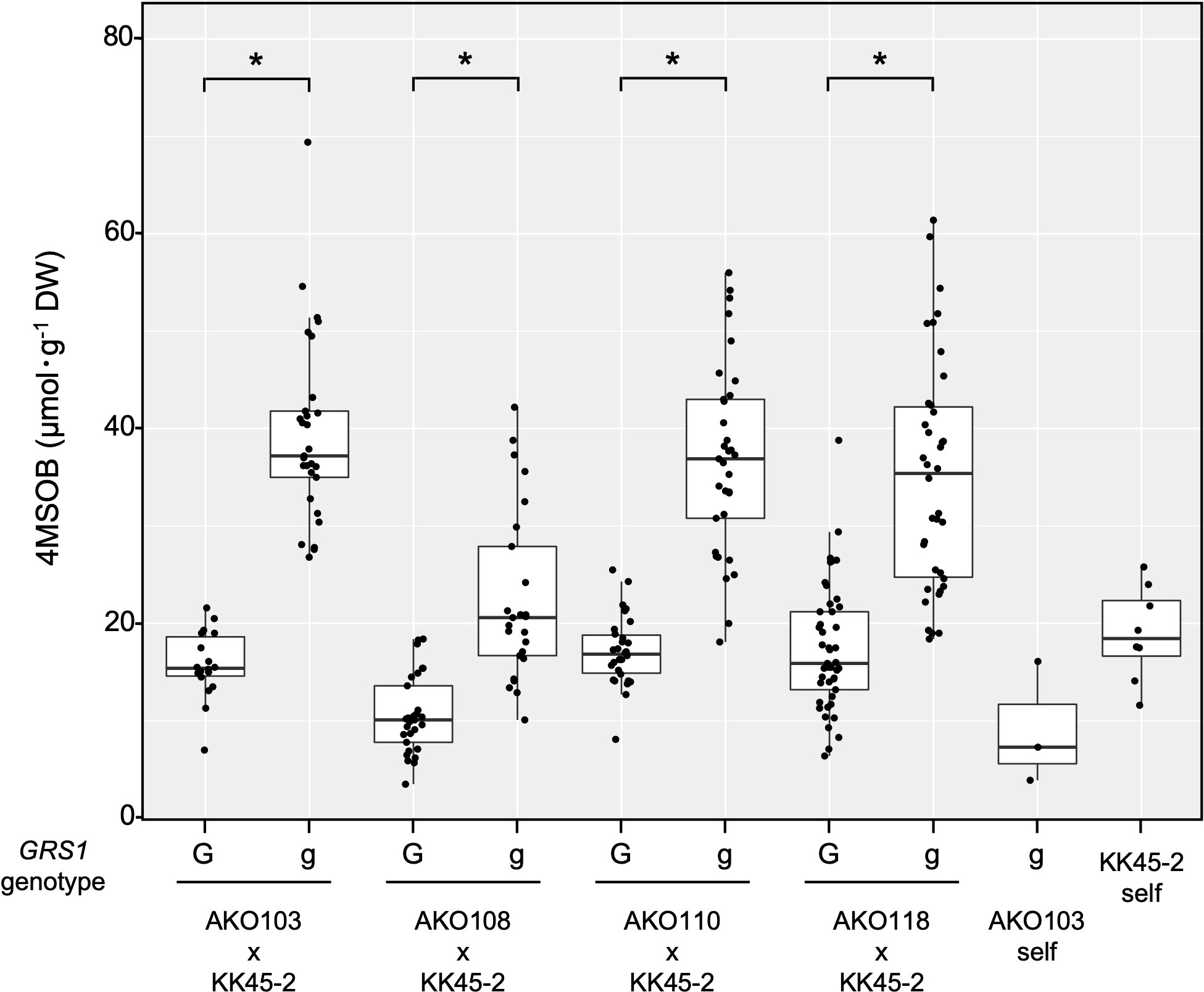
Figure 5 Distribution of leaf 4SMOB content in intergeneric hybrids and parent lines. The genotype of GRS1 is indicated by “G” for functional and “g” for null allele. Asterisks above the bars indicate significant differences (p < 0.01) between the genotypes of GRS1 as determined via the Welch’s t-test. GRS1, GLUCORAPHASATIN SYNTHASE 1.
