- 1Department of Clinical Microbiology and Immunology Faculty of Medicine, King AbdulAziz University, Jeddah, Saudi Arabia
- 2Special Infectious Agents Unit, King Fahad Medical Research Center, King AbdulAziz University, Jeddah, Saudi Arabia
- 3Department of Medical Laboratory Sciences, Faculty of Applied Medical Sciences, King Abdulaziz University, Jeddah, Saudi Arabia
- 4Department of Medical Microbiology and Parasitology, Faculty of Medicine, University of Jeddah, Jeddah, Saudi Arabia
- 5Department of Hematology/Oncology, Yousef Abdulatif Jameel Scientific Chair of Prophetic Medicine Application, Faculty of Medicine, King Abdulaziz University, Jeddah, Saudi Arabia
- 6Department of Clinical Laboratory Sciences, College of Applied Medical Sciences, Jouf University, Sakaka, Saudi Arabia
- 7Department of Botany and Microbiology, Faculty of Science, Beni-Suef University, Beni-Suef, Egypt
Introduction: Medicinal plants have been considered as potential source of therapeutics or as starting materials in drugs formulation.
Methods: The current study aims to shed light on the therapeutic potential of the Amomum subulatom and Amomum xanthioides Fruits by analyzing the phytochemical composition of their seeds and fruits using gas chromatography-mass spectrometry (GC-MS) and high-performance liquid chromatography (HPLC) techniques to determine the presence of bioactive components such as flavonoids, phenols, vitamins, steroids, and essential oils.
Results and Discussion: The protein content is usually higher than the total lipids in both species except the fruit of A. subulatum which contain more lipids than proteins. The total protein contents for A. subulatum were 235.03 ± 21.49 and 227.49 ± 25.82 mg/g dry weight while for A. xanthioides were 201.9 ± 37.79 and 294.99 ± 37.93 mg/g dry weight for seeds and fruit, respectively. The Carvacrol levels in A. subulatum is 20 times higher than that in A. xanthioides. Lower levels of α-Thujene, Phyllanderenes, Ascaridole, and Pinocarvone were also observed in both species. According to DPPH (2,2-diphenylpicrylhydrazyl) assay, seed the extract of A. subulatum exhibited the highest antioxidant activity (78.26±9.27 %) followed by the seed extract of A. xanthioides (68.21±2.56 %). Similarly, FRAP (Ferric Reducing Antioxidant Power) assay showed that the highest antioxidant activity was exhibited by the seed extract of the two species; 20.14±1.11 and 21.18±1.04 µmol trolox g−1 DW for A. subulatum and A. xanthioides, respectively. In terms of anti-lipid peroxidation, relatively higher values were obtained for the fruit extract of A. subulatum (6.08±0.35) and the seed extract of A. xanthioides (6.11±0.55). Ethanolic seed extracts of A. subulatum had the highest efficiency against four Gram-negative bacterial species which causes serious human diseases, namely Pseudomonas aeruginosa, Proteus vulgaris, Enterobacter aerogenes, and Salmonella typhimurium. In addition, P. aeruginosa was also inhibited by the fruit extract of both A. subulatum and A. xanthioides. For the seed extract of A. xanthioides, large inhibition zones were formed against P. vulgaris and the fungus Candida albicans. Finally, we have in silico explored the mode of action of these plants by performing detailed molecular modeling studies and showed that the antimicrobial activities of these plants could be attributed to the high binding affinity of their bioactive compounds to bind to the active sites of the sterol 14-alpha demethylase and the transcriptional regulator MvfR.
Conclusion: These findings demonstrate the two species extracts possess high biological activities and therapeutical values, which increases their potential value in a number of therapeutic applications.
1 Introduction
Historically, medicinal plants were used worldwide either directly as therapeutics or as starting materials in drug formulation (Grover et al., 2002; Bauer and Brönstrup, 2014; Pešić and Stanković, 2015). The World Health Organization (WHO) evaluates that approximately 80% of the people worldwide now depend on medicinal plants for basic medical requirements (WHO establishes the Global Centre for Traditional Medicine in India (n.d.)). Medicinal plants are used to treat numerous illnesses, including diabetes, cardiovascular diseases, nervous system disorders, asthma, hypertension, and cancer (Prasathkumar et al., 2021). Natural antimicrobials found in medicinal plants are highly effective against newly emerging microbial strains (Cowan, 1999; Tijjani et al., 2011). Due to the inappropriate and excessive use of antimicrobial medications, microbes have become resistant to many antibiotics, which poses a significant challenge for the treatment of infectious illnesses (Davies, 1994; McGregor et al., 2014; Saleem et al., 2019; Thompson, 2022). Antibiotic resistance occurs due to the constant generation of resistant strains to drugs and the adaptation of microorganisms to frequently used antibiotics (Ventola, 2015; Aslam et al., 2018; Saleem et al., 2019). Lately, the interest in medicinal plants has increased because of the great potential of plant-based medicines. Consequently, one of the main sources of commercial pharmaceuticals is still the useful compounds extracted from medicinal plants. In addition to being medicinal, numerous plant extracts have been widely employed in fragrances, food flavoring, and food preservation (Pandey et al., 2017; Delesa, 2018).
Medicinal plants are distributed and considered a valuable source of novel medications worldwide (Balunas and Kinghorn, 2005; Rafieian-Kopaei, 2012). The usage of medicinal plants is expanding quickly globally due to the rising need for herbal medicines, healthcare products, and plant secondary metabolites. Therefore, isolation and purification of extracts from medicinal plants are important for discovering and developing new drugs. A. subulatum, generally recognized as black cardamom, is a herbaceous plant used as a medicinal spice in India (Gautam et al., 2016). It has historically been used to treat vomiting, abdominal pain, gastrointestinal (GI) infections, and rectal diseases (Kumar et al., 2015; Alam and Singh, 2021; Dhakal et al., 2022). The therapeutic capabilities of this medicinal herb have gained great attention in the past years since it is found to be a source of antimicrobials, antioxidants, anti-inflammatory, and cardio-protective compounds (Bhaswant et al., 2015; Gautam et al., 2016). The essential oil components of A. subulatum are primarily responsible for its therapeutic capability (Dhakal et al., 2022). Essential oils from A. subulatum seeds are found to have an antimicrobial effect against various pathogens including Staphylococcus aureus, Escherichia coli, Bacillus pumilus, Pseudomonas aeruginosa, and Aspergillus niger (Agnihotri and Wakode, 2010; Satyal et al., 2012). Hexane seed extract of A. subulatum exhibited high cellular toxicity on HeLa and MCF-7 cell lines, which are human cancer cell lines, indicating its anticancer potential, while the ethyl acetate extract possessed a considerable antioxidant activity based on the free radical scavenging assay (Sharma et al., 2017). In addition, fruit extracts of A. subulatum have shown high anti-inflammatory effects in rats with carrageenan-induced paw edema when compared with diclofenac, the main drug for this disease (Alam et al., 2011). The fruit extracts also exhibited anticancer activity in mice through the control of cytokines that cause inflammation as well as the NF-κB signaling (Sudarsanan et al., 2021). A recent study discovered that the dichloromethane extract of A. subulatum had an apoptotic effect against lung cancer cells (Makhija et al., 2022).
Amomum xanthioides is a vigorous herb that is used for food and medicinal purposes in southern China, India, Thailand, Vietnam, Laos, and Cambodia (Lamxay and Newman, 2012). Ethyl acetate extracts from A. xanthioides seeds are found to have therapeutic potential against liver fibrosis in rat models (Kim et al., 2015). When administered to high-fat-diet mice, ethyl acetate extract from A. xanthioides showed an anti-fatty liver effect, indicating its therapeutic potential in managing non-alcoholic fatty liver disorders (Im et al., 2020). Moreover, anti-inflammatory effects are associated with A. xanthioides extracts in atopic dermatitis, a chronic relapsing skin inflammation (Choi et al., 2017). Essential oils extracted from A. xanthioides fruits were found to have growth inhibitory effects on Enterococcus faecalis, Bacillus cereus, P. aeruginosa, and S. aureus, with minimum inhibitory concentration values between 100 and 200 g/ml (Thinh et al., 2022).
In spite of all the previous data, A. subulatum and A. xanthioides are not being given much consideration for therapeutic development as a result of a lack of comprehensive chemical analysis and pharmacological research (Thinh et al., 2021; Dhakal et al., 2022). In this manner, the current study aimed to provide insight into the phytochemical composition of both species by investigating the bioactive components in their seeds and fruits, including flavonoids, phenols, vitamins, steroids, and essential oils. To this end, techniques such as gas chromatography–mass spectrometry (GC-MS) and high-performance liquid chromatography (HPLC) were employed. In addition, the antimicrobial and antioxidant potentials of the ethanol extracts were assessed through multiple assays. Finally, computational docking was performed to investigate the molecular interactions of the proposed compounds against certain microbial proteins determined from prior analysis to confirm the antimicrobial activity observed from these compounds.
2 Materials and methods
2.1 Plant sample collection and identification
The seeds and fruits of A. subulatum and A. xanthioides were obtained at a local Pakistani store in Antwerp, Belgium. The plant specimen was identified and authenticated by a plant taxonomist at the Botany Department of Beni Suef University, Egypt.
2.2 Total nutrients
Sugars were extracted in 0.2 g dry weight of Amomum seeds and fruit samples in 2 ml of boiled distilled water for 60 min at 100°C. After being cooled, the extract was centrifuged at 6,000 ×g for 15 min. The pellet was re-extracted using 2 ml of boiled distilled water. After centrifugation, the two supernatants were combined for further analysis. The concentration of total sugars in the supernatant was assessed following Nelson’s method as defined by Clark and Switzer (1977). Sugar extract was added to freshly prepared Nelson’s alkaline copper reagent (1:1 v/v), and the mixture was boiled for 20 min. Subsequently, 1 ml of arseno-molybdate reagent was added to the reaction mixture with shaking to dissolve Cu2O. When the effervescence stopped, the change in color intensity was measured at 540 nm. The reduced sugar content was determined by means of a glucose standard curve. The protein concentration was assessed for Amomum seeds and fruits (0.2 g DW) extracted twice in NaOH (0.4% w/v) at room temperature. Extracts were shaken by using an orbital shaker at 220 rpm for 45 min. The total protein content was measured by using Lowry’s method at 660 nm (Oh et al., 1951). Bovine serum albumin was used as a standard reagent. Total lipid concentrations were determined, where Amomum seeds and fruit samples were homogenized twice in a 2:1 mixture of chloroform/methanol (v/v). Then plants were centrifuged for 15 min at 3,000 ×g. In a 4:1 ratio of toluene:ethanol (v/v), the pellets were re-dissolved. The total lipid content was determined after concentration. Gravimetric analysis was used to determine the extracted lipids, which were represented as weight (g) per fresh weight (g) of the plant. To eliminate unwanted protein and starch, crude fibers were gelatinized by a heat-stable alpha-amylase at pH 6 and 100°C for 25 min and then enzymatically digested by a combination of protease (pH 7.5, 60°C, 25 min) and amyloglucosidase (pH 6, 0°C, 30 min). Fibers were allowed to precipitate in ethanol for washing, and the residues were weighed after washing.
2.3 Mineral quantification
The recognition of mineral elements was achieved according to AbdElgawad et al. (2014), where 200 mg dry weight of Amomum seeds and fruit samples was digested in 5:1 (v:v) HNO3/H2O solution for 30 min. Thereafter, macroelements and microelements were estimated (inductively coupled plasma–mass spectrometry (ICP-MS), Finnigan Element XR, and Scientific, Bremen, Germany). Nitric acid (1%) was used as blank.
2.4 Total phenolics and flavonoids
Total polyphenols and flavonoids were obtained by homogenizing 200 mg dry weight of Amomum seeds and fruit samples in 2 ml of 80% ethanol (v/v). The contents of phenolic and flavonoid were assessed using the Folin–Ciocalteu and aluminum chloride colorimetric assays, respectively (Saleh and Madany, 2015), with gallic acid and quercetin as standards, respectively (Sigma-Aldrich Co., St. Louis, MO, USA). For the determination of total phenolics, 1 ml of the phenolic extract was mixed with 1 ml of 10% Folin–Ciocalteu phenol reagent and 1 ml of 20% anhydrous Na2CO3 and afterward filled to a given volume with distilled water. The absorbance of the resulting blue color was observed after 30 min at 650 nm against a water-reagent blank (samples extracted and replaced by distilled water). The total phenolic content was determined from a catechol (Sigma-Aldrich Co., St. Louis, MO, USA) standard curve and reported as mg gallic/g dry weight. Total flavonoid content was determined by mixing 0.25 ml of the extract with 1.25 ml of distilled water in a test tube, followed by the addition of 75 µl of 5% (w/v) Na nitrite solution. After 6 min, 150 µl of 10% (w/v) AlCl3 was added, and the mixture was left for another 5 min prior to adding 0.5 ml of 1 M of sodium hydroxide. The solution was filled and mixed with distilled water up to 2.5 ml, and the absorbance was recorded at 510 nm. The total flavonoid content was measured using a quercetin standard curve and reported as mg quercetin/g dry weight.
2.5 Total alkaloid estimation
The total alkaloid was estimated following the protocol described by Sreevidya et al (Sreevidya and Mehrotra, 2003). along with the bismuth nitrate pentahydrate (Bi(NO3)3·5H2O) calibration curve. Samples were extracted in methanol-HCl at pH 2–2.5. The extract was mixed with Dragendorff’s reagent (bismuth nitrate pentahydrate, glacial acetic acid, and 8.0 g of potassium iodide) and centrifuged for 10 min at 5,000 rpm, 25°C. The precipitate was further washed twice with methanol, and the residue was added to a disodium sulfide solution. The resulting brownish-black precipitate was afterward centrifuged for 10 min at 5,000 rpm. The residue was dissolved in concentrated nitric acid by mild warming. This solution was diluted with distilled water and mixed with a 3% thiourea solution. At 435 nm, the absorbance was recorded in comparison to a blank containing HNO3 and thiourea by using a spectrophotometer (Perkin Elmer Lambda 25).
