- 1State Forestry and Grassland Administration Key Laboratory of Silviculture in Downstream Areas of the Yellow River, Forestry College of Shandong Agricultural University, Tai’an, Shandong, China
- 2Shandong Academy of Forestry Sciences, Jinan, Shandong, China
Secondary trunk Ginkgo biloba is one of the specific germplasms of G. biloba. In this study, paraffin sectioning, high-performance liquid chromatography and transcriptome sequencing technology were used to study the development of the secondary trunk of G. biloba from the morphological, physiological and molecular levels. The results showed that the secondary trunk of G. biloba originated from the latent buds in the stem cortex at the junction of the root and stem of the main trunk. The development process of secondary trunk was divided into 4 periods: the dormancy period of the secondary trunk buds, the differentiation period, the formation period of transport tissue, and the budding period. Transcriptome sequencing was performed by comparing the germination period and elongation growth period of the secondary trunk with the normal parts of the same period where no secondary trunks occurred. Differential genes involved in phytohormone signal transduction, phenylpropane biosynthesis, phenylalanine metabolism, glycolysis and other pathways can regulate not only the inhibition of early dormant buds but also the later development of the secondary trunk. Genes related to IAA synthesis are upregulated and indole-3-acetic acid content is increased, leading to the up-regulated expression of IAA intracellular vector genes. The IAA response gene (SAUR) receives and responds to IAA signals to promote the development of the secondary trunk. Through the enrichment of differential genes and functional annotations, a key regulatory pathway map for the occurrence of the secondary trunk of G. biloba was sorted out.
1 Introduction
Ginkgo biloba L. is the only remaining species of the Ginkgoaceae in China during the Quaternary glacial period with high adaptability, longevity and ornamental value (Seward, 1938; Cao, 2007). G. biloba is different from other gymnosperm species in that the phenomenon of ‘arising branches from the base of a stem’ (secondary trunk) is common from young trees to thousand-year-old trees. Del Tredici found in his survey of the West Tianmu Mountain Nature Reserve that 40% of the G. biloba in the area could produce sprouts, most of which were connected to a callus-like tumor (basal tree tumor) at the base of the stem. Therefore, it was believed that G. biloba could be regenerated by secondary trunk from the basal tree tumors, but the origin of the above-ground sprouts was not mentioned (Tredici, 1992a; Tredici, 1992b).
Xing Shiyan named the ‘branches arising from the base of a stem’ of G. biloba as the ‘secondary trunk’ for the first time and showed that the secondary trunk of G. biloba originated from the latent buds of the stem at the junction of root and stem. And the secondary trunk was essentially different from the root tillers of other tree species. The root system of return-young G. biloba does not produce rootstocks like the paulownia and other species. The secondary trunks of G. biloba usually grow upright around the main stem, with thick stems, small angles to the main stem, large, thick, multi-lobed leaves, obvious ‘return-young’ characteristics, growing faster than the main stem (Xing, 1996; Xing, 2013). The mechanism of the secondary trunk is related to the type of stem (branch) differentiation system, and the secondary trunk of G. biloba was part of the normal development of individuals in the natural state. With the increase of age, the number of secondary trunks increases greatly after the senescence of the tree top or destruction of the top buds of the secondary trunk, and the secondary trunk can be regenerated on the secondary trunk. As the number of secondary trunks increases, the base of the secondary trunk can form root discs. The secondary trunk retains the characteristics of the main stem and has a low basal rooting rate (Xing, 1996). The current research on the secondary trunk of G. biloba has mainly focused on the growth characteristics, distribution, regeneration, and utilization of the secondary trunk, but little has been reported on the origin of the secondary trunk of G. biloba at the anatomical level and the intrinsic molecular mechanism affecting the development of the secondary trunk.
In this study, the paraffin sectioning method was used to observe the initiation of the secondary trunk of G. biloba. By high-performance liquid chromatography, the endogenous hormones were measured in two different developmental stages of the secondary trunk of G. biloba and lateral branches to understand the influence of endogenous hormones on the development of the secondary trunk. At the same time, transcriptome sequencing and bioinformatics analysis were used to screen the differentially expressed genes affecting the formation and development of the secondary trunk and to speculate possible transcriptional regulation relationships. Through the research on the development of the secondary trunk, the occurrence mechanism of the secondary trunk can be understood and it can lay a theoretical foundation for revealing the mystery of the longevity of ancient ginkgo trees.