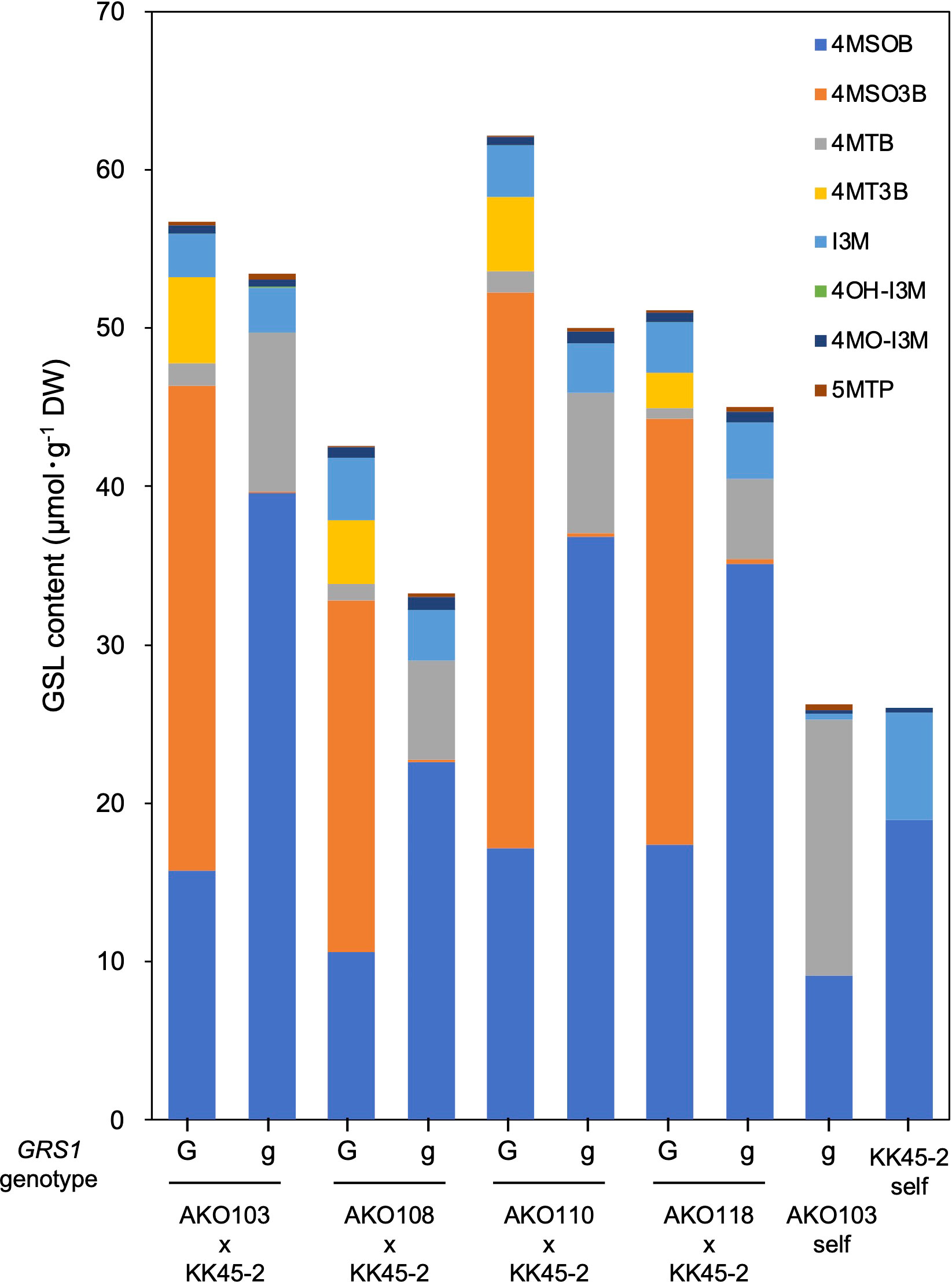
Figure 6 GSL composition and content in Raphanobrassica leaf. The genotype of GRS1 is indicated by “G” for functional and “g” for null allele. GRS1, GLUCORAPHASATIN SYNTHASE 1; GSL, glucosinolate. 4MSOB, 4-(methylsulfinyl)butyl GSL; 4MSO3B, 4-methylsulfinyl-3-butenyl GSL; 4MTB, 4-(methylthio)butyl GSL; 4MT3B, 4-methylthio-3-butenyl GSL; I3M, indol-3-ylmethyl GSL; 4OH-I3M, 4-methoxyindol-3-ylmethyl GSL, 4MO-I3M, 4-methoxyindol-3-ylmethyl GSL; 5MTP, 5-(methylthio)pentyl GSL.