2.6 Saponins
The extraction and quantification of saponin in Amomum seeds and fruit samples were performed (Lai et al., 2013). Ground dried seeds and fruits were extracted in petroleum ether and shaken for 4 h at 1,000 rpm and 25°C ± 3°C. The solvent was then removed using a vacuum rotary evaporator at 60°C. Saponin in residues was extracted in 80% aqueous methanol and shaken for another 4 h. The extract was filtered and preserved at 4°C in the dark. For quantification, the spectrophotometric technique was applied to estimate the total saponin content of the samples. In a cold-water bath (0°C), 0.1 ml of the extract attained above was added to 0.4 ml of methanol solution (80%), 0.5 ml of freshly prepared vanillin solution (8% (w/v; prepared in ethanol), and 5.0 ml of sulfuric acid (72%). After that, the mixture was put in a water bath at 60°C for 10 min and lastly cooled in ice-cold water. The absorbance was measured at 544 nm against a reagent blank with a UV–Vis spectrophotometer (Shimadzu UV-160A PC, Shimadzu Corporation, Kyoto, Japan). The reagent blank was prepared by applying the same technique, but the extract was exchanged with an equivalent volume of 80% methanol. The results were estimated from a standard curve plotted with various crude soya saponin concentrations (0, 1,000, 2,000, and 3,000 ppm) containing at least 80% saponin (Waki, Osaki, Japan) in 80% aqueous methanol and expressed as mg soya saponin/100 g sample.
2.7 Fatty acid profile
The fatty acid profile was quantified according to Hassan et al. (2018). To obtain lipophilic fraction, 0.2 g dry weight of Amomum seeds and fruit samples was extracted in chloroform/methanol (2:1, v/v) at 25°C in the presence of the internal standard tripentadecanoic acid triglyceride. Fatty acids were derivatized with 1% pentafluorobenzyl (PFB) bromide in acetonitrile at room temperature for 20 min. The fatty acid PFB esters dissolved in 50 μl of iso-octane are injected. The investigation was applied by means of an Agilent single-quadrupole mass spectrometer with an inert mass selective detector (MSD-5975C detector, Agilent Technologies, Santa Clara, CA, USA) coupled directly to an Agilent 7890A gas chromatograph that was equipped with a split–splitless injector, a quick-swap assembly, an Agilent model 7693 autosampler, and an HP-5MS fused silica capillary column (5% phenyl/95% dimethylpolysiloxane, 30 m × 0.25 mm i.d., film thickness 0.25 μm, Agilent Technologies, USA). The temperature of the oven was kept at 80°C for 2 min and then elevated up to 200°C at 5°C/min (1 min hold) and then to 280°C at 20°C/min (3 min hold). A 1.0-μl sample was injected by means of a split mode (split ratio, 1:10). At a flow rate of 1.5 ml/min, helium gas was employed as a carrier gas. For MS detection, an electron ionization technique with an ionization energy of 70 eV was applied. The temperatures of the injector and MS transfer line were set at 220°C and 290°C, respectively. The mass scan ranged from 50 to 550 m/z with an Em voltage of 1,035 V. The quantitative examination of fatty acids is performed by comparing the target molecule’s mass spectrometric ion signal to that of an equivalent standard. Fatty acid standard curves were obtained by serially diluting a standard mixture of unlabeled quantitative fatty acid standards at specified quantities. Each fatty acid is given in quantitative standard dilution sets in the 0.15–500-ng range. A standard curve is created by doing a linear regression model on the ratio of the quantitative standard and internal standard ion yields plotted vs. the quantitative standard absolute quantities. The fatty acid content of the sample is then determined from the standard curve using analyte/internal standard ion yield ratios. In parallel, software freely available for the deconvolution of fatty acids spectrum was applied; i.e., the metabolite libraries available for metabolite identification were obtained using NIST08, a generalized chemical library (http://chemdata.nist.gov/mass-spc/ms-search/), and those specifically for metabolites were obtained using the Golm Metabolome (http://gmd.mpimp-golm.mpg.de).
2.8 Essential oil analysis
Plant samples were placed in 1 L of distilled water and subjected to hydrodistillation for 3 h, using a Clevenger-type apparatus (1.5% yield). To remove water remnants from essential oil, anhydrous Na2SO4 was utilized. The essential oil was stored at +4°C until tested and analyzed.
2.9 Gas chromatography and gas chromatography–mass spectrometry
Gas chromatography–flame ionization detector (GC-FID) and GC-MS were used to determine the essential oil both quantitatively and qualitatively. GC analyses were performed on a Varian (Les Ulis, France) Star 3400 Cx chromatograph fitted with a fused silica capillary DB-5MS (5% phenyl methylpolysiloxane; 30 m/0.25 mm; film thickness, 0.25 mm) column. Chromatographic conditions were 60°C to 260°C temperature increases with a gradient of 5°C/min and 15 min isothermal at 260°C. A second increase was applied, reaching 340°C at 40°C/min. The total time of the analysis was 57 min. Petroleum ether was used for dissolving the oils to avoid saturating the column. Injection of the sample was performed at a split mode ratio of 1:10. Helium (purity 99.999%) was utilized as the carrier gas at 1 ml/min. The injector was operated at 200°C. The mass spectrometer (Varian Saturn GC/MS/MS 4D) was set at an electron multiplier voltage between 1,400 and 1,500 V and an emission current of 10 mA. The transfer line’s temperature was 170°C, whereas the trap’s temperature was 150°C. A total of 40 to 650 atomic mass units was covered by the mass scanning. The components were recognized by means of Wiley 2001 library data (NIST 02 version 2.62) of the GC-MS system, literature data, and comparison of the components’ Kovats indices (KIs) and mass spectra with those of standards. In order to calculate KI, alkanes (C5–C24) were employed as reference points. Every determination was made twice and then averaged.
2.10 Vitamins
Carotene and β-cryptoxanthin contents were obtained in acetone and examined by a reversed-phase HPLC conducted with a diode array detector (Sarungallo et al., 2015). To extract carotene and β-cryptoxanthin, seed samples were shaken in a MagNa Lyser (3 × 10 s, 6,000 rpm) using acetone as a solvent. After centrifugation, the sample was brought in the autosampler of the HPLC (Shimadzu SIL10-ADvp) and kept at 4°C. The separation of the carotenoids and β-cryptoxanthin was performed using a reversed-phase method with a low-pressure gradient and was performed on a silica-based C18 column (Waters Spherisorb 5-µm ODS1 4.6 × 250 mm). Solvent A (acetonitrile:methanol:water, 1:9:10) and solvent B (methanol:ethyl acetate, 68:32) act as the mobile phase. The detection of the carotene and β-cryptoxanthin was performed by a diode array detector (Shimadzu SPD-M10Avp) at a wavelength range of 446–470 nm and integrated via the software program (Shimadzu Lab Solutions Lite).
Phylloquinone was detected according to the methods of Jakob et al (Jakob and Elmadfa, 1996). A reversed-phase HPLC system and samples were separated on analytical column Gynkotek ODS Hyper-sil (250 · 4.6 mm i.d., 5 lm), a guard-column Gynkotek ODS Hypersil (20 · 4.6 mm i.d., 5 lm, Bischoff, Leonberg, Germany). The mobile phase contained 1 L of dichloromethane–methanol mixture (ratio 1:9). This solvent was then combined with 5 ml of a methanolic solution containing l.37 g of ZnCl2, 0.41 g of CH3COONa, and 0.30 g of CH3COOH. Recognition was performed at 243-nm excitation and 430-nm emission. The concentrations were calculated using a linear regression curve from standard solutions.
Tocopherols were obtained with hexane by means of the MagNa Lyser. The dried extract (CentriVap concentrator, Labconco, KS, USA) was resuspended in hexane, and tocopherols were isolated and estimated by HPLC (Shimadzu, ‘s Hertogenbosch, The Netherlands) (normal phase conditions, Particil Pac 5 µm column material, length 250 mm, i.d. 4.6 mm). Dimethyl tocol (DMT) was used as an internal standard (5 ppm). Data were investigated with Shimadzu Class VP 6.14 software.
2.11 UHPLC–MS/MS determination of phenolics and flavonoids
For quantification of individual phenolic acids and flavonoids, 0.3 g of dried powdered seeds or fruits was extracted in 2 ml of 80% (v/v) ethanol in a water bath at 70°C for 0.5 h. After centrifugation (12,000 rpm for 30 min), the supernatant was concentrated by a rotary evaporator (IKA-WERKE-RV06ML; Staufen, Germany). The obtained residue was dissolved in HPLC-grade methanol (final concentration = 1,000 ppm). All solutions were filtered through a 0.45-μm membrane filter (Iwaki Glass) before analysis. An Acquity UPLC System from Waters (Milford, CT, USA) was used for the extract’s chromatographic analysis. This system is supplied with a binary solvent supply system, degasser, autosampler, and column heater. Utilizing a 100 mm × 2.1 mm Acquity BEH C18 column from Waters with 1.7-m particle size, chromatographic separation was carried out. A Waters (Manchester, UK) Xevo TQD tandem quadrupole mass spectrometer was used for MS/MS detection together with an electrospray ionization interface (ESI) that worked in the negative ion mode. The capillary voltage was 4.5 kV, the source temperature was 120°C, the desolvation gas temperature was 400°C, and the nitrogen flow rates for the cone and desolvation gases were 30 and 600 L/h, respectively. Eluent A, ultrapure water containing 0.1% formic acid, and Eluent B, acetonitrile, were the components of the mobile phase. Samples measuring 2 μl were introduced at a flow rate of 0.2 ml/min with a linear gradient starting at 3% B and escalating to 100% B in 10 min. The presence of phenolic chemicals in the sample was confirmed using the multiple-reaction monitoring (MRM) mode, together with m/z transitions of the precursor ions and product ions.
3,5-Dichloro-4-hydroxybenzoic acid (Sigma-Aldrich Co., St. Louis, MO, USA) was utilized as an internal standard to take into consideration recovery losses and ionization efficiencies. The concentrations were calculated based on the relative response of the internal analyte in relation to this internal standard added. The amount of internal tracer is always chosen in relation to the internal concentration of the analyte, taking into account that the concentration difference does not exceed a factor of 100, the range in which linearity is guaranteed.
2.12 DPPH antioxidant assay
Seven samples (300 µl of methanolic extract) were mixed with 900 µl of DPPH solution (4 × 10−5 M). After incubation at 60 nm in the dark at 37°C, the absorbance was measured at 517 nm with a spectrophotometer UV–visible (A(sample)). A blank solution was also measured at the same wavelength (A(blank)). The free radical-scavenging activity of each solution was then calculated as percent inhibition according to the following equation: (radical scavenging activity (%) = ((A(blank) − A(sample))/A(blank)) × 100).
2.13 FRAP assay
The ferric ion-reducing antioxidant power (FRAP) activity was determined in the alcoholic (80% ethanol) seed and fruit extracts, which showed the highest activity by FRAP methods (AbdElgawad et al., 2019). FRAP assay was performed by adding 20 μl of each extract to a micro-titer plate and filling up to 200 μl of freshly prepared pre-warmed FRAP reagent. The mixture was incubated for 30 min at 37°C. The absorbance was determined at 593 nm. The antioxidant capability of the extracts was measured using the Trolox calibration curve.
2.14 TBARS lipid peroxidation assay
The level of lipid peroxidation was assessed by the thiobarbituric acid reactive substance (TBARS) method, using an egg yolk homogenate as the lipid-rich medium (Ohkawa et al., 1979). The seed extract and egg homogenate (0.5 ml of 10% v/v) were mixed with 15 mM of ferrous sulfate (to induce lipid peroxidation), and after 0.5 h, 1.5 ml of 10% trichloroacetic acid (TCA) was added. The mixture was then added to a 1.5-ml solution of 0.67% TBA and then boiled for 0.5 h. The chromogen obtained was measured at 535 nm.
2.15 Antimicrobial activity
The antibacterial activities of the seed and fruit ethanolic extracts were verified using the disc diffusion method (bacterial suspension containing 106 CFU/ml of the bacterial test strain spread on Mueller–Hinton agar). Each extract was put on sterilized filter paper discs (5 μg/disc). Absolute ethanol was used as a negative control. These discs were placed on the agar plates to be incubated at 37°C for 24 h. The inhibition zones were measured by Vernier caliper. Regarding antifungal activity, a well diffusion assay was used to determine the antifungal properties of seed and fruit ethanolic extracts. Inoculum measuring 0.2 ml (fungal strain in saline) was spread on an agar plate. Five ditches of 4 mm were made on each plate. Absolute ethanolic plant extracts (50 mg/ml) were prepared, and each well was filled with 50 μl of the methanolic extracts. Fluconazole (5 mg/ml) was used as a positive control, while absolute ethanolic was used as a negative control. The plates were incubated at 37°C for 24 h. Strains of Candida species were obtained from the Research Laboratory, Clinical Laboratory Sciences Department, Juef University, Saudi Arabia.
2.16 In silico studies and molecular docking
The 3D structures of the proposed molecules were retrieved from the PubChem database and energy-minimized to investigate their interactions with microbial proteins through molecular docking analyses. The proposed molecules were five essential oils (cadinol, cryptone, elemol, thyme, and pinene), tocopherol (vitamin E), kaempferol (flavonoid), and glutamine (amino acid). Two microbial receptors were targeted: sterol 14-alpha demethylase from Candida albicans (Protein Data Bank (PDB): 5FSA (Hargrove et al., 2017)) and the transcriptional regulator MvfR of P. aeruginosa (PDB: 6Q7U (Zender et al., 2020)). The receptors were retrieved from the PDB database and prepared for docking through the removal of associated ligands and solvent molecules and the addition of polar hydrogen atoms. In AutoDock, the grid box was adjusted to target the entire protein target with a grid spacing of 1 Å between the set points (Morris et al., 2009). Docking simulations and binding affinity were performed and calculated using AutoDock Vina (Trott and Olson, 2010). The molecular interactions were further investigated by visualizing the formed chemical bonds in each ligand–receptor complex as well as the 3D surface structures showing the aromatic interactions, H-bond formation, ionizability, solvent-accessible surface (SAS), and other properties by using BIOVIA Discovery Studio (Gaber et al., 2020; Samaha et al., 2020; El Azab et al., 2021; Gaber et al., 2021; Mohamed et al., 2021; Saied et al., 2021; Healey et al., 2022; Khirallah et al., 2022a; Khirallah et al., 2022b).