2 Materials and methods
2.1 Experimental material
The experimental materials for anatomical observation and endogenous hormone determination of the secondary trunk of G. biloba were collected from 4 years of seedling G. biloba and grafted G. biloba in the Forestry Experimental Station (N36°10’, E117°10’) of the South Campus of Shandong Agricultural University. The seedlings grow robustly and free from pests and diseases. The region is located in the southeast of Tai’an City, Shandong Province. It belongs to a warm temperate zone and semi-humid continental monsoon climate.
The experimental materials for the transcriptome sequencing of the secondary trunks were collected from the 4-year-old G. biloba seedlings from the 75# family of Shandong Forestry Germplasm Resource Center. The seedlings grew robustly and free from pests and diseases (Figure 1). The region is located in Zhangqiu District, Jinan City, Shandong Province. It belongs to a warm temperate zone and semi-humid continental monsoon climate.
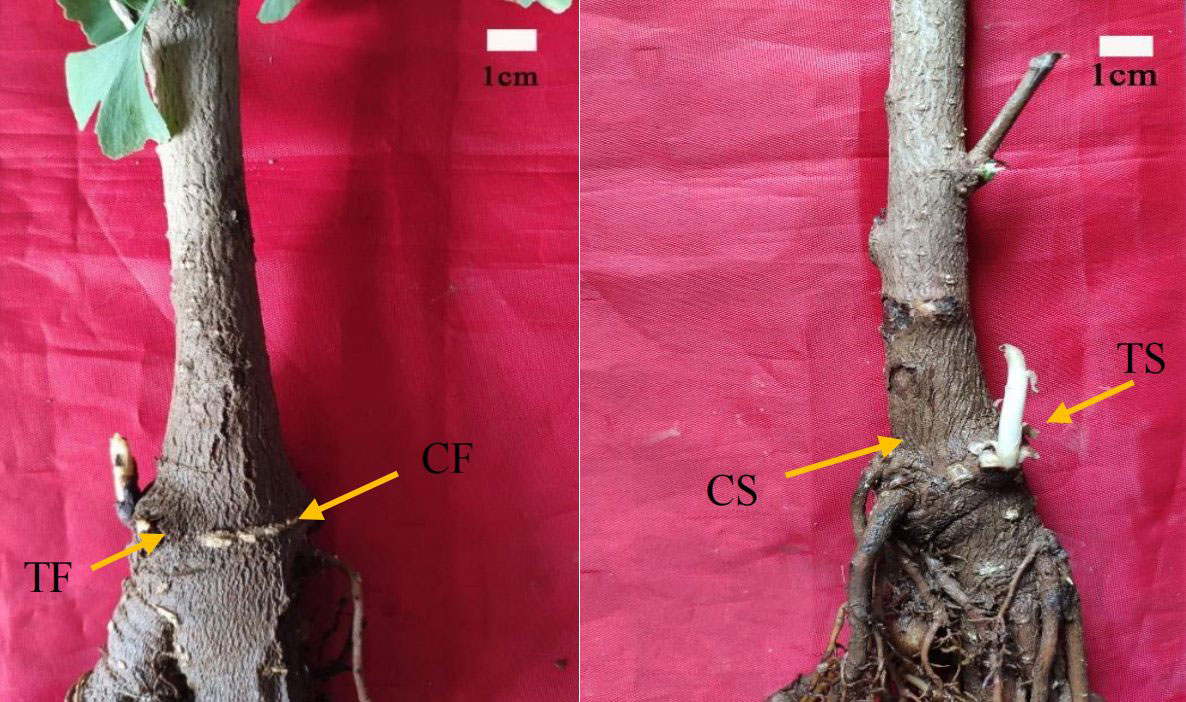
Figure 1 Experimental samples of transcriptome. TF, Secondary trunk at stage of germination; TS, Secondary trunk at stage of elongation; CF、CS, Normal tissue around secondary trunk.