3.3 GSL content in roots, flower buds, and stems was affected by GRS1 function
The grs1 mutation increased 4MSOB concentration by approximately 2-fold in the leaves of the hybrids. Next, we analyzed the GSL profiles in various edible plant parts such as roots, flower buds, and stems (Table 5). The 4MSOB content was highest in the flower buds, followed by stems and roots. The grs1 mutation increased the concentration of 4MSOB by more than 2-fold in flower buds and stems, similar to that in leaves. The major GSLs in the roots were 4MT3B and 4MTB, whereas those in the flower buds and stems were 4MSO3B and 4MSOB (Figure 7). 2-Hydroxy-3-butenyl GSL (progoitrin, 2H3B) and I3M, which are rarely detected in the roots, were detected in flower buds and stems. Regarding the total GSL, the GRS1-type plants had a higher content than that in the grs1-type, similar to that in the leaves. The total GSL content was highest in flower buds for both GRS1 and grs1-types, followed by roots and stems.
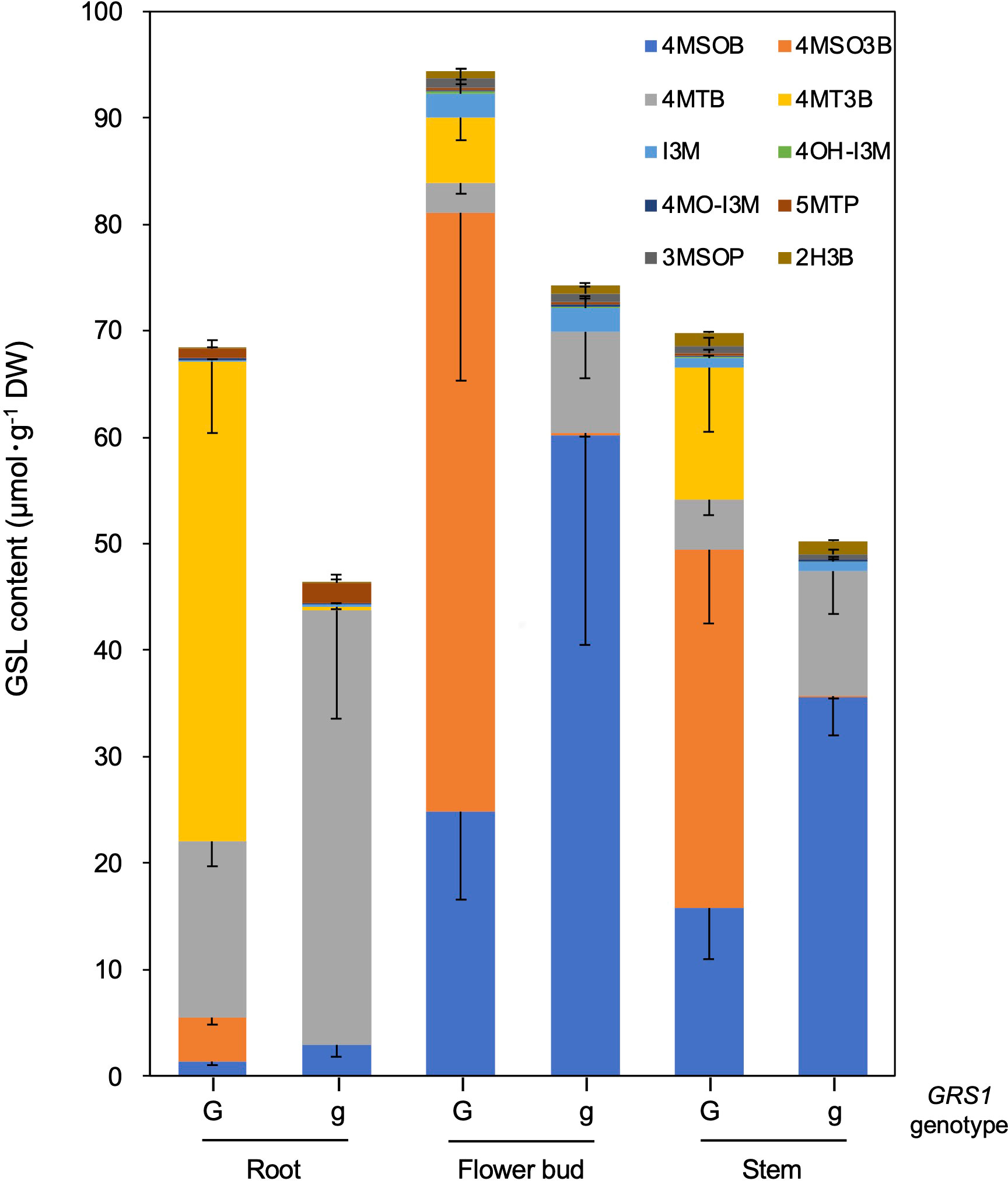
Figure 7 GSL composition and content in root, flower bud, and stem in Raphanobrassica. The genotype of GRS1 is indicated by “G” for functional and “g” for null allele. Error bars represent standard deviation. GRS1, GLUCORAPHASATIN SYNTHASE 1; GSL, glucosinolate. 4MSOB, 4-(methylsulfinyl)butyl GSL; 4MSO3B, 4-methylsulfinyl-3-butenyl GSL; 4MTB, 4-(methylthio)butyl GSL; 4MT3B, 4-methylthio-3-butenyl GSL; I3M, indol-3-ylmethyl GSL; 4OH-I3M, 4-methoxyindol-3-ylmethyl GSL, 4MO-I3M, 4-methoxyindol-3-ylmethyl GSL; 5MTP, 5-(methylthio)pentyl GSL; 3MSOP, 3-(methythio)propyl GSL; 2H3B, 2-hydroxy-3-butenyl GSL.
4 Discussion
4.1 An intergeneric hybrid between radish and kale
Raphanobrassica, an intergeneric hybrid between the genera Raphanus and Brassica, contains large amounts of 4MSOB and 4MSO3B (Schutze et al., 1999). Lyophilized powder derived from Raphanobrassica inhibits Helicobacter pylori-induced gastritis in Mongolian gerbils (Yamada et al., 2014). Although Raphanobrassica has useful properties, the hybridization rate between Raphanus and Brassica is considerably low. In 1973, McNaughton reported that the number of seeds per pollinated flower between R. sativus and B. oleracea is between 0.38 and 0.4 (McNaughton, 1973). This value is comparable to that of the most efficient combination in the crossing experiment of the present study (AKO118 × KK45-2, 0.34). Further, the