2.17 Statistical investigation
Our tests were accomplished as quadruple. The data are shown as mean ± standard error of the mean. Results were investigated by using one-way ANOVA and subsequently Tukey’s post hoc test. The significance of the data was defined by p-value; p > 0.05 is regarded as non-significant and p < 0.05 as significant. The statistical investigation was performed using the software program GraphPad Prism (GraphPad Software, San Diego, CA, USA, 2007) (Abdel-Wahab BA et al., 2022; Mohamed et al., 2022b; Mohamed et al., 2022a).
3 Results and discussion
3.1 Proximate composition analysis
For A. subulatum, sugar has the largest total content among other nutrients. For seeds, it was estimated to be approximately 33% of the total nutrient content in seeds, while that in fruits was approximately 25.5% of the nutrient content. Higher sugar content was found in A. xanthioides than A. subulatum. After sugars, both species contained considerable amounts of proteins and lipids. Notably, the protein content is usually higher than the total lipids in both species except the fruit of A. subulatum, which contains more lipids than proteins. However, lower amounts of steroids, tannins, and alkaloids were also observed. The investigation of alcoholic extracts of some Amomum species revealed the presence of various phytoconstituents, including flavonoids, carbohydrates, anthocyanin, tannins, phenols, alkaloids, and steroids (Konappa et al., 2020). Li et al. reported that Amomum tsao-ko is rich in a variety of chemical components of proteins, phenolic compounds, tannins, organic acids, saponins, flavonoids, anthraquinone, coumarin, lactones, steroids, terpenoids, volatile oil, anthocyanins, and so on (Li et al., 2021). As tannin, a wide group of water-soluble polyphenolics, and alkaloid possess anti-nutritional and anti-feed properties (Mueller-Harvey, 2006; Itkin et al., 2013), low availability of these compounds indicated high tissue quality of both Amomum species. However, tannins possessed antioxidant characteristics and have been shown to reduce total cholesterol, blood pressure, and immune system stimulation (Tong et al., 2021). Other nutrients are present in much lower concentrations including flavonoids, crude fiber, and ash (Figures 1A, B). The content of ash often indicated high levels of inorganic compounds and essential macroelements (Alzahrani et al., 2017).
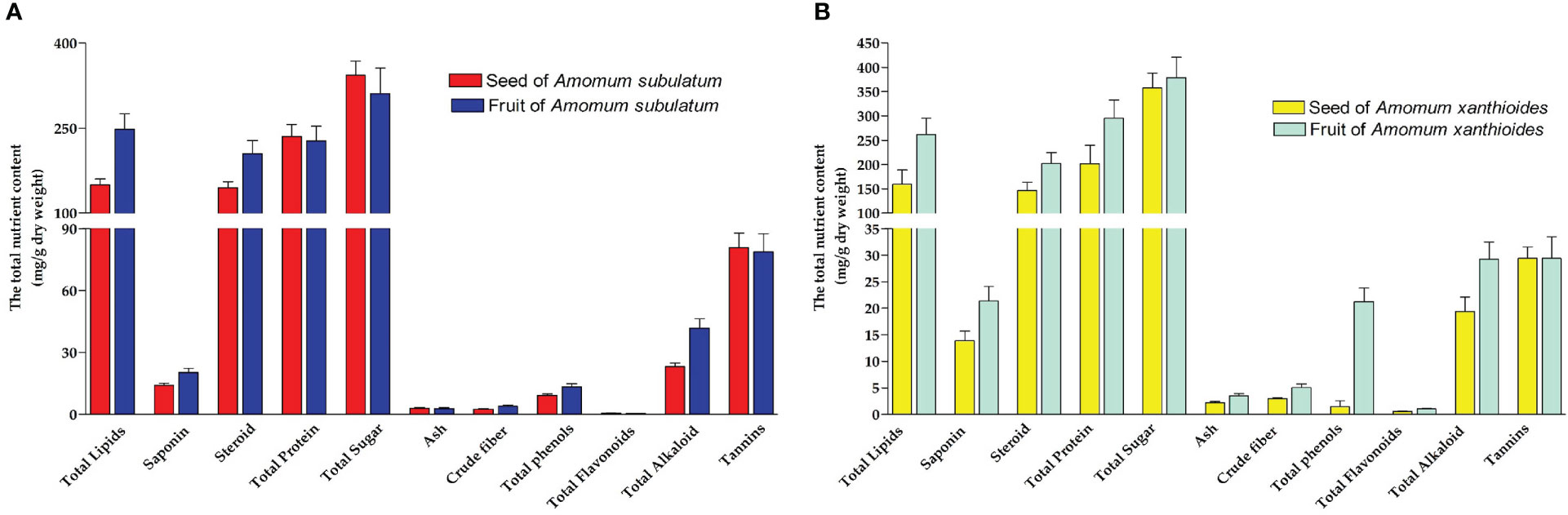
Figure 1 The total nutrient content (mg/g dry weight) in (A) seed and fruit of Amomum subulatum and (B) seed and fruit of Amomum xanthioides. The data are shown as the mean ± SEM of four plants per group.
This is consistent with the antioxidant activity associated with the ethyl acetate seed extract and the immune response activated in mice upon administration of fruit extracts (Sharma et al., 2017; Sudarsanan et al., 2021). Moreover, an anti-inflammatory function associated with tannins from various sources was reported in other studies (Park et al., 2014; Ambreen and Mirza, 2020; Sharma et al., 2021), which explains the beneficial use of A. xanthioides in the management of atopic dermatitis (Choi et al., 2017).
3.2 Essential oil isolation
Our data revealed that there are several essential oils found in both A. subulatum and A. xanthioides with different concentrations in both seeds and fruits (Table 1). Some of them showed significant differences between the two species in either seed or fruits, while the others showed no significant changes between the two species. High levels of thyme oil may promote the antimicrobial activity associated with A. subulatum and A. xanthioides (Satyal et al., 2012; Choi et al., 2017; Thinh et al., 2022). Thyme oil is used in food preservation and cosmetics due to its antibacterial, antifungal, and anti-inflammatory characteristics (Lorenzo et al., 2019). Thyme oil aids in promoting blood flow to the skin, which improves the healing process and removal of scars and imperfections, leaving the skin even and healthy (Zarzuelo and Crespo, 2002; Salehi et al., 2018). Reports of antibacterial activity of thyme oil have been found against Clostridium botulinum, a gram-positive bacterium that produces one of the most lethal neurotoxins known (De and De, 2019; Corsalini et al., 2021). In addition, thyme oil significantly reduced the growth of the fungus Pyrenochaeta terrestris during soil treatment, which suggests the potential use of thyme oil in the biological control of plant diseases (Hussien and Ibrahim, 2020). Due to their wide range of applications, A. subulatum and A. xanthioides can be used as sources for the extraction and production of thyme oil.
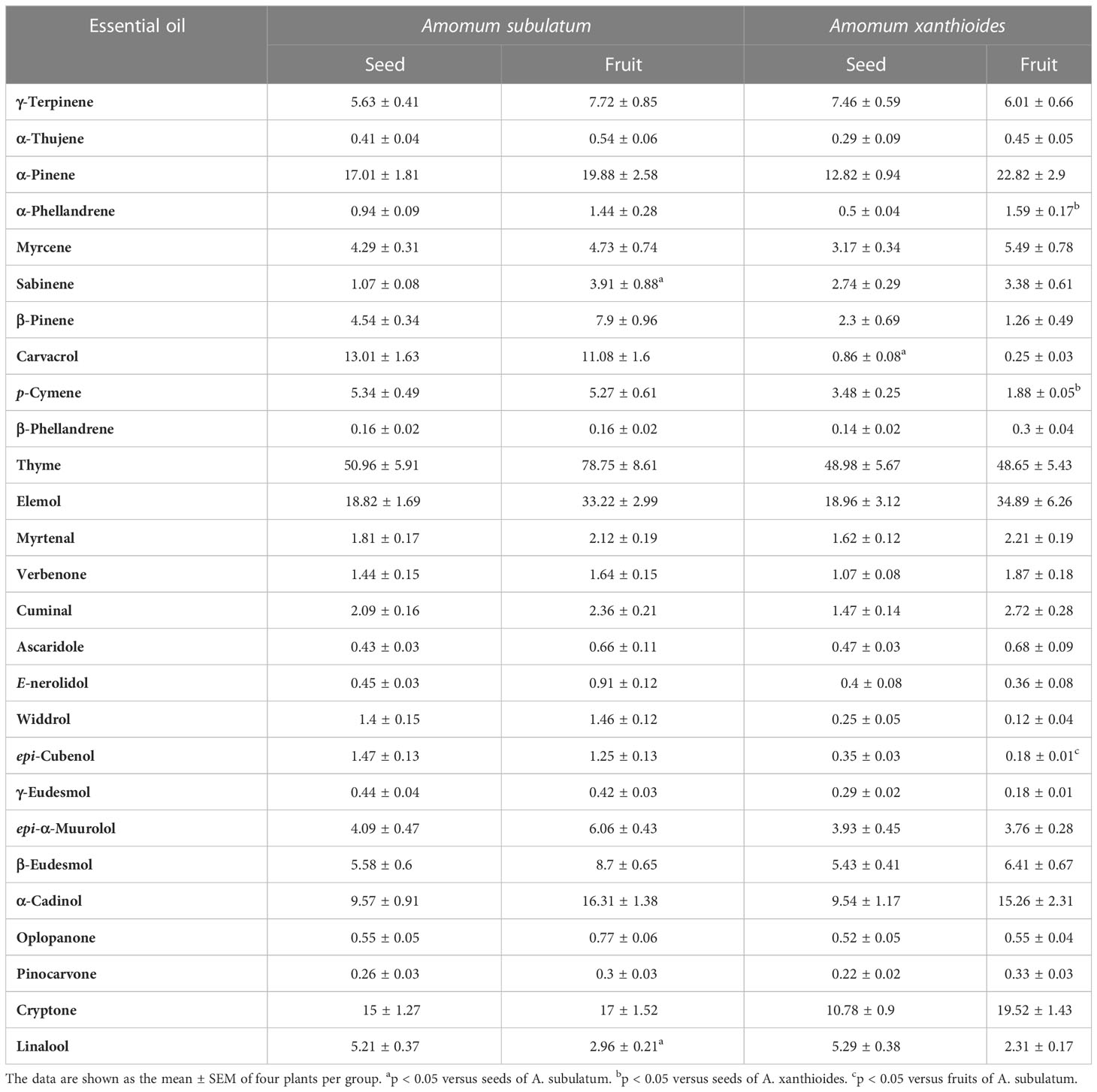
Table 1 Essential oil percentages (v/g dry weight) in the seed and fruit of Amomum subulatum and Amomum xanthioides.
After thyme, elemol oil is the next-most prevalent oil in both species. The percent of elemol oil in seed was approximately 19%, while that in fruits was 34% for the two species. It is commonly used in fine fragrances as well as other applications (Bhatia et al., 2008). Elemol oil isolated from essential oils of Rhynchanthus beesianus rhizomes exhibited insecticidal activities against the adults of Liposcelis entomophila and Tribolium castaneum insects (Pan et al., 2022). Essential oils of Cymbopogon schoenanthus contained 22.8% elemol oil and exhibited larvicidal effects against Anopheles funestus and Culex quinquefasciatus larvae with lethal concentrations of 120.5 and 23.32 ppm, respectively (Wangrawa et al., 2022). In addition, elemol was the most abundant constituent (13.54%) in the essential oils of Drimys winteri, which showed insecticidal activity against the pests Acanthoscelides obtectus and Aegorhinus superciliosus (Tampe et al., 2020). The reported insecticidal activity of essential oils with elemol in multiple studies suggests its role as a natural eco-friendly insecticide for the biocontrol of herbivorous arthropods.
In addition to thyme and elemol components, considerable levels of the terpenoid α-pinene were also noticed in the two species. 1,8-Cineole, α-pinene, α-terpinene, and β-pinene were found to be the major components in Amomum kravanh (Diao et al., 2014). The oil has demonstrated a strong anticancer effect on various cell lines in addition to anti-inflammatory effects against multiple autoimmune disorders (Salehi et al., 2019; Prado-Audelo et al., 2021), which agrees with the high therapeutic activity of A. subulatum. Essential oils from Magnolia candollei, which contained 29.7% α-pinene and 10.2% elemol components, exhibited inhibitory against lipoxygenase and acetylcholinesterase, suggesting the potential therapeutic capabilities of these oils in diseases related to lipid metabolism as well as neurological disorders (Yahaya et al., 2022). Noticeably, the carvacrol levels in A. subulatum were 10 times higher than those in A. xanthioides. Lower levels of α-thujene, phellandrenes, ascaridole, and pinocarvone were also observed in both species. Other components were also isolated and summarized in Table 1. Statistical analyses showed that A. subulatum contained significant correlation levels (p < 0.05) of sabinene component in fruits more than in seeds while having linalool in seeds more than in fruits (p < 0.05). For A. xanthioides, significantly higher levels (p < 0.05) of α-phellandrene were observed in fruits. In addition, cymene seed levels were higher compared to those of fruits in A. xanthioides. When compared to A. xanthioides, A. subulatum showed higher significance (p < 0.05) of carvacrol in seeds and lower significance (p < 0.05) of cubenol in fruits. α-Pinene from some Salvia spp. showed moderate antibacterial activity against multiple bacterial species including Bacillus subtilis, S. aureus, and Staphylococcus epidermidis with inhibition zones ranging from 13 to 15 mm and minimum inhibitory concentration (MIC) values of 3.7–7.5 mg/ml (Yousefzadi et al., 2007). Moreover, α-pinene had a strong antifungal potential against Candida species isolated from otomycosis patients, indicating its promising clinical utility as a natural drug (Nóbrega et al., 2021). In this regard, it was suggested that essential oil might bind to the bacterial cell surface and then penetrate the phospholipid bilayer of the cytoplasmic membrane. Consequentially, it results in the leakage of various vital intracellular constituents and leads to cell death (Lv et al., 2011). Thus, the presence of α-pinene and elemol components in considerably high amounts in A. subulatum and A. xanthioides makes the plant species extremely important sources of these oils due to their great benefits in fighting both plant and human pathogens.