2.2 Experimental method
2.2.1 Anatomical observation on the origin of the secondary trunk of G. biloba
For the 4-year-old seedling G. biloba, to observe the morphology of dormant buds of the secondary trunk and the development process of the secondary trunk after breaking the dormancy, the following experiment method was used: the G. biloba seedlings with consistent growth were divided into 8 groups of 3 replicates each, with 10 seedlings in each replicate. At the end of February, the first sampling was conducted and 5 seedlings were collected from each replicate from the first group to observe the dormant bud status by paraffin section. In early March, the remaining 7 groups of G. biloba were treated with stumping before the leaves were unfolded to break the dormant state of buds and promote the occurrence of the secondary trunk of G. biloba After cutting, a set of samples were taken at weekly intervals and brought back to the laboratory to make paraffin sections, and stained with safranin and green counterstaining. The paraffin sections were observed and photographed under a Nikon E200 microscope.
2.2.2 Endogenous hormone determination
The secondary trunks and the lowermost lateral branches of four-year-old grafted G. biloba were collected at two different developmental stages (germination and elongation), and three replicates of secondary trunks and lateral branches from each developmental stage were set up for the endogenous hormone determination by high-performance liquid chromatography. The specific method referred to Zhang Ning’s extraction and determination method (Zhang, 2019). The hormones determined: zeatin (ZT), auxin (IAA), abscisic acid (ABA), and gibberellin (GA3).
2.2.3 Transcriptome sequencing and analysis
The 4-year-old G. biloba seedlings from the no.75 family of Shandong Forest Germplasm Resource Center were selected. In April 2018, the secondary trunks of the four-year-old G. biloba seedlings of the same family at two stages of development (germination stage and elongation growth stage) were collected, and the normal tissues near the secondary trunk were collected as controls. Three replicates were set up for each period and control samples (Table 1). According to the RNA extraction method of Liu Xiaojing (Liu et al, 2018), the total RNA of 12 G. biloba samples was extracted to ensure the use of qualified samples for transcriptome sequencing.
After the samples were qualified, 3ug of RNA was taken from each sample as the starting material for library construction. The qualified total RNA samples were enriched into mRNA. The obtained mRNA was broken into short fragments by adding a fragmentation buffer. The fragmented mRNA was then used as a template to synthesize the first strand of cDNA with a six-base random primer. The buffer, DNA polymerase I, RNase H and dNTPs were added to synthesize the second strand of cDNA. The double-stranded cDNA was purified by QiaQuick PCR kit and eluted with EB buffer. The eluted and purified double-stranded cDNA was followed by terminal repair, base A addition and sequencing joint. Finally, different size fragments were selected for PCR amplification to complete the preparation of the library. After the library was constructed, the preliminary quantification was done with Qubit3.0 and the library was diluted to 1ng/ul. Agilent 2100 was used to detect the insert size of the library, and the qRT-PCR method was used to accurately quantify the effective concentration of the library. After the library inspection was qualified, sequencing was carried out by illumine platform.
Raw Data was filtered by removing low-quality sequences and joint pollution to obtain high-quality Clean Data. Mapped Data was get by comparing Clean Data with G. biloba reference genome (http://gigadb.org/dataset/100613). DESeq2 was used to analyze differentially expressed genes between the treatment and control groups. Genes with q<0.05 and |log2 Fold change|≥1 were selected as significantly differentially expressed genes, and each comparison group was screened to obtain the number of up-regulated and down-regulated genes. All the different Genes were analyzed by GO (Gene Ontology) and KEGG (Kyoto Encyclopedia of Genes and Genomes). The Sequence data are available in the NCBI Sequence Read Archive (SRA): SRR23730290, SRR23730291, SRR23730292, SRR23730293, SRR23730294, SRR23730288, SRR23730289, SRR23730295, SRR23730296, SRR23730297, SRR23730298, SRR23730299.
The RNA of secondary trunks at two different development stages and normal tissue without the secondary trunk of the same plant at the same location were extracted for reverse transcription, and the expression of differentially expressed genes in the transcriptome was verified. Fifteen randomly selected genes related to the development of the secondary trunk of G. biloba were quantified by real-time fluorescence.