formation rates of hybrids differ between varieties (Kakizaki, 1925). This phenomenon is known as the “hybridization barrier” and is divided into two types: pre-zygotic and post-zygotic barriers. The pre-zygotic barrier is caused mainly by defects in fertilization, such as interspecific incompatibility and defects in pollen tube guidance (Dresselhaus and Marton, 2009). The post-zygotic barrier includes hybrid embryo breakdown and hybrid sterility. Candidate genes or quantitative trait loci responsible for these barriers have been cloned (Udagawa et al., 2010; Tonosaki et al., 2013). In the present study, the hybridization rates were markedly different between AKO plants originating from the same radish ‘cv. Nishimachi-Riso’ (Table 2). It is not known whether the barriers observed in the present study are pre- or post-zygotic, but differences in their degree within the same species may provide good material for genetic analysis.
4.2 GSLs in Raphanobrassica
The hybridization between a radish and Chinese cabbage produces a cultivar called ‘Baemoochae’ (Lee et al., 2011). Baemoochae contains GSL molecular species present in both radish and Chinese cabbage. However, the total GSL content is similar to that of both parents (Nugroho et al., 2020). In contrast, the total GSL content of Raphanobrassica produced in this study was higher than that of either parent, suggesting that the entire GSL synthesis pathway was activated. It is reported that the GSL synthesis gene expression is elevated in resynthesized Brassica allotetraploids compared with that in their diploids (Zhang et al., 2015). Further studies are needed to determine whether the increased 4MSOB in Raphanobrassica developed in this study is solely due to mutations in GRS1 or is also influenced by the increased expression of other biosynthetic enzyme genes. GSLs are hydrolyzed by myrosinases to not only ITCs but other products (thiocyanates, nitriles, or epithionitriles) depending on pH and the presence of epithiospecifier protein (Bones and Rossiter, 1996). Distribution of degradated products vary widely between plant species. B. oleracea has a higher proportion of nitriles and epithionitriles than ITCs, whereas R. sativus has a higher proportion of ITCs (Cole, 1976). Therefore, analysis of the abundance ratios of degradation products in Raphanobrassica, which has both genomes, is of great importance for its use as a functional vegetable.