3.3 Elemental analysis
In the seed and fruit of both species, nitrogen was the highest frequent element followed by potassium and then phosphorus (Figures 2A, B). A. subulatum contained lower levels of nitrogen (~45 mg/g dry weight) than A. xanthioides seed and fruit. Some elements were found in much lower amounts (less than 3 mg/g dry weight) including calcium, magnesium, sodium, and zinc. A significant increase in levels (p < 0.05) of magnesium was observed in the fruits of A. subulatum compared to seeds. However, zinc levels were significantly higher (p < 0.05) in fruits of A. subulatum compared to fruits of A. xanthioides. It was also reported that Amomum longiligulare is rich in microminerals (Chau et al., 2015). In this context, edible Zingiberaceae plants contained relatively high macroelement amounts including K, Ca, and Fe (Rachkeeree et al., 2018). Nitrogen is regarded as a critical macronutrient among all mineral nutrients including all living tissues within the plant, ranging from metabolism to resource allocation, growth, and development. Nitrogenous compounds play several roles in metabolism, including acting as protein building blocks and as precursors in the biosynthetic pathways of substances like lignin (Famiani et al., 2020; Yousaf et al., 2021). Phosphorus is a crucial nutrient in crop yields since many soils in their natural condition do not have enough accessible phosphorus to enhance crop yield (Sett and Soni, 2013). Potassium supplementation improved osmotic adjustment and water relations in a variety of crop species. K alleviates drought conditions in plant material primarily by managing stomatal closure and preserving stromal pH, thereby lowering photo-oxidative injury to chloroplasts (Santos et al., 2021). This means that these plants have excellent quality in improving the osmotic adjustment of water and preserving stromal pH.
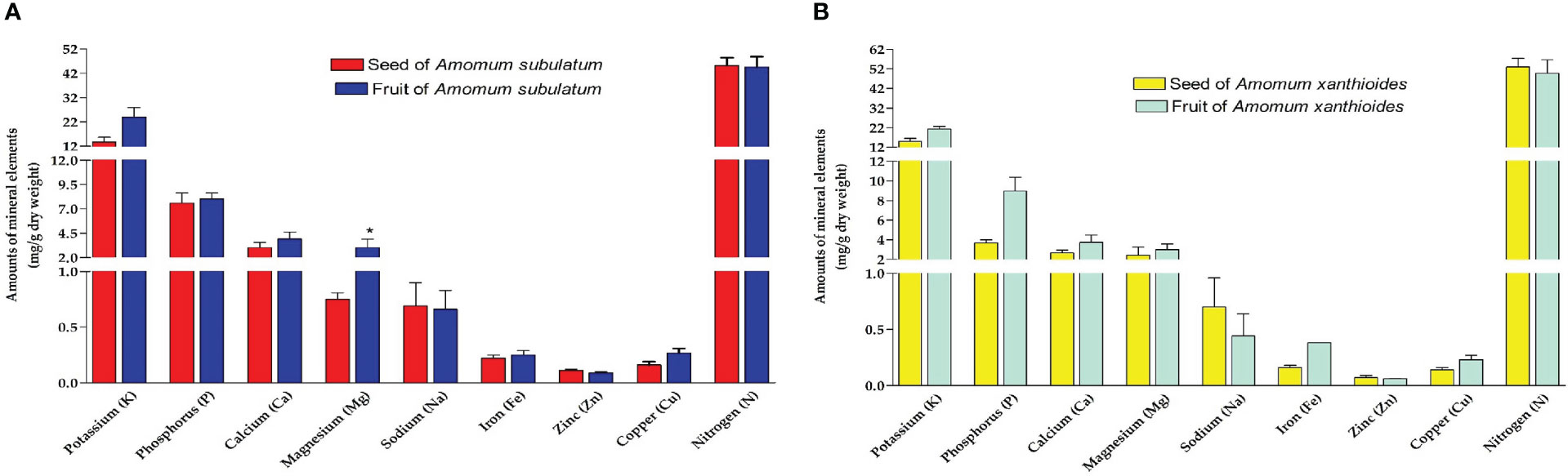
Figure 2 Amounts of mineral elements extracted (mg/g dry weight) in (A) seed and fruit of A. subulatum, (B) seed and fruit of A. xanthioides. The data are shown as the mean ± SEM of 4 plants per group. a P < 0.05 versus seeds of A. subulatum, c P < 0.05 versus fruits of A. subulatum.
3.4 Total phenolics and flavonoids
In determining the total phenolic and flavonoid components in both species A. subulatum and A. xanthioides in either seeds or fruits, we found that both species had no significant difference in the phenolic or flavonoid levels. However, there was a significant increase in the levels of caffeic acid and velutin (p < 0.05) in A. xanthioides fruits compared to seeds (Figures 3A, B). The phenolic–flavonoid profiling showed that only gallic acid, a phenolic compound, is produced in high amounts in the two species compared to the other screened compounds. Most of the phytochemical constituents in Amomum sp. are phenolics, flavonoids, and essential oils (Alkandahri et al., 2021). Smaller amounts of other phenolics including caffeic acid, ferulic acid, and catechin were found. Isolated flavonoids were quercetin, kaempferol, naringenin, luteolin, and apigenin. Similarly, quercetin was previously isolated from A. longiligulare (Chau et al., 2015).
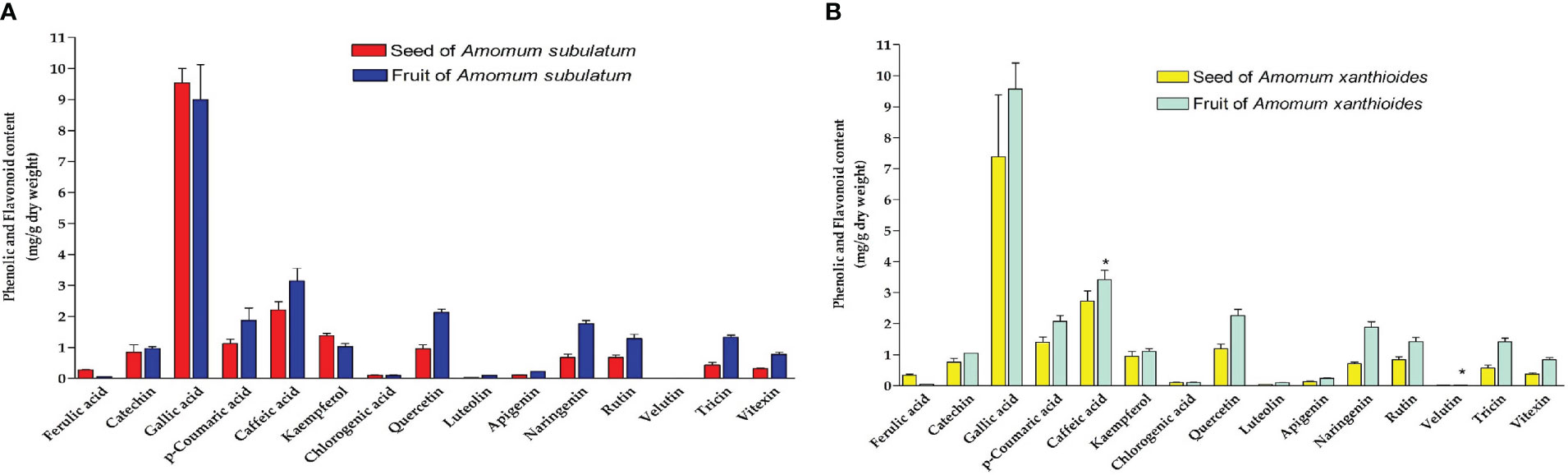
Figure 3 Phenolic and flavonoid content (mg/g dry weight) in (A) seed and fruit of Amomum subulatum and (B) seed and fruit of Amomum xanthioides. The data are shown as the mean ± SEM of four plants per group. b p < 0.05 versus seeds of A. xanthioides.
Owing to its anticancer, antioxidant, antimicrobial, and anti-inflammatory capabilities, gallic acid has therapeutic applications in treating multiple medical conditions including GI infections, neurological diseases, and heart disorders (Kahkeshani et al., 2019; Shukla et al., 2022). Gallic acid was reported to induce apoptosis in leukemia HL60 cell lines owing to its antitumor and anti-proliferative effects observed in multiple studies (Maruszewska and Tarasiuk, 2019; Soto-Maldonado et al., 2019; Roba’ei et al., 2022). Gallic acid also showed potent anti-obesity properties by directly targeting adipose tissue and inhibiting lipogenesis (Dludla et al., 2018), which was reported upon administration of ethyl acetate extract of A. xanthioides to high-fat-diet mouse and rat models (Kim et al., 2015; Im et al., 2020). Regarding skin diseases, gallic acid was also reported to have an immunosuppressive role in the regulation of atopic dermatitis by inhibiting the production of certain pro-inflammatory cytokines and chemokines (Tsang et al., 2016). These studies further increase the medicinal importance of A. subulatum and A. xanthioides plants as sources of natural product-based drugs since they contain considerable amounts of gallic acid.
3.5 Vitamins
While screening for vitamins, small amounts of vitamins A, B, and K were found, which did not exceed 1 mg/g dry weight, while slightly higher amounts of vitamins C and E were found in seed and fruit of both species (Figures 4A, B). Despite the overall small amounts of vitamins, seeds of A. xanthioides had significantly (p < 0.05) higher levels of vitamin E and vitamin C in seeds when compared to A. subulatum. A significant increase (p < 0.05) of vitamin E and vitamin K levels was also observed in A. xanthioides fruits than in A. subulatum fruits. Vitamin E content in plants is thought to be linked to ripening and senescence. The non-volatile substances isolated from this Amomum including vitamins were also observed (Chau et al., 2015). Plants are subjected to oxidative stress throughout senescence, which leads to a rise in fatty acid-free radicals. Enhancing the content of vitamin E during senescence may be a stress-reduction technique for plants. In addition, by collaborating with other antioxidants, vitamin E can effectively eliminate the generation of reactive oxygen species (ROS). In plants, vitamin C is the most abundant and widely distributed water-soluble cellular antioxidant. Plants can avoid oxidative damage by directly scavenging ROS with vitamin C. Furthermore, vitamin C is essential for plant growth, development, and stress responses. It functions as a cofactor for many enzymes, regulates cell division, and influences cell expansion. It also regulates plant senescence (Paciolla et al., 2019; Zhang et al., 2020). These results revealed the antioxidant effects of these plants in the DPPH, FRAP, and anti-lipid peroxidation tests.
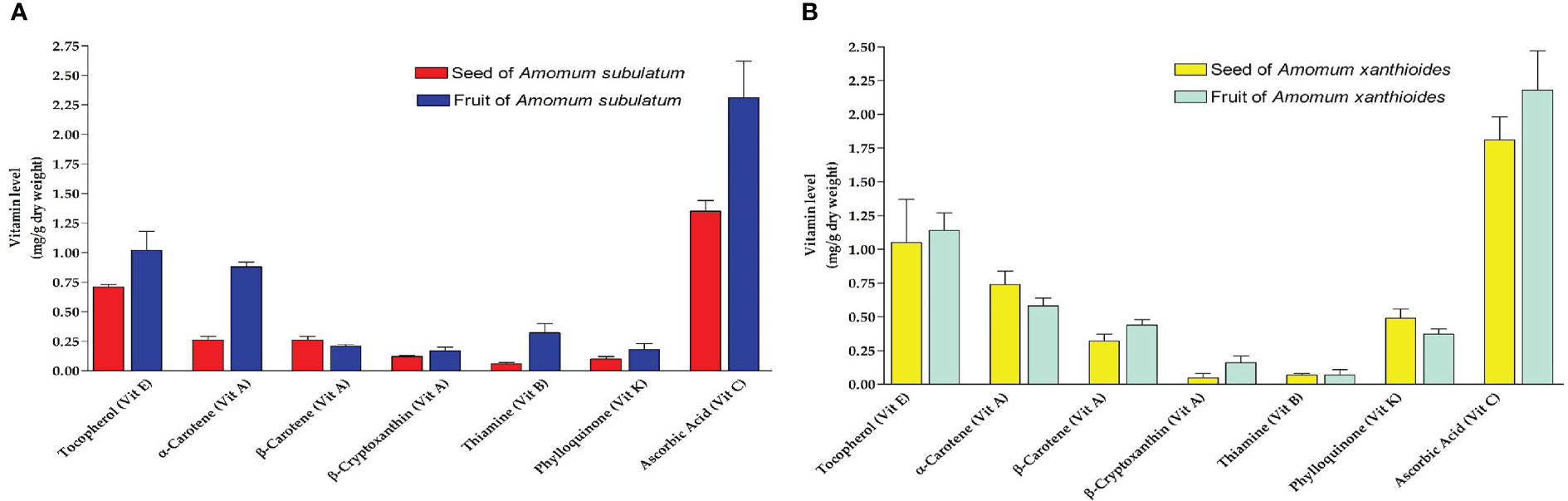
Figure 4 Vitamins levels (mg/g dry weight) isolated in (A) seed and fruit of Amomum subulatum and (B) seed and fruit of Amomum xanthioides. The data are shown as the mean ± SEM of four plants per group. a p < 0.05 versus seeds of A. subulatum, c p < 0.05 versus fruits of A. subulatum.