3 Result
3.1 Anatomic characteristics of the origin of the secondary trunk
Anatomical studies on the secondary trunk of G. biloba at different developmental periods of showed that the development of the secondary trunk can be divided into four periods:
Dormancy period of the buds of the secondary trunk: In the natural state, that was, before the cutting treatment, a longitudinal cut at the base of the G. biloba stem showed a group of active parenchyma cells at the cortex, which had a small volume, large nucleus, deep staining, dense arrangement, and relatively vigorous division ability, and formed a densely distributed region. This part of the cells was the germinal cells of the buds of the secondary trunk, namely the dormant buds of the secondary trunk (Figure 2A). This part of cells differentiated into a primordial cell group composed of 1-2 layers of cells at the top through vertical and horizontal divisions, and a central blast cell area derived from the primordial cell group was below. The rib-shaped meristem area was under the central blast area. The meristem region was a cluster of closely arranged cells in a sub-circular or oval shape, the cells of the cluster were significantly smaller than surrounding parenchyma cells, and the cells were not uniform in size. One side of the cells were smaller, closely arranged, with a large nucleus, dense cytoplasm and deep staining, and the other side of the cells were larger and sub-circular. The cell cluster showed a strong capacity to divide (Figure 2B).
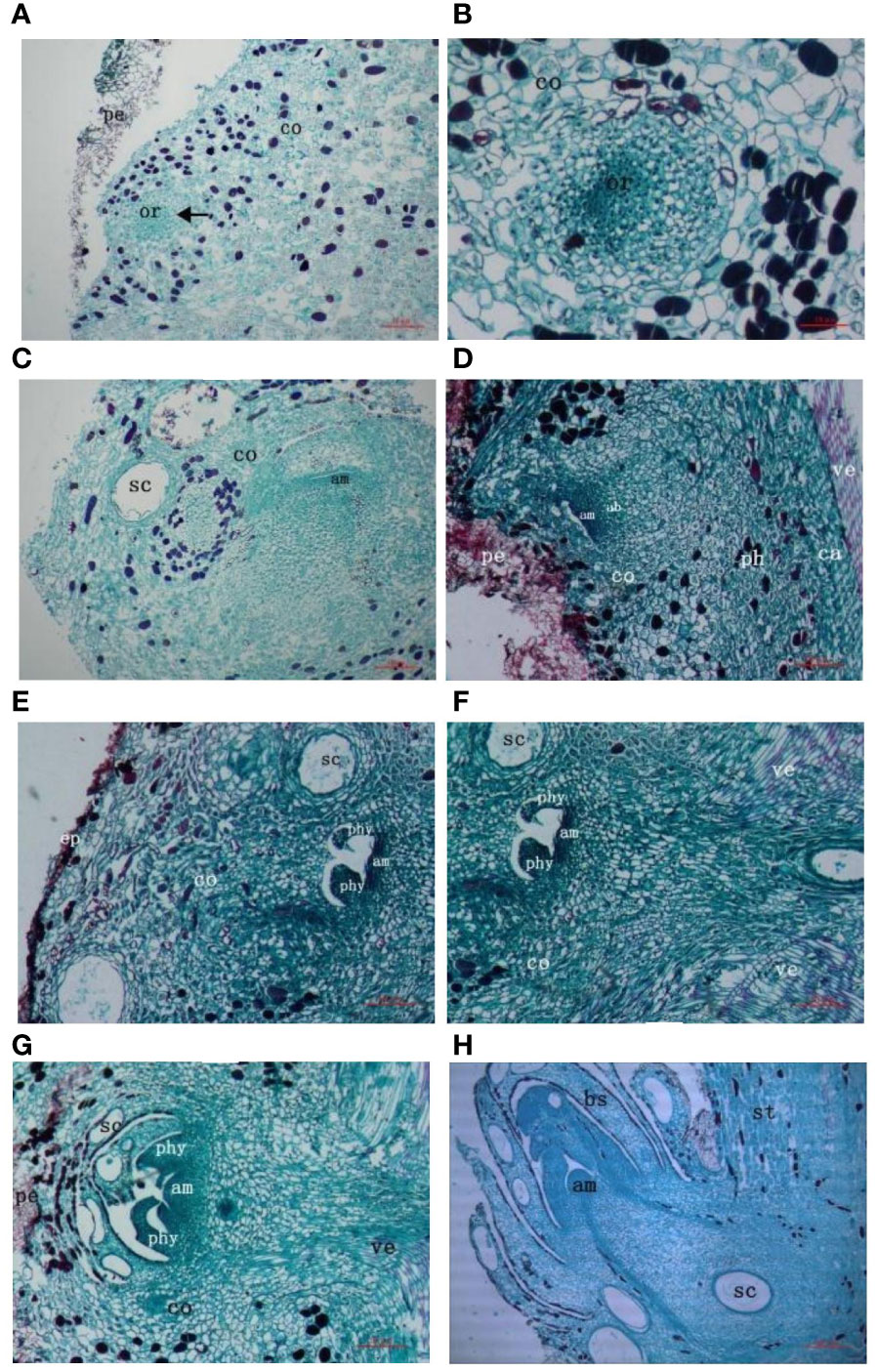
Figure 2 Anatomy of the origin of G. biloba secondary trunk. (A) The originating cells of secondary trunk form at the cortex. Arrows indicate originating cells. (B) The originating cells of secondary trunk differentiate into cell mass. (C, D) The cell mass further divides and differentiates to form meristematic regions with cytological division of shoot end. (E, F) The meristem region develops into leaf primordium and adventitious bud begins to connect with the vascular system of stem. (G) The adventitious buds continue to develop and produce new leaf primordial. The adventitious buds are connected to the vascular system of the stem. (H) The buds of secondary trunk form and break bark to grow. Ruler is 10μm. pe, periderm; or, originating cells of secondary trunk, co, cortex; sc, secretory cavity; am, apical meristem; ab, adventitious bud; ph, phloem; ca, cambium; ve, vessel; ep, epidermis; phy, phyllopodium; st, stem; bs, bud scale.
Differentiation period: Series of sections after cutting treatment showed that the dormancy stage of dormant buds was broken and the cell mass began to differentiate further, forming a clear gap above the primitive cell mass, which gradually enlarged. The apical cells in the meristematic region were closely arranged, with dense cytoplasm, deep staining, and vigorous division, forming a slight protrusion in the middle, which was the apical meristem. At the same time, the peripheral meristem areas on both sides of the apical meristem also showed strong splitting ability, but the differentiation of leaf primordium was not obvious (Figures 2C, D).