4.3 Effects of grs1 mutation on the GSL synthesis pathway
Hybrids containing grs1 showed almost no 4MT3B or 4MSO3B content in their leaves (Table 4 and Figure 6). This result supports the hypothesis that the introduction of grs1 into Raphanobrassica enhances 4MTB utilization in 4MSOB synthesis and increases the 4MSOB content. However, in all cross combinations, the total content of each GSL in the population possessing grs1-type tended to be lower than that in GRS1-type plants (Figure 6). Therefore, increased 4MSOB content in plants lacking GRS1 function may cause feedback inhibition of FMO. For example, YUCCA, an enzyme in the auxin synthesis pathway, also belongs to the monooxygenase family, similar to FMO. YUCCA transcription levels are negatively regulated by the synthetic product auxin (Suzuki et al., 2015). If FMO activity and transcription levels are negatively regulated by 4MSOB, understanding the underlying mechanisms and applying them in breeding could lead to higher 4MSOB content.
4.4 Potential and challenges of using Raphanobrassica as a high-4MSOB containing vegetable
4MSOB is present in the genera Brassica, Eruca, and Raphanus (Ciska et al., 2000; Ishida et al., 2014). A study in 1992 reported that sulforaphane (ITC derived from 4MSOB) in broccoli functions as a major inducer of anti-carcinogenic defense enzymes. Consequently, the recognition of broccoli as a representative vegetable containing 4MSOB has increased, which has prompted research in various fields. To develop broccoli varieties with high 4MSOB content, MYB28 of the wild species B. villosa (BvMYB28) has been introduced into cultivated species, and the F1 hybrid Beneforté® has been cultivated (Traka et al., 2013). In Arabidopsis, AtMYB28 positively regulates aliphatic GSL biosynthesis (Sonderby et al., 2007). The expression of several aliphatic GSL genes is elevated in broccoli, which is homozygous for BvMYB28. The 4MSOB concentration in the homozygous BvMYB28 inbred broccoli line is 20 µmol·g-1 dry weight in floret (Neequaye et al., 2022). In contrast, the average value of 4MSOB in the leaves of Raphanobrassica with grs1 grown in this study was 34.1 µmol·g-1 dry weight (Table 4). Although simple comparisons cannot be made because the analyzed sites contained the highest contents in adult plants and the content varies with the growing region and cultivation method, the variety with defective GRS1 in Raphanobrassica may be used in various cuisines as a new leafy vegetable that contains as much 4MSOB in broccoli Beneforté®. Compared with kale, the taste of Raphanobrassica leaves is softer and less gruel-like, making it suitable for a variety of dishes such as stir-fry and salads. The low hybridization affinity between radish and kale and the inability to ensure a commercial level of seed production represent issues that need to be addressed to popularize the variety in the future. However, chromosome doubling may be used to breed seed-fertile allotetraploid Raphanobrassica (Niimi et al., 2015).
Data availability statement
The original contributions presented in the study are included in the article/Supplementary Material, further inquiries can be directed to the corresponding author/s.
Author contributions
TK and HC supervised and conceived the project. RE and TK wrote the manuscript. EI, MK, and TO supported the experiments and revised the manuscript. TK and RE cultivated the plants and evaluated the GSLs. All authors contributed to the article and approved the submitted version.
Funding
Research funding for this study was provided in part by KAGOME CO., LTD.
Acknowledgments
We thank Y. Kawamoto, S. Morimoto, E. Yamamoto, H. Saito, and M. Kitazumi for technical assistance. We would like to thank Editage (www.editage.com) for English language editing.
Conflict of interest
KAGOME CO., LTD. Nagoya, Japan supported this work and provided support in the form of salaries to RE and HC.
The remaining authors declare that the research was conducted in the absence of any commercial or financial relationships that could be construed as a potential conflict of interest.