3.6 Amino acids
Amino acid profiling revealed that both species possess small amounts of all amino acids except for glutamine and glutamic acid, which were found in much higher amounts in both seed and fruit (Figures 5A, B). Generally, A. subulatum had higher amounts of the two amino acids than A. xanthioides. Leucine levels were significantly increased (p < 0.05) in seeds of A. subulatum compared with the fruits of the same species. A comprehensive chemical investigation found that A. tsao-ko contained a variety of chemical components including protein and amino acids (Xie et al., 2022). Among the amino acids, glutamine serves as a major amino donor for the synthesis of amino acids, nucleotides, and other nitrogen-containing compounds, in addition to its role in nutrition and metabolism (Kan et al., 2015).
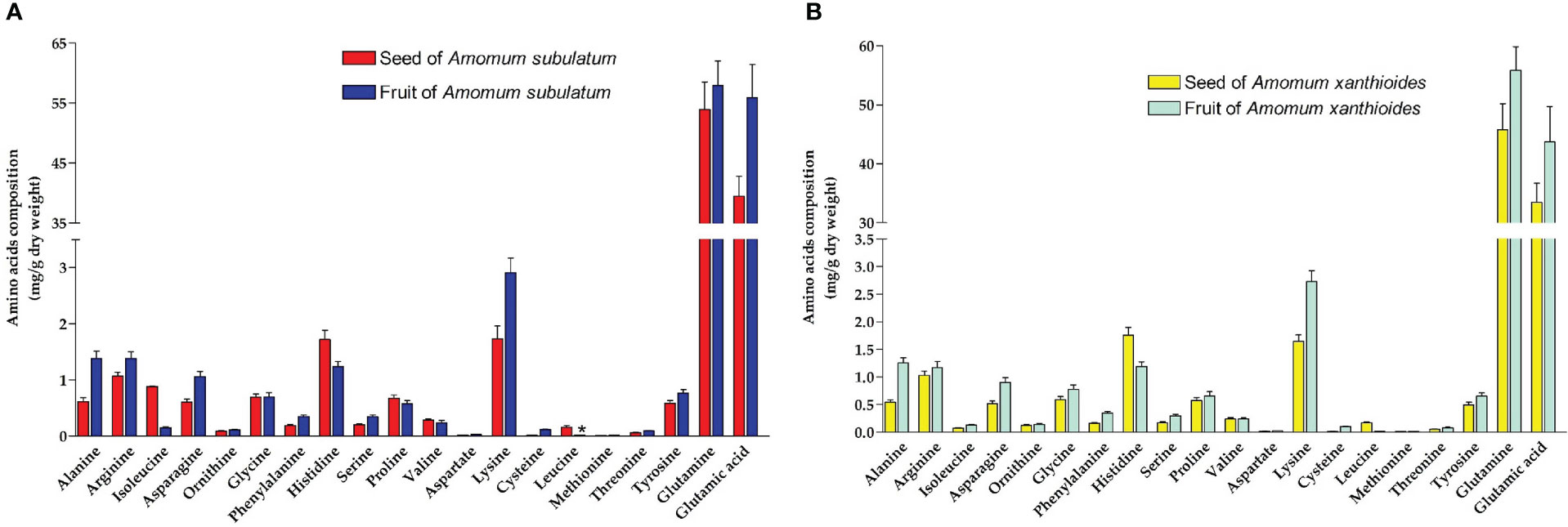
Figure 5 The amino acid compositions (mg/g dry weight) in (A) seed and fruit of Amomum subulatum and (B) seed and fruit of Amomum xanthioides. The data are shown as the mean ± SEM of four plants per group. a p < 0.05 versus seeds of A. subulatum.
3.7 Antioxidant biological activity
Multiple tests revealed the antioxidant activity of seed and fruit extracts of both species (Table 2). According to the DPPH assay, the seed extract of A. subulatum exhibited the highest antioxidant activity followed by the seed extract of A. xanthioides. Similarly, FRAP assay showed that the seed extract of the two species had the best antioxidant activity, i.e., 20.14 ± 1.11 and 21.18 ± 1.04 µmol Trolox g−1 DW for A. subulatum and A. xanthioides, respectively. In terms of anti-lipid peroxidation, higher values were obtained from the fruit extract of A. subulatum and the seed extract of A. xanthioides. No statistical significance was found between the antioxidant activity of seeds and fruits in the same plant or the two species. These results indicate the high antioxidant activity of these extracts that contributes to their usefulness in a variety of therapeutic applications. It was also observed that plants of the genus Amomum showed antioxidant and anti-inflammatory activities (Kwon et al., 2003). The presence of gallic acid and tannins may be the main contributor to the high antioxidant activity associated with A. subulatum, which was previously reported in various studies (Bhaswant et al., 2015; Gautam et al., 2016; Sharma et al., 2017). The intermediate antioxidant activity of essential oils isolated from two A. subulatum fruit samples collected from India and Saudi Arabia was formerly reported (Alam and Singh, 2021). The free radical scavenging activity at 1,000 μg/ml was approximately 85.27% in the Indian sample and 86.86% in the Saudi Arabian sample, and the IC50 values were 219.38 and 203.79 μg/ml for the Indian and Saudi Arabian samples, respectively. For A. xanthioides, antioxidant activity was previously evaluated for the water and ethanol extracts using DPPH assay, which showed that the water extract had higher antioxidant activity than the ethanolic one with IC50 values of 2.87 and 3.31 µg/ml for water and ethanol extracts, respectively (Myint et al., 2012).
3.8 Antimicrobial activity
Collectively, extracts from both species demonstrated strong antimicrobial effects against various pathogenic bacteria and fungi (Figures 6A, B). Ethanolic seed extracts of A. subulatum had the highest efficiency against four gram-negative bacterial species that cause serious human diseases, namely, Enterobacter aerogenes, Proteus vulgaris, P. aeruginosa, and Salmonella typhimurium. In addition, P. aeruginosa was also inhibited by the fruit extract of both A. subulatum and A. xanthioides. For the seed extract of A. xanthioides, large inhibition zones were formed against P. vulgaris and the fungus C. albicans. In this regard, Amomum compactum extracts damaged the membrane of fungal cells and inhibited the enzyme system of fungi to create a bland zone around the disc (Oh et al., 1951). The fruit extract of A. xanthioides caused high inhibition of Staphylococcus saprophyticus and S. typhimurium in addition to P. aeruginosa. While there were non-significant correlations between the antimicrobial activity of seeds and fruits between the two species, a statistically significant increase (p < 0.05) was observed in the antimicrobial activity of A. subulatum fruits with E. coli and Candida glabrata compared with seeds.
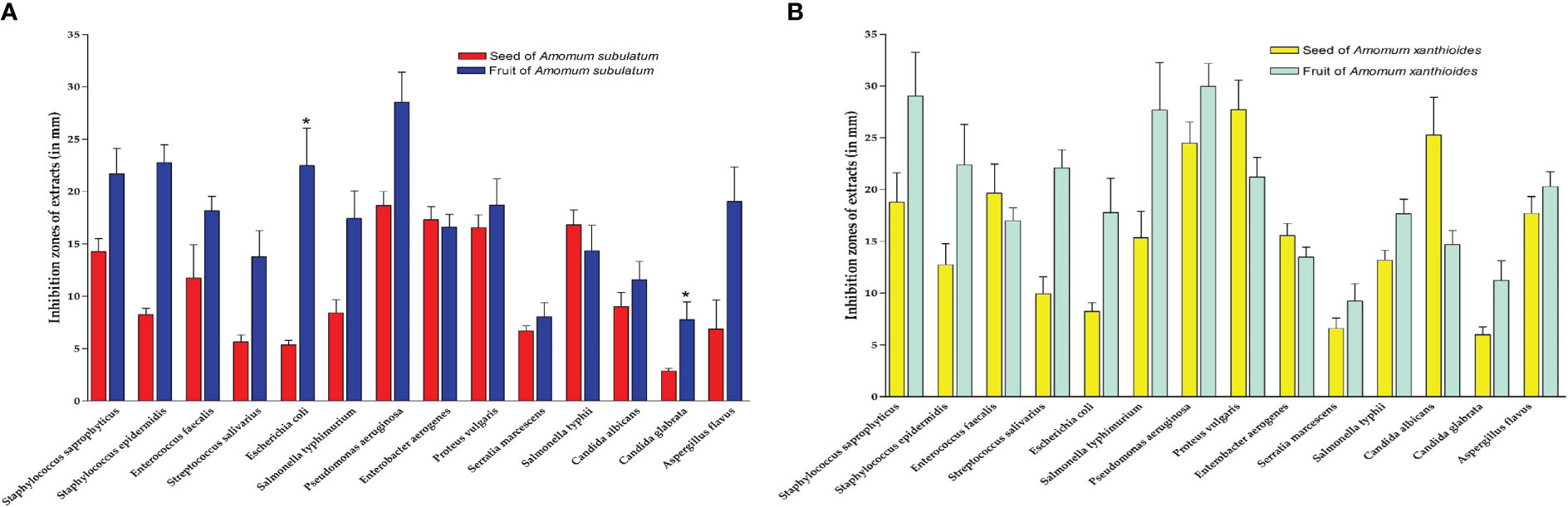
Figure 6 Inhibition zones (mm) of extracts in (A) seed and fruit of Amomum subulatum and (B) seed and fruit of Amomum xanthioides. The data are shown as the mean ± SEM of four plants per group. a p < 0.05 versus seeds of A. subulatum..
Indeed, the antimicrobial potential of both species was reported in various studies against the tested pathogens including S. aureus and A. niger (Satyal et al., 2012; Thinh et al., 2022). A concentration-dependent antibacterial activity was observed upon testing the essential oils of Indian and Saudi Arabian A. subulatum fruit samples against Acinetobacter baumannii, E. coli, and P. aeruginosa, with an inhibition zone range of 12–16 mm (Alam and Singh, 2021). In an older study, essential oils from Indian A. subulatum fruits exhibited antimicrobial activity similar to or higher than that of ciprofloxacin (as a standard) against B. pumilus, S. aureus, S. epidermidis, and P. aeruginosa (Agnihotri et al., 2012). For the seeds, methanolic seed extracts of A. subulatum exhibited reasonable antimicrobial activity at a dose of 800 mg/ml against B. subtilis, Salmonella typhii, and P. aeruginosa, when compared to tetracycline as a standard (Tijjani et al., 2012). Bacterial cell death might come from the effect of essential oil on membrane permeability, resulting in bacterial cell wall lysis (Bajpai et al., 2013). Generally, essential oils are considered the plant immune system due to their antimicrobial effect against a wide range of pathogens (Monica and Ioan, 2018). The pure form of essential oils from A. subulatum exhibited antimicrobial activity against various bacterial pathogens including E. faecalis, S. aureus, and Shigella dysenteriae forming inhibition zones of 14–23 mm (Tandukar et al., 2018). For the two species, the ratio of thyme oil was the highest among other oils in seed and fruit. In general, thyme oil and gallic acid could be the main factors promoting the antimicrobial properties of the two species due to their known antibacterial and antifungal activities and their high abundance among other oils (Borges et al., 2013; Sarjit et al., 2015; Lorenzo et al., 2019).
3.9 Molecular docking simulations
Sterol 14-alpha demethylase of the fungus C. albicans is a common target of azole drugs since it is essential in maintaining the fungus cell membrane integrity through catalyzing a vital step in ergosterol synthesis (Hargrove et al., 2017; Marek and Timmons, 2019; Zhang et al., 2019). The docking results of the proposed molecules are summarized in Table 3. The standard antifungal drug, posaconazole, formed the highest interaction with the receptor, which was indicated by the low binding affinity (−15.2 kcal/mol), followed by tocopherol (−9.6 kcal/mol) and the flavonoid kaempferol (−8.4 kcal/mol). The hydrophobicity of 3D surfaces shows that more hydrophobic interactions were formed between the ligands and the target molecules (Figure 7). Aromatic interactions visualize the most stable conformation of each ligand in the binding pocket with respect to the whole protein molecule (Figure S2). SAS structures show that a large surface area, shown in blue, is involved in the ligand binding, which increases the binding efficiency and further stabilizes the ligand in the protein binding site (Figure S3). Since the interactions mainly depended on the hydrophobic interactions, no formal charges or ionized bonds were formed, and a relatively neutral interface (white) is formed between the ligands and the receptor (Figure S4). Some molecules, including kaempferol, elemol, and glutamine, formed polar hydrogen bonds with the target receptor, which decreased the binding energy and contributed markedly to stabilizing the formed complex. The hydrogen bond donor/acceptor surface showed the polar areas (pink/green) between the ligand and the receptor due to the formation of hydrogen bonds (Figure S5). The 2D interaction diagram (Figure 8) showed the specific positions of amino acids involved in the interaction with each molecule as well as the types of chemical bonds formed between them in addition to their distances stated in Table 4.