Publisher’s note
All claims expressed in this article are solely those of the authors and do not necessarily represent those of their affiliated organizations, or those of the publisher, the editors and the reviewers. Any product that may be evaluated in this article, or claim that may be made by its manufacturer, is not guaranteed or endorsed by the publisher.
Supplementary material
The Supplementary Material for this article can be found online at: https://www.frontiersin.org/articles/10.3389/fpls.2023.1132302/full#supplementary-material
References
Bones, A. M., Rossiter, J. T. (1996). The myrosinase-glucosinolate system, its organisation and biochemistry. Physiol. Plant. 97, 194–208. doi: 10.1111/j.1399-3054.1996.tb00497.x
Cartea, M. E., Velasco, P. (2007). Glucosinolates in brassica foods: bioavailability in food and significance for human health. Phytochem. Rev. 7, 213–229. doi: 10.1007/s11101-007-9072-2
Chen, Q. M., Maltagliati, A. J. (2018). Nrf2 at the heart of oxidative stress and cardiac protection. Physiol. Genomics 50, 77–97. doi: 10.1152/physiolgenomics.00041.2017
Ciska, E., Martyniak-Przybyszewska, B., Kozlowska, H. (2000). Content of glucosinolates in cruciferous vegetables grown at the same site for two years under different climatic conditions. J. Agric. Food Chem. 48, 2862–2867. doi: 10.1021/jf981373a
Cole, R. A. (1976). Isothiocyanates, nitriles and thiocyanates as products of autolysis of glucosinolates in Cruciferae. Phytochemistry 15, 759–762. doi: 10.1016/S0031-9422(00)94437-6
Dresselhaus, T., Marton, M. L. (2009). Micropylar pollen tube guidance and burst: adapted from defense mechanisms? Curr. Opin. Plant Biol. 12, 773–780. doi: 10.1016/j.pbi.2009.09.015
Egner, P. A., Chen, J. G., Zarth, A. T., Ng, D. K., Wang, J. B., Kensler, K. H., et al. (2014). Rapid and sustainable detoxication of airborne pollutants by broccoli sprout beverage: results of a randomized clinical trial in China. Cancer Prev. Res. (Phila) 7, 813–823. doi: 10.1158/1940-6207.CAPR-14-0103
Fahey, J. W., Zalcmann, A. T., Talalay, P. (2001). The chemical diversity and distribution of glucosinolates and isothiocyanates among plants. Phytochemistry 56, 5–51. doi: 10.1016/S0031-9422(00)00316-2
Farnham, M. W., Wilson, P. E., Stephenson, K. K., Fahey, J. W. (2004). Genetic and environmental effects on glucosinolate content and chemoprotective potency of broccoli. Plant Breed. 123, 60–65. doi: 10.1046/j.0179-9541.2003.00912.x
Halkier, B. A., Gershenzon, J. (2006). Biology and biochemistry of glucosinolates. Annu. Rev. Plant Biol. 57, 303–333. doi: 10.1146/annurev.arplant.57.032905.105228
Ishida, M., Hara, M., Fukino, N., Kakizaki, T., Morimitsu, Y. (2014). Glucosinolate metabolism, functionality and breeding for the improvement of brassicaceae vegetables. Breed Sci. 64, 48–59. doi: 10.1270/jsbbs.64.48
Ishida, M., Kakizaki, T., Morimitsu, Y., Ohara, T., Hatakeyama, K., Yoshiaki, H., et al. (2015). Novel glucosinolate composition lacking 4-methylthio-3-butenyl glucosinolate in Japanese white radish (Raphanus sativus l.). Theor. Appl. Genet. 128, 2037–2046. doi: 10.1007/s00122-015-2564-3
Kakizaki, Y. (1925). A preliminary report of crossing experiments with cruciferous plants, with special reference to sexual compatibility and matroclinous hybrids. Japanese J. Genet. 3, 49–82. doi: 10.1266/jjg.3.49
Kakizaki, T., Kitashiba, H., Zou, Z., Li, F., Fukino, N., Ohara, T., et al. (2017). A 2-Oxoglutarate-Dependent dioxygenase mediates the biosynthesis of glucoraphasatin in radish. Plant Physiol. 173, 1583–1593. doi: 10.1104/pp.16.01814
Karpechenko, G. D. (1924). Hybrids of female Raphanus sativus l x male Brassica oleracea l. J. Genet. 14, 375–396. doi: 10.1007/BF02983104