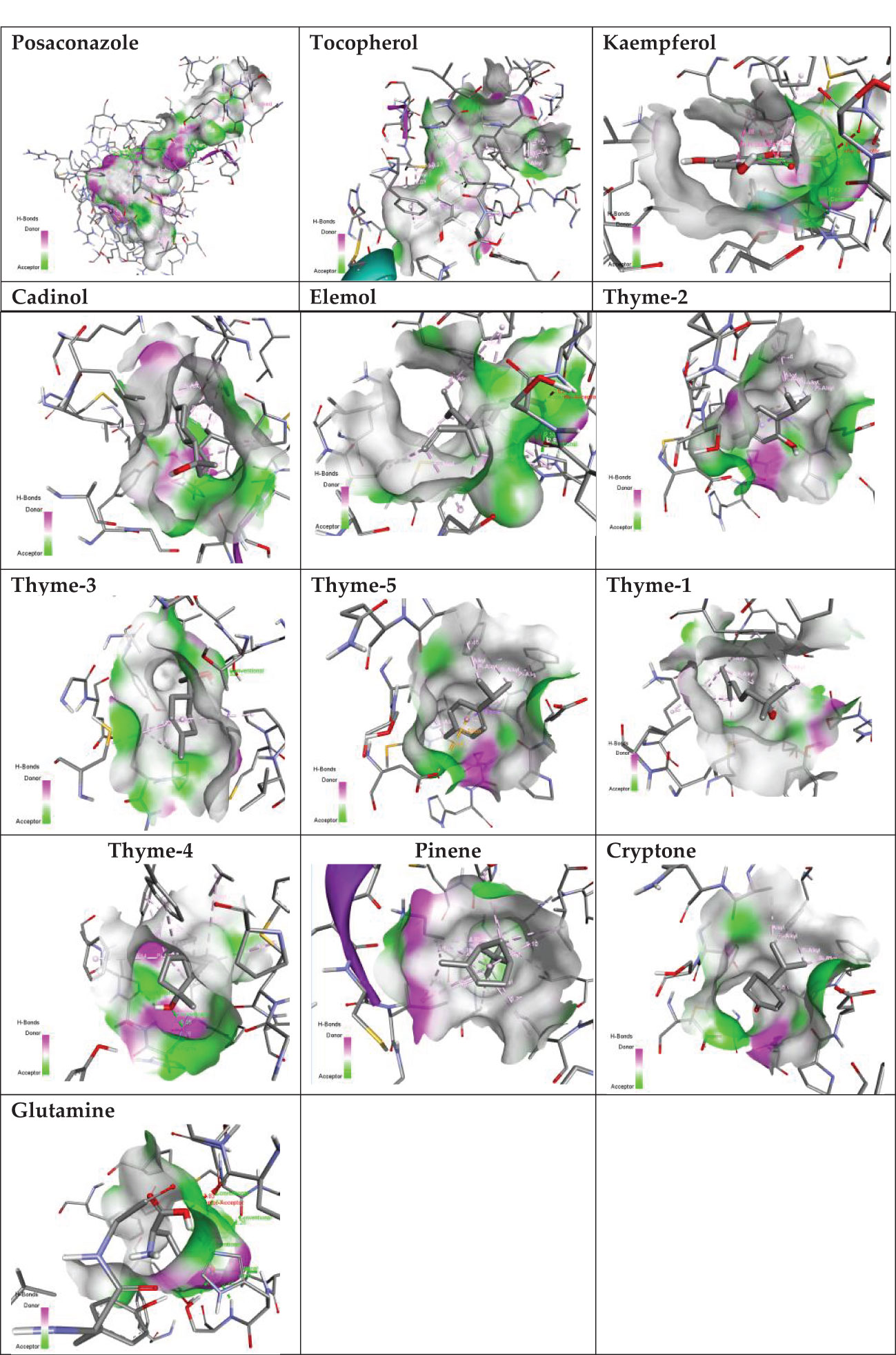
Figure 7 Hydrogen bond donor/acceptor surface view of the proposed molecules on sterol 14-alpha demethylase.
The transcriptional regulator MvfR of P. aeruginosa is responsible for the regulation of multiple virulence genes that are responsible for the pathogenicity of P. aeruginosa; therefore, it is an important drug target (Déziel et al., 2005; Allegretta et al., 2017; Zender et al., 2020). Similar to the demethylase, the interaction of the proposed molecules with the MvfR was dependent on hydrophobic interactions rather than hydrophilic ones, except for glutamine. The docking results, in Table 3, showed that tocopherol and kaempferol formed stronger and more stable complexes with the receptor than the antibacterial agent 2-heptylquinolin-4(1H)-one (HQQ), which indicates the high potency of these compounds and their promising antibacterial capabilities. The binding affinity was −8.3 and −8.2 kcal/mol for tocopherol and kaempferol, respectively, while for HQQ, it was −8 kcal/mol. In addition, more residues were involved in the hydrophobic interactions between tocopherol and MvfR, while the kaempferol–MvfR complex involved the formation of one hydrogen bond in addition to the hydrophobic one, which further stabilizes the ligand through partial charge formation. A large hydrophobic surface was observed between the ligands and the receptor (Figure S6). Aromatic surfaces show the conformation of the docked molecules responsible for the calculated binding affinity (Figure S7), and the large solvent-accessible area further confirms the stable complex (Figure S8). As expected, very low charge bonds are formed between the ligands and the receptors since the reactions relied mostly on hydrophobic interactions leading to the formation of neutral interfaces (Figure S9). The hydrogen bond formation was observed in the cases of kaempferol, cryptone, thyme-3,4, and glutamine, which are shown in the hydrogen bond donor/acceptor surface view in Figure S10. The stable ligand conformation was increased due to the formation of multiple chemical bonds with many atoms of the ligand that hold the ligand steady and make it more stable in the binding site. This was highly observed in tocopherol, cadinol, and elemol (Figures 9, 10).
4 Conclusion
This study showed the composition and the major constituents of the medicinal plants A. subulatum and A. xanthioides as well as the therapeutic functions associated with them. Both species are considered good sources of antimicrobials including gallic acid, α-pinene, and thyme oil. The most effective antibacterial agents were ethanolic seed extracts of A. subulatum against E. aerogenes, P. vulgaris, P. aeruginosa, and S. typhimurium, while the antifungal effect was demonstrated by α-pinene oil against Candida species. The strong antioxidant activity was associated with tannins and gallic acid. Gallic acid had various medicinal benefits including apoptotic and anti-proliferative effects on cancer cells, potent anti-obesity activity on fatty mice and rats, and an immunosuppressive role in the regulation of skin diseases. The anti-inflammatory potential associated with essential oils, namely, thyme and α-pinene oils, can also be employed in immunosuppressive drugs. Finally, detailed molecular modeling studies indicated that the antimicrobial activities of these plants could be attributed to the high binding affinity of their bioactive compounds to bind to the active sites of the sterol 14-alpha demethylase and the transcriptional regulator MvfR. These findings further emphasize the significance of A. subulatum and A. xanthioides plants for the formulation and production of novel, less toxic, and more efficient pharmaceuticals in the future. However, clinical and in vivo studies are needed to validate the proposed therapeutic applications.
Data availability statement
The original contributions presented in the study are included in the article/Supplementary Material. Further inquiries can be directed to the corresponding authors.
Author contributions
All authors contributed to the article and approved the submitted version.
Funding
This research work was funded by Institutional Fund Projects under grant no. (IFPIP: 621-140-1443). The authors gratefully acknowledge the technical and financial support provided by the Ministry of Education and King AbdulAziz University, DSR, Jeddah, Saudi Arabia.
Conflict of interest
The authors declare that the research was conducted in the absence of any commercial or financial relationships that could be construed as a potential conflict of interest.
Publisher’s note
All claims expressed in this article are solely those of the authors and do not necessarily represent those of their affiliated organizations, or those of the publisher, the editors and the reviewers. Any product that may be evaluated in this article, or claim that may be made by its manufacturer, is not guaranteed or endorsed by the publisher.
Supplementary material
The Supplementary Material for this article can be found online at: https://www.frontiersin.org/articles/10.3389/fpls.2023.1136961/full#supplementary-material
References
AbdElgawad, H., Peshev, D., Zinta, G., Van den Ende, W., Janssens, I. A., Asard, H. (2014). Climate extreme effects on the chemical composition of temperate grassland species under ambient and elevated CO2: a comparison of fructan and non-fructan accumulators. PloS One 9, e92044. doi: 10.1371/journal.pone.0092044
AbdElgawad, H., Saleh, A. M., Al Jaouni, S., Selim, S., Hassan, M. O., Wadaan, M. A. M., et al. (2019). Utilization of actinobacteria to enhance the production and quality of date palm (Phoenix dactylifera l.) fruits in a semi-arid environment. Sci. Total Environ. 665, 690–697. doi: 10.1016/j.scitotenv.2019.02.140
Abdel-Wahab BA, F., Abd El-Kareem, H., Alzamami, A., Fahmy, C. H., Elesawy, B., Mostafa Mahmoud, M., et al. (2022). Novel exopolysaccharide from marine bacillus subtilis with broad potential biological activities: Insights into antioxidant, anti-inflammatory, cytotoxicity, and anti-Alzheimer activity. Metabolites 12, 715. doi: 10.3390/metabo12080715
Agnihotri, S., Wakode, S. (2010). Antimicrobial activity of essential oil and various extracts of fruits of greater cardamom. Indian J. Pharm. Sci. 72, 657–659. doi: 10.4103/0250-474X.78542
Agnihotri, S. A., Wakode, S. R., Ali, M. (2012). Chemical composition, antimicrobial and topical anti-inflammatory activity of essential oil of amomum subulatum fruits. Acta Poloniae Pharm. 69, 1177–1181.
Alam, K., Pathak, D., Ansari, S. (2011). Evaluation of anti-inflammatory activity of ammomum subulatum fruit extract. Int. J. Pharm. Sci. Drug Res. 3, 35–37.
Alam, A., Singh, V. (2021). Composition and pharmacological activity of essential oils from two imported amomum subulatum fruit samples. J. Taibah Univ. Med. Sci. 16, 231–239. doi: 10.1016/j.jtumed.2020.10.007
Alkandahri, M. Y., Shafirany, M. Z., Rusdin, A., et al. (2021). Amomum compactum: A REVIEW OF PHARMACOLOGICAL STUDIES. Plant Cell Biotechnol. AND Mol. Biol. 4, 61–69. doi: 10.9734/bpi/caprd/v4/2775E
Allegretta, G., Maurer, C. K., Eberhard, J., Maura, D., Hartmann, R. W., Rahme, L., et al. (2017). In-depth profiling of MvfR-regulated small molecules in pseudomonas aeruginosa after quorum sensing inhibitor treatment. Front. Microbiol. 8, 924. doi: 10.3389/fmicb.2017.00924
Alzahrani, H. R., Kumakli, H., Ampiah, E., Mehari, T., Thornton, A. J., bbyak, C. M., et al. (2017). Determination of macro, essential trace elements, toxic heavy metal concentrations, crude oil extracts and ash composition from Saudi Arabian fruits and vegetables having medicinal values. Arabian J. Chem. 10, 906–913. doi: 10.1016/j.arabjc.2016.09.012
Ambreen, M., Mirza, S. A. (2020). Evaluation of anti-inflammatory and wound healing potential of tannins isolated from leaf callus cultures of achyranthes aspera and ocimum basilicum. Pakistan J. Pharm. Sci. 33, 361–369.
Aslam, B., Wang, W., Arshad, M. I., Khurshid, M., Muzammil, S., Rasool, M. H., et al. (2018). Antibiotic resistance: a rundown of a global crisis. Infection Drug Resist. 11, 1645. doi: 10.2147/IDR.S173867
Bajpai, V. K., Sharma, A., Baek, K.-H. (2013). Antibacterial mode of action of cudrania tricuspidata fruit essential oil, affecting membrane permeability and surface characteristics of food-borne pathogens. Food Control 32, 582–590. doi: 10.1016/j.foodcont.2013.01.032
Balunas, M. J., Kinghorn, A. D. (2005). Drug discovery from medicinal plants. Life Sci. 78, 431–441. doi: 10.1016/j.lfs.2005.09.012
Bauer, A., Brönstrup, M. (2014). Industrial natural product chemistry for drug discovery and development. Natural product Rep. 31, 35–60. doi: 10.1039/C3NP70058E
Bhaswant, M., Poudyal, H., Mathai, M. L., Ward, L. C., Mouatt, P., Brown, L. (2015). Green and black cardamom in a diet-induced rat model of metabolic syndrome. Nutrients 7, 7691–7707. doi: 10.3390/nu7095360
Bhatia, S. P., Letizia, C. S., Api, A. M. (2008). Fragrance material review on elemol. Food Chem. Toxicol. 46, S147–S148. doi: 10.1016/j.fct.2008.06.045
Borges, A., Ferreira, C., Saavedra, M. J., Simões, M. (2013). Antibacterial activity and mode of action of ferulic and gallic acids against pathogenic bacteria. Microb. Drug Resist. 19, 256–265. doi: 10.1089/mdr.2012.0244
Chau, L. T. M., Thang, T. D., Huong, L. T., Ogunwande, I. A. (2015). Constituents of essential oils from amomum longiligulare from Vietnam. Chem. Nat. Compd. 51, 1181–1183. doi: 10.1007/s10600-015-1525-z
Choi, Y., Choi, J. K., Jang, Y. H., Lee, S., Lee, S., Choi, J. H., et al. (2017). Anti−inflammatory effect of amomum xanthioides in a mouse atopic dermatitis model. Mol. Med. Rep. 16, 8964–8972. doi: 10.3892/mmr.2017.7695
Clark, J., Switzer, R. (1977). Isolation and characterization of phosphatidyl choline from egg yolks. Exp. Biochem., 187–190. doi: 10.1080/10826076.2014.962148
Corsalini, M., Inchingolo, F., Dipalma, G., Wegierska, A. E., Charitos, I. A., Potenza, M. A., et al. (2021). Botulinum neurotoxins (BoNTs) and their biological, pharmacological, and toxicological issues: A scoping review. Appl. Sci. 11, 8849. doi: 10.3390/app11198849
Cowan, M. M. (1999). Plant products as antimicrobial agents. Clin. Microbiol. Rev. 12, 564–582. doi: 10.1128/CMR.12.4.564
Davies, J. (1994). Inactivation of antibiotics and the dissemination of resistance genes. Science 264, 375–382. doi: 10.1126/science.8153624
De, A. K., De, M. (2019). “Functional and therapeutic applications of some important spices,” in The Role of Functional Food Security in Global Health. 2019, 499–510. doi: 10.1016/B978-0-12-813148-0.00029-3
Delesa, D. A. (2018). Traditional medicinal plants for industrial application as natural food preservatives. Int. J. Adv. Res. Biol. Sci. 5, 85–94. doi: 10.26655/ijabbr.2018.1.1
Déziel, E., Gopalan, S., Tampakaki, A. P., et al. (2005). The contribution of MvfR to pseudomonas aeruginosa pathogenesis and quorum sensing circuitry regulation: multiple quorum sensing-regulated genes are modulated without affecting lasRI, rhlRI or the production of n-acyl-L-homoserine lactones. Mol. Microbiol. 55, 998–1014. doi: 10.1111/j.1365-2958.2004.04448.x
Dhakal, R., Dihingia, A., Ahmed, R. S., Gupta, D. D., Sahu, R. K., Dutta, P., et al. (2022). Prophylactic and therapeutic potential of active phytoconstituents from amomum subulatum roxb. Food Front. 4 (1), 60–84. doi: 10.1002/fft2.184
Diao, W.-R., Zhang, L.-L., Feng, S.-S., Xu, J.-G. (2014). Chemical composition, antibacterial activity, and mechanism of action of the essential oil from amomum kravanh. J. Food Prot. 77, 1740–1746. doi: 10.4315/0362-028X.JFP-14-014
Dludla, P. V., Nkambule, B. B., Jack, B., Mkandla, Z., Mutize, T., Silvestri, S., et al. (2018). Inflammation and oxidative stress in an obese state and the protective effects of gallic acid. Nutrients 11, 23. doi: 10.3390/nu11010023
El Azab, I. H., Saied, E. M., Osman, A. A., Mehana, A. E., Saad, H. A., Elkanzi, N. A. (2021). Novel n-bridged pyrazole-1-carbothioamides with potential antiproliferative activity: design, synthesis, in vitro and in silico studies. Future Medicinal Chem. 13, 1743–1766. doi: 10.4155/fmc-2021-0066
Famiani, F., Bonghi, C., Chen, Z.-H., Drincovich, M. F., Farinelli, D., Lara, M. V., et al. (2020). Stone fruits: Growth and nitrogen and organic acid metabolism in the fruits and seeds–a review. Front. Plant Sci. 11. doi: 10.3389/fpls.2020.572601
Gaber, A., Alsanie, W. F., Kumar, D. N., Refat, M. S., Saied, E. M. (2020). Novel papaverine metal complexes with potential anticancer activities. Molecules 25, 5447. doi: 10.3390/molecules25225447
Gaber, A., Refat, M. S., Belal, A. A. M., El-Deen, I. M., Hassan, N., Zakaria, R., et al. (2021). New mononuclear and binuclear Cu(II), Co(II), Ni(II), and Zn(II) thiosemicarbazone complexes with potential biological activity: Antimicrobial and molecular docking study. Molecules 26, 2288. doi: 10.3390/molecules26082288
Gautam, N., Bhattarai, R. R., Khanal, B. K. S., Oli, P. (2016). Technology, chemistry and bioactive properties of large cardamom (Amomum subulatum roxb.): An overview. Int. J. Appl. Sci. Biotechnol. 4, 139–149. doi: 10.3126/ijasbt.v4i2.15104
Grover, J. K., Yadav, S., Vats, V. (2002). Medicinal plants of India with anti-diabetic potential. J. Ethnopharmacology 81, 81–100. doi: 10.1016/S0378-8741(02)00059-4
Hargrove, T. Y., Friggeri, L., Wawrzak, Z., Qi, A., Hoekstra, W. J., Schotzinger, R. J., et al. (2017). Structural analyses of candida albicans sterol 14α-demethylase complexed with azole drugs address the molecular basis of azole-mediated inhibition of fungal sterol biosynthesis. J. Biol. Chem. 292, 6728–6743. doi: 10.1074/jbc.M117.778308
Hassan, M. O., Saleh, A. M., AbdElgawad, H. (2018). Sonchus oleraceus residue improves nutritive and health-promoting value of common bean (Phaseolus vulgaris l.): A metabolic study. J. Agric. Food Chem. 66, 2092–2100. doi: 10.1021/acs.jafc.7b05821
Healey, R. D., Saied, E. M., Cong, X., Karsai, G., Gabellier, L., Saint-Paul, J., et al. (2022). Discovery and mechanism of action of small molecule inhibitors of ceramidases**. Angewandte Chemie Int. Edition 61, e202109967. doi: 10.1002/anie.202109967
Hussien, Z. N., Ibrahim, M. M. (2020). Integrated management of onion pink root rot caused by setophoma terrestris. Middle East J. Appl. Sci. 10, 703–714. doi: 10.36632/mejas/2020.10.4.62
Im, H.-J., Hwang, S.-J., Lee, J.-S., Lee, S.-B., Kang, J.-Y., Son, C.-G. (2020). Ethyl acetate fraction of amomum xanthioides ameliorates nonalcoholic fatty liver disease in a high-fat diet mouse model. Nutrients 12, 2433. doi: 10.3390/nu12082433
Itkin, M., Heinig, U., Tzfadia, O., Bhide, A. J., Shinde, B., Cardenas, P. D., et al. (2013). Biosynthesis of antinutritional alkaloids in solanaceous crops is mediated by clustered genes. Science 341, 175–179. doi: 10.1126/science.1240230
Jakob, E., Elmadfa, I. (1996). Application of a simplified HPLC assay for the determination of phylloquinone (vitamin K1) in animal and plant food items. Food Chem. 56, 87–91. doi: 10.1016/0308-8146(95)00150-6
Kahkeshani, N., Farzaei, F., Fotouhi, M., Alavi, S. S., Bahramsoltani, R., Naseri, R., et al. (2019). Pharmacological effects of gallic acid in health and disease: A mechanistic review. Iranian J. Basic Med. Sci. 22, 225–237. doi: 10.22038/ijbms.2019.32806.7897
Kan, C.-C., Chung, T.-Y., Juo, Y.-A., Hsieh, M.-H. (2015). Glutamine rapidly induces the expression of key transcription factor genes involved in nitrogen and stress responses in rice roots. BMC Genomics 16, 731. doi: 10.1186/s12864-015-1892-7
Khirallah, S. M., Ramadan, H. M. M., Aladl, H. A. A., Ayaz, N. O., Kurdi, L. A.F., Jaremko, M., et al. (2022a). Antidiabetic potential of novel 1,3,5-Trisubstituted-2-Thioxoimidazloidin-4-One analogues: Insights into α-glucosidase, α-amylase, and antioxidant activities. Pharmaceuticals 15, 1576. doi: 10.3390/ph15121576
Khirallah, S. M., Ramadan, H. M. M., Shawky, A., Qahl, S. H., Baty, R. S., Alqadri, N., et al. (2022b). Development of novel 1,3-Disubstituted-2-Thiohydantoin analogues with potent anti-inflammatory activity; In vitro and in silico assessments. Molecules 27, 6271. doi: 10.3390/molecules27196271
Kim, H.-G., Han, J.-M., Lee, J.-S., Suk Lee, J., Son, C.-G. (2015). Ethyl acetate fraction of amomum xanthioides improves bile duct ligation-induced liver fibrosis of rat model via modulation of pro-fibrogenic cytokines. Sci. Rep. 5, 1–11. doi: 10.1038/srep14531
Konappa, N., Udayashankar, A. C., Krishnamurthy, S., Pradeep, C. K., Chowdappa, S., and Jogaiah, S., et al. (2020). GC–MS analysis of phytoconstituents from amomum nilgiricum and molecular docking interactions of bioactive serverogenin acetate with target proteins. Sci. Rep. 10, 16438. doi: 10.1038/s41598-020-73442-0
Kumar, A., Goyal, R., Kumar, S., Jain, S., Jain, N., Kumar, P. (2015). Estrogenic and anti-alzheimer’s studies of zingiber officinalis as well as amomum subulatum roxb.: the success story of dry techniques. Med. Chem. Res. 24, 1089–1097. doi: 10.1007/s00044-014-1166-y
Kwon, K.-B., Kim, J.-H., Lee, Y.-R., Lee, H.-K., Jeong, Y.-J., Rho, H.-W., et al. (2003). Amomum xanthoides extract prevents cytokine-induced cell death of RINm5F cells through the inhibition of nitric oxide formation. Life Sci. 73, 181–191. doi: 10.1016/s0024-3205(03)00267-4
Lai, L.-R., Hsieh, S.-C., Huang, H.-Y., Chou, C.-C. (2013). Effect of lactic fermentation on the total phenolic, saponin and phytic acid contents as well as anti-colon cancer cell proliferation activity of soymilk. J. bioscience bioengineering 115, 552–556. doi: 10.1016/j.jbiosc.2012.11.022
Lamxay, V., Newman, M. (2012). A revision of amomum (Zingiberaceae) in cambodia, laos and vietnam. Edinburgh J. Bot. 69, 99–206. doi: 10.1017/S0960428611000436
Li, G., Lu, Q., Wang, J., Hu, Q., Liu, P., Yang, Y., et al. (2021). Correlation analysis of compounds in essential oil of amomum tsaoko seed and fruit morphological characteristics, geographical conditions, locality of growth. Agronomy 11, 744. doi: 10.3390/agronomy11040744
Lorenzo, J. M., Mousavi Khaneghah, A., Gavahian, M., Marszałek, K., Eş, I., Munekata, P. E., et al. (2019). Understanding the potential benefits of thyme and its derived products for food industry and consumer health: From extraction of value-added compounds to the evaluation of bioaccessibility, bioavailability, anti-inflammatory, and antimicrobial activities. Crit. Rev. Food Sci. Nutr. 59, 2879–2895. doi: 10.1080/10408398.2018.1477730
Lv, F., Liang, H., Yuan, Q., Li, C. (2011). In vitro antimicrobial effects and mechanism of action of selected plant essential oil combinations against four food-related microorganisms. Food Res. Int. 44, 3057–3064. doi: 10.1016/j.foodres.2011.07.030
Makhija, P., Handral, H. K., Mahadevan, G., Kathuria, H., Sethi, G., Grobben, B. (2022). Black cardamom (Amomum subulatum roxb.) fruit extracts exhibit apoptotic activity against lung cancer cells. J. Ethnopharmacology 287, 114953. doi: 10.1016/j.jep.2021.114953
Marek, C. L., Timmons, S. R. (2019). “9 - antimicrobials in pediatric dentistry,” in Pediatric dentistry, Sixth Edition. Eds. Nowak, A. J., Christensen, J. R., Mabry, T. R., Townsend, J. A., Wells, M. H. (Philadelphia: Elsevier), 128–141.e1. doi: 10.1016/B978-0-323-60826-8.00009-2
Maruszewska, A., Tarasiuk, J. (2019). Antitumour effects of selected plant polyphenols, gallic acid and ellagic acid, on sensitive and multidrug-resistant leukaemia HL60 cells. Phytotherapy Res. 33, 1208–1221. doi: 10.1002/ptr.6317
McGregor, J. C., Quach, Y., Bearden, D. T., Smith, D. H., Sharp, S. E., Guzman-Cottrill, J. A. (2014). Variation in antibiotic susceptibility of uropathogens by age among ambulatory pediatric patients. J. Pediatr. Nurs. 29, 152–157. doi: 10.1016/j.pedn.2013.09.001
Mohamed, D. I., Abou-Bakr, D. A., Ezzat, S. F., El-Kareem, H. F. A., Nahas, H. H. A., Saad, H. A., et al. (2021). Vitamin D3 prevents the deleterious effects of testicular torsion on testis by targeting miRNA-145 and ADAM17: In silico and In vivo study. Pharmaceuticals 14, 1222. doi: 10.3390/ph14121222
Mohamed, D. I., Alaa El-Din Aly El-Waseef, D., Nabih, E. S., El-Kharashi, O. A., Abd El-Kareem, H. F., Abo Nahas, H. H., et al. (2022a). Acetylsalicylic acid suppresses alcoholism-induced cognitive impairment associated with atorvastatin intake by targeting cerebral miRNA155 and NLRP3: In vivo, and in silico study. Pharmaceutics 14, 529. doi: 10.3390/pharmaceutics14030529
Mohamed, D. I., Ezzat, S. F., Elayat, W. M., El-Kharashi, O. A., El-Kareem, H. F. A., Nahas, H. H. A., et al. (2022b). Hepatoprotective role of carvedilol against ischemic hepatitis associated with acute heart failure via targeting miRNA-17 and mitochondrial dynamics-related proteins: An In vivo and in silico study. Pharmaceuticals 15, 832. doi: 10.3390/ph15070832
Monica, B., Ioan, S. (2018). Essential oils from plants. J. Biotechnol. Biomed. Sci. 1, 35–43. doi: 10.14302/issn.2576-6694.jbbs-18-2489
Morris, G. M., Huey, R., Lindstrom, W., Sanner, M. F., Belew, R. K., Goodsell, D. S., et al. (2009). AutoDock4 and AutoDockTools4: Automated docking with selective receptor flexibility. J. Comput. Chem. 30, 2785–2791. doi: 10.1002/jcc.21256
Mueller-Harvey, I. (2006). Unravelling the conundrum of tannins in animal nutrition and health. J. Sci. Food Agric. 86, 2010–2037. doi: 10.1002/jsfa.2577
Myint, P. P., Thu, K., Win, K. C., Win, N. N., Ngwe, D. H. (2012). A study of antidiabetic and antioxidant activities of amomum xanthioides Wall.(Chinbaung hpalar) seed. Universities Res. J. 5(4), 19–32.
Nóbrega, J. R., Silva D de, F., de Andrade Júnior, F. P., de Sousa, P. M.S., Figueiredo, P. T.R., Cordeiro, L. V., et al. (2021). Antifungal action of α-pinene against candida spp. isolated from patients with otomycosis and effects of its association with boric acid. Natural Product Res. 35, 6190–6193. doi: 10.1080/14786419.2020.1837803
Oh, L., Nj, R., Al, F., Rj, R. (1951). Protein measurement with the folin phenol reagent. J. Biol. Chem. 193(1), 265–275.
Ohkawa, H., Ohishi, N., Yagi, K. (1979). Assay for lipid peroxides in animal tissues by thiobarbituric acid reaction. Analytical Biochem. 95, 351–358. doi: 10.1016/0003-2697(79)90738-3
Paciolla, C., Fortunato, S., Dipierro, N., Paradiso, A., De Leonardis, S., Mastropasqua, L., et al. (2019). Vitamin c in plants: From functions to biofortification. Antioxidants (Basel) 8, 519. doi: 10.3390/antiox8110519
Pan, X., Xiao, H., Hu, X., Liu, Z. L. (2022). Insecticidal activities of the essential oil of rhynchanthus beesianus rhizomes and its constituents against two species of grain storage insects. Z. für Naturforschung C. 78(1-2), 83–89.