Kensler, T. W., Chen, J. G., Egner, P. A., Fahey, J. W., Jacobson, L. P., Stephenson, K. K., et al. (2005). Effects of glucosinolate-rich broccoli sprouts on urinary levels of aflatoxin-DNA adducts and phenanthrene tetraols in a randomized clinical trial in he zuo township, qidong, people's republic of China. Cancer Epidemiol. Biomarkers Prev. 14, 2605–2613. doi: 10.1158/1055-9965.EPI-05-0368
Kensler, T. W., Egner, P. A., Agyeman, A. S., Visvanathan, K., Groopman, J. D., Chen, J. G., et al. (2013). Keap1-nrf2 signaling: a target for cancer prevention by sulforaphane. Top. Curr. Chem. 329, 163–177. doi: 10.1007/128_2012_339
Kikuchi, M., Ushida, Y., Shiozawa, H., Umeda, R., Tsuruya, K., Aoki, Y., et al. (2015). Sulforaphane-rich broccoli sprout extract improves hepatic abnormalities in male subjects. World J. Gastroenterol. 21, 12457–12467. doi: 10.3748/wjg.v21.i43.12457
Lee, S. S., Lee, S. A., Yang, J., Kim, J. (2011). Developing stable progenies of xBrassicoraphanus, an intergeneric allopolyploid between Brassica rapa and Raphanus sativus, through induced mutation using microspore culture. Theor. Appl. Genet. 122, 885–891. doi: 10.1007/s00122-010-1494-3
McNaughton, I. H. (1973). Synthesis and sterility of raphanobrassica. Euphytica 22, 70–88. doi: 10.1007/BF00021558
Neequaye, M., Steuernagel, B., Saha, S., Trick, M., Troncoso-Rey, P., Van Den Bosch, F., et al. (2022). Characterisation of the introgression of Brassica villosa genome into broccoli to enhance methionine-derived glucosinolates and associated health benefits. Front. Plant Sci. 13, 855707. doi: 10.3389/fpls.2022.855707
Niimi, H., Watanabe, M., Serizawa, H., Koba, T., Nakamura, I., Mii, M. (2015). Amiprophosmethyl-induced efficient in vitro production of polyploids in raphanobrassica with the aid of aminoethoxyvinylglycine (AVG) in the culture medium. Breed Sci. 65, 396–402. doi: 10.1270/jsbbs.65.396
Nouchi, R., Hu, Q. Q., Saito, T., Kawata, N. Y. D., Nouchi, H., Kawashima, R. (2021). Brain training and sulforaphane intake interventions separately improve cognitive performance in healthy older adults, whereas a combination of these interventions does not have more beneficial effects: evidence from a randomized controlled trial. Nutrients 13, 352. doi: 10.3390/nu13020352
Nugroho, A. B. D., Han, N., Pervitasari, A. N., Kim, D. H., Kim, J. (2020). Differential expression of major genes involved in the biosynthesis of aliphatic glucosinolates in intergeneric baemoochae (Brassicaceae) and its parents during development. Plant Mol. Biol. 102, 171–184. doi: 10.1007/s11103-019-00939-2
Rask, L., Andreasson, E., Ekbom, B., Eriksson, S., Pontoppidan, B., Meijer, J. (2000). Myrosinase: gene family evolution and herbivore defense in Brassicaceae. Plant Mol. Biol. 42, 93–113. doi: 10.1023/A:1006380021658
Riedl, M. A., Saxon, A., Diaz-Sanchez, D. (2009). Oral sulforaphane increases phase II antioxidant enzymes in the human upper airway. Clin. Immunol. 130, 244–251. doi: 10.1016/j.clim.2008.10.007
Schutze, W., Mandel, F., Schulz, H. (1999). Identification of glucosinolates in radish (Raphanus sativus l.) and cross-breeds of R. sativus l. x Brassica oleracea l. (Raphanobrassica) by LC-MS. Nahrung-Food 43, 245–248. doi: 10.1002/(SICI)1521-3803(19990801)43:4<245::AID-FOOD245>3.0.CO;2-#
Sonderby, I. E., Hansen, B. G., Bjarnholt, N., Ticconi, C., Halkier, B. A., Kliebenstein, D. J. (2007). A systems biology approach identifies a R2R3 MYB gene subfamily with distinct and overlapping functions in regulation of aliphatic glucosinolates. PLoS One 2, e1322. doi: 10.1371/journal.pone.0001322
Suzuki, M., Yamazaki, C., Mitsui, M., Kakei, Y., Mitani, Y., Nakamura, A., et al. (2015). Transcriptional feedback regulation of YUCCA genes in response to auxin levels in Arabidopsis. Plant Cell Rep. 34, 1343–1352. doi: 10.1007/s00299-015-1791-z
The International Organization for Standardization (1992). Rapeseed- determination of glucosinolate content. ISO 9167–1, 1–9.