Pandey, A. K., Kumar, P., Singh, P., Tripathi, N. N., Bajpai, V. K. (2017). Essential oils: Sources of antimicrobials and food preservatives. Front. Microbiol. 7, 2161. doi: 10.3389/fmicb.2016.02161
Park, M., Cho, H., Jung, H., Lee, H., Hwang, K. T. (2014). Antioxidant and anti-inflammatory activities of tannin fraction of the extract from black raspberry seeds compared to grape seeds. J. Food Biochem. 38, 259–270. doi: 10.1111/jfbc.12044
Pešić, M., Stanković, S. (2015). Development of natural product drugs in a sustainable manner. Brief United Nations Global Sustain. Dev. Rep.
Prado-Audelo, D., Luisa, M., Cortés, H., Caballero-Florán, I. H., González-Torres, M., Escutia-Guadarrama, L., et al. (2021). Therapeutic applications of terpenes on inflammatory diseases. Front. Pharmacol. 2114. doi: 10.3389/fphar.2021.704197
Prasathkumar, M., Anisha, S., Dhrisya, C., Becky, R., Sadhasivam, S. (2021). Therapeutic and pharmacological efficacy of selective Indian medicinal plants – a review. Phytomedicine Plus 1, 100029. doi: 10.1016/j.phyplu.2021.100029
Rachkeeree, A., Kantadoung, K., Suksathan, R., Puangpradab, R., Page, P. A., Sommano, S. R. (2018). Nutritional compositions and phytochemical properties of the edible flowers from selected zingiberaceae found in Thailand. Front. Nutr. 5. doi: 10.3389/fnut.2018.00003
Roba’ei, D. R. M., Osman, W. N. W., Zainudin, H. Z. M., Aziz, M. Y. A., Johari, S. A. T. T. (2022). Anti-proliferative and apoptosis inducing effects of Gallic acid on human acute myeloid leukaemia cell lines (HL-60). Asian J. Med. Biomedicine 6, 160–162. doi: 10.37231/ajmb.2022.6.S1.572
Saied, E. M., El-Maradny, Y. A., Osman, A. A., Darwish, A. M. G., Abo Nahas, H. H., Niedbała, G., et al. (2021). A comprehensive review about the molecular structure of severe acute respiratory syndrome coronavirus 2 (SARS-CoV-2): Insights into natural products against COVID-19. Pharmaceutics 13, 1759. doi: 10.3390/pharmaceutics13111759
Saleem, M., Deters, B., de la Bastide, A., Korzen, M. (2019). Antibiotics overuse and bacterial resistance. Ann. Microbiol. Res. 3, 93.
Saleh, A. M., Madany, M. M. Y. (2015). Coumarin pretreatment alleviates salinity stress in wheat seedlings. Plant Physiol. Biochem. 88, 27–35. doi: 10.1016/j.plaphy.2015.01.005
Salehi, B., Mishra, A. P., Shukla, I., Sharifi‐Rad, M., Contreras, M. M., Segura‐Carretero, A., et al. (2018). Thymol, thyme, and other plant sources: Health and potential uses. Phytotherapy Res. 32, 1688–1706. doi: 10.1002/ptr.6109
Salehi, B., Upadhyay, S., Erdogan Orhan, I., Kumar Jugran, A., Jayaweera, L. D S., Dias, A. D., et al. (2019). Therapeutic potential of α-and β-pinene: A miracle gift of nature. Biomolecules 9, 738. doi: 10.3390/biom9110738
Samaha, D., Hamdo, H. H., Cong, X., Schumacher, F., Banhart, S., Aglar, Ö., et al. (2020). Liposomal FRET assay identifies potent drug-like inhibitors of the ceramide transport protein (CERT). Chem. – A Eur. J. 26, 16616–16621. doi: 10.1002/chem.202003283
Santos, E. F., Mateus, N. S., Rosário, M. O., Garcez, T. B., Mazzafera, P., Lavres, J. (2021). Enhancing potassium content in leaves and stems improves drought tolerance of eucalyptus clones. Physiologia Plantarum 172, 552–563. doi: 10.1111/ppl.13228
Sarjit, A., Wang, Y., Dykes, G. A. (2015). Antimicrobial activity of gallic acid against thermophilic campylobacter is strain specific and associated with a loss of calcium ions. Food Microbiol. 46, 227–233. doi: 10.1016/j.fm.2014.08.002
Sarungallo, Z. L., Hariyadi, P., Andarwulan, N., Purnomo, E. H., Wada, M. (2015). Analysis of α-cryptoxanthin, β-cryptoxanthin, α -carotene, and β-carotene of pandanus conoideus oil by high-performance liquid chromatography (HPLC). Proc. Food Sci. 3, 231–243. doi: 10.1016/j.profoo.2015.01.026
Satyal, P., Dosoky, N. S., Kincer, B. L., Setzer, W. N. (2012). Chemical compositions and biological activities of amomum subulatum essential oils from Nepal. Nat. Prod. Commun. 7, 1233–1236. doi: 10.1177/1934578X1200700935
Sett, R., Soni, B. (2013). Foliar nitrogen, phosphorus and potassium content in trees in environmentally toxic plastic industry area. J. Environ. Sci. Eng. 55, 167–174.
Sharma, K., Kumar, V., Kaur, J., Tanwar, B., Goyal, A., Sharma, R., et al. (2021). Health effects, sources, utilization and safety of tannins: A critical review. Toxin Rev. 40, 432–444. doi: 10.1080/15569543.2019.1662813
Sharma, V., Lohia, N., Handa, V., Baranwal, M. (2017). Amomum subulatum seed extract exhibit antioxidant, cytotoxic and immune-suppressive effect. Indian J. Biochem. Biophys. 54, 135–139.
Shukla, S., Singh, B., Singh, A., Singh, C. (2022). Emerging and advanced drug delivery systems for improved biopharmaceutical attributes of gallic acid: A review. Phytomedicine Plus 2, 100369. doi: 10.1016/j.phyplu.2022.100369
Soto-Maldonado, C., Vergara-Castro, M., Jara-Quezada, J., Caballero-Valdés, E., Müller-Pavez, A., Zúñiga-Hansen, M. E., et al. (2019). Polyphenolic extracts of walnut (Juglans regia) green husk containing juglone inhibit the growth of HL-60 cells and induce apoptosis. Electronic J. Biotechnol. 39, 1–7. doi: 10.1016/j.ejbt.2019.02.001
Sreevidya, N., Mehrotra, S. (2003). Spectrophotometric method for estimation of alkaloids precipitable with dragendorff’s reagent in plant materials. J. AOAC Int. 86, 1124–1127. doi: 10.1093/jaoac/86.6.1124
Sudarsanan, D., Suresh Sulekha, D., Chandrasekharan, G. (2021). Amomum subulatum induces apoptosis in tumor cells and reduces tumor burden in experimental animals via modulating pro-inflammatory cytokines. Cancer Invest. 39, 333–348. doi: 10.1080/07357907.2021.1878529
Tampe, J., Espinoza, J., Chacón-Fuentes, M., Quiroz, A., Rubilar, M. (2020). Evaluation of drimys winteri (Canelo) essential oil as insecticide against acanthoscelides obtectus (Coleoptera: Bruchidae) and aegorhinus superciliosus (Coleoptera: Curculionidae). Insects 11, 335. doi: 10.3390/insects11060335
Tandukar, U., Lakhey, P. B., Shrestha, N., Dhungana, A., Bhatt, T. D. (2018). Antimicrobial activity and chemical composition of essential oil of fruits of amomum subulatum roxb. J. OF Plant Resour. 16, 57.
Thinh, B. B., Chac, L. D., Hanh, D. H., Korneeva, A. A., Hung, N., Igoli, J. O. (2022). Effect of extraction method on yield, chemical composition and antimicrobial activity of essential oil from the fruits of amomum villosum var. xanthioides. J. Essential Oil Bearing Plants 25, 28–37. doi: 10.1080/0972060X.2022.2049893
Thinh, B. B., Doudkin, R. V., Thanh, V. Q. (2021). Chemical composition of essential oil of amomum xanthioides wall. ex baker from northern Vietnam. Biointerface Res. Appl. Chem. 11, 12275–12284. doi: 10.33263/BRIAC114.1227512284
Thompson, T. (2022). The staggering death toll of drug-resistant bacteria. Nature. doi: 10.1038/d41586-022-00228-x
Tijjani, M., Abdulrahman, F., Sandabe, U. (2011). Chemical composition and anti-nociceptive effects of the leaf extract of vitexdoniana sweet. J. Chem. Soc. Nigeria 36, 213–219.
Tijjani, M. A., Dimari, G. A., Buba, S. W., Khan, I. Z. (2012). In-vitro antibacterial properties and pre-liminary phtytochemical analysis of amomum subulatum roxburg (Large cardamom). J. Appl. Pharm. Sci., 69–73. doi: 10.7324/JAPS.2012.2511
Tong, Z., He, W., Fan, X., Guo, A. (2021). Biological function of plant tannin and its application in animal health. Front. Veterinary Sci. 8.
Trott, O., Olson, A. J. (2010). AutoDock vina: improving the speed and accuracy of docking with a new scoring function, efficient optimization, and multithreading. J. Comput. Chem. 31, 455–461. doi: 10.1002/jcc.21334
Tsang, M. S., Jiao, D., Chan, B. C., Hon, K.-L., Leung, P. C., Lau, C. B., et al. (2016). Anti-inflammatory activities of pentaherbs formula, berberine, gallic acid and chlorogenic acid in atopic dermatitis-like skin inflammation. Molecules 21, 519. doi: 10.3390/molecules21040519
Ventola, C. L. (2015). The antibiotic resistance crisis: part 1: causes and threats. Pharm. Ther. 40, 277.
Wangrawa, D. W., Kosgei, J., Machani, M., Opala, J., Agumba, S., Yaméogo, F., et al. (2022). Larvicidal activities and synergistic effects of essential oils against anopheles funestus and culex quinquefasciatus (Diptera: Culicidae) from kisumu, Kenya. Psyche: A J. Entomology. doi: 10.1155/2022/8302696
WHO. Global Centre for Traditional Medicine in India (n.d.). Avaialble at: https://www.who.int/news/item/25-03-2022-who-establishes-the-global-centre-for-traditional-medicine-in-india (Accessed November 6, 2022).
Xie, L., Yu, D., Li, Y., Ju, H., Chen, J., Hu, L., et al. (2022). Characterization, hypoglycemic activity, and antioxidant activity of methanol extracts from amomum tsao-ko: in vitro and in vivo studies. Front. Nutr. 9. doi: 10.3389/fnut.2022.869749
Yahaya, A. A. H., Salleh, W. M. N. H. W., Ab Ghani, N., Khamis, S., Rezali, N. S., Juhari, M. A. A. (2022). Chemical composition and bioactivities of magnolia candollii h. keng essential oil. Z. für Naturforschung C 77, 519–523. doi: 10.1515/znc-2022-0100
Yousaf, M., Bashir, S., Raza, H., Shah, A. N., Iqbal, J., Arif, M., et al. (2021). Role of nitrogen and magnesium for growth, yield and nutritional quality of radish. Saudi J. Biol. Sci. 28, 3021–3030. doi: 10.1016/j.sjbs.2021.02.043
Yousefzadi, M., Sonboli, A., Karimi, F., Ebrahimi, S. N., Asghari, B., Zeinali, A. (2007). Antimicrobial activity of some salvia species essential oils from Iran. Z. für Naturforschung C 62, 514–518. doi: 10.1515/znc-2007-7-809
Zarzuelo, A., Crespo, E. (2002). The medicinal and non-medicinal uses of thyme. In: Thyme. CRC Press pp, 277–306.
Zender, M., Witzgall, F., Kiefer, A., Kirsch, B., Maurer, C. K., Kany, A. M., et al. (2020). Flexible fragment growing boosts potency of quorum-sensing inhibitors against pseudomonas aeruginosa virulence. ChemMedChem 15, 188–194. doi: 10.1002/cmdc.201900621
Zhang, J., Li, L., Lv, Q., Yan, L., Wang, Y., Jiang, Y. (2019). The fungal CYP51s: Their functions, structures, related drug resistance, and inhibitors. Front. Microbiol. 10, 691. doi: 10.3389/fmicb.2019.00691
Keywords: phytochemical analysis, natural products, medicinal plants, Amomum subulatom, Amomum xanthioides, antioxidant activity, antimicrobial activities, molecular docking
Citation: Alruhaili MH, Almuhayawi MS, Gattan HS, Alharbi MT, Nagshabandi MK, Jaouni SKA, Selim S and AbdElgawad H (2023) Insight into the phytochemical profile and antimicrobial activities of Amomum subulatum and Amomum xanthioides: an in vitro and in silico study. Front. Plant Sci. 14:1136961. doi: 10.3389/fpls.2023.1136961
Received: 03 January 2023; Accepted: 23 March 2023;
Published: 20 April 2023.
Edited by:
Rajesh Chandra Misra, John Innes Centre, United KingdomReviewed by:
Kanika Sharma, Central Institute for Research on Cotton Technology (CIRCOT), IndiaArif Jamal Siddiqui, University of Hail, Saudi Arabia
Copyright © 2023 Alruhaili, Almuhayawi, Gattan, Alharbi, Nagshabandi, Jaouni, Selim and AbdElgawad. This is an open-access article distributed under the terms of the Creative Commons Attribution License (CC BY). The use, distribution or reproduction in other forums is permitted, provided the original author(s) and the copyright owner(s) are credited and that the original publication in this journal is cited, in accordance with accepted academic practice. No use, distribution or reproduction is permitted which does not comply with these terms.
*Correspondence: Mohammed H. Alruhaili, bWFscnVoYWlsaUBrYXUuZWR1LnNh; Samy Selim, c2FiZHVsc2FsYW1AanUuZWR1LnNh; Hamada AbdElgawad, aGFtYWRhLmFiZEVsZ2F3YWRAdWFudHdlcnBlbi5iZQ==