Tonosaki, K., Michiba, K., Bang, S. W., Kitashiba, H., Kaneko, Y., Nishio, T. (2013). Genetic analysis of hybrid seed formation ability of Brassica rapa in intergeneric crossings with Raphanus sativus. Theor. Appl. Genet. 126, 837–846. doi: 10.1007/s00122-012-2021-5
Traka, M. H., Saha, S., Huseby, S., Kopriva, S., Walley, P. G., Barker, G. C., et al. (2013). Genetic regulation of glucoraphanin accumulation in Beneforté® broccoli. New Phytol. 198, 1085–1095. doi: 10.1111/nph.12232
Udagawa, H., Ishimaru, Y., Li, F., Sato, Y., Kitashiba, H., Nishio, T. (2010). Genetic analysis of interspecific incompatibility in Brassica rapa. Theor. Appl. Genet. 121, 689–696. doi: 10.1007/s00122-010-1340-7
Wermter, N. S., Rohn, S., Hanschen, F. S. (2020). Seasonal variation of glucosinolate hydrolysis products in commercial white and red cabbages (Brassica oleracea var. capitata). Foods 9, 1682. doi: 10.3390/foods9111682
Yagishita, Y., Fahey, J. W., Dinkova-Kostova, A. T., Kensler, T. W. (2019). Broccoli or sulforaphane: is it the source or dose that matters? Molecules 24, 3593. doi: 10.3390/molecules24193593
Yamada, T., Wei, M., Toyoda, T., Yamano, S., Wanibuchi, H. (2014). Inhibitory effect of raphanobrassica on helicobacter pylori-induced gastritis in Mongolian gerbils. Food Chem. Toxicol. 70, 107–113. doi: 10.1016/j.fct.2014.04.037
Yuanfeng, W., Chengzhi, L., Ligen, Z., Juan, S., Xinjie, S., Yao, Z., et al. (2021). Approaches for enhancing the stability and formation of sulforaphane. Food Chem. 345, 128771. doi: 10.1016/j.foodchem.2020.128771
Keywords: glucosinolate, radish, kale, intergeneric hybrid, Raphanobrassica, GLUCORAPHASATIN SYNTHASE 1, sulforaphane, glucoraphanin
Citation: Endo R, Chikano H, Itabashi E, Kawasaki M, Ohara T and Kakizaki T (2023) Large insertion in radish GRS1 enhances glucoraphanin content in intergeneric hybrids, Raphanobrassica (Raphanus sativus L. x Brassica oleracea var. acephala). Front. Plant Sci. 14:1132302. doi: 10.3389/fpls.2023.1132302
Received: 27 December 2022; Accepted: 05 April 2023;
Published: 06 June 2023.
Edited by:
Xiangshu Dong, Yunnan University, ChinaReviewed by:
Zhansheng Li, Insititute of Vegetables and Flowers (CAAS), ChinaPrabhakaran Soundararajan, National Institute of Plant Genome Research (NIPGR), India
Franziska S. Hanschen, Leibniz Institute of Vegetable and Ornamental Crops, Germany
Copyright © 2023 Endo, Chikano, Itabashi, Kawasaki, Ohara and Kakizaki. This is an open-access article distributed under the terms of the Creative Commons Attribution License (CC BY). The use, distribution or reproduction in other forums is permitted, provided the original author(s) and the copyright owner(s) are credited and that the original publication in this journal is cited, in accordance with accepted academic practice. No use, distribution or reproduction is permitted which does not comply with these terms.
*Correspondence: Tomohiro Kakizaki, dGtha2lAYWZmcmMuZ28uanA=