The formation period of the transport tissue: With the continuous development of the adventitious buds and the enlargement of the apical gap, the apical meristem formed more obvious protrusions. The surrounding meristem maintained a high frequency of cell division during the development of adventitious buds and formed protrusions after periclinal division, resulting in two leaf primordium. At this time, obvious vascular tissue could be seen at the bottom of adventitious buds, indicating that adventitious buds began to connect with the vascular bundle of the stem, forming a continuous vascular system to ensure that the trunk provided sufficient nutrients for the development of the buds of the secondary trunk (Figures 2E, F). The leaf primordium continued to grow to form young leaves and the secretory cavity began to exist in the young leaves. The surrounding meristem developed further to create a new leaf primordium on the inner side of the young leaf, at which point a continuous vascular system had been formed (Figure 2G).
Germination period: Adventitious buds continued to differentiate and produced new young leaves, with a certain number of secretory cavities in the young leaves, bud scales and buds. Since the tangential division speed of the rib-like meristem was greater than that of the periclinal division, the cells proliferated continuously in the longitudinal direction, so that the adventitious buds broke through the bark of the main trunk and developed into the secondary trunk under suitable light, temperature and moisture conditions (Figure 2H).
3.2 Hormone changes in the growth process of the secondary trunk and lateral branches
During the germination and elongation growth period, the content of each hormone in the secondary trunk was significantly higher than that in the lateral branches. During the germination period, the content of IAA in the secondary trunk (1.52μg·g-1) and the lateral branches (1.47μg·g-1) remained at a high level, and the content of IAA in the secondary trunk was significantly higher than that in the lateral branches (t <0.05) at the same development stage. In addition, the content of ZA and GA3 in the secondary trunk (0.98μg·g-1、1.26μg·g-1) was significantly different from that in the lateral branch (0.66μg·g-1、0.63μg·g-1) (t <0.01). And the content of the two hormones in the secondary trunk was also higher than that in the lateral branch.
The content of various hormones in the secondary trunk also showed significant differences at different developmental stages (t<0.05). At the elongation stage (1.64μg·g-1、1.58μg·g-1), the content of IAA and ZA in the secondary trunk increased significantly (t<0.05) compared with that at the germination stage (1.52μg·g-1、0.98μg·g-1), while the content of GA3 decreased significantly (t <0.01). The same expression trend was also observed in the lateral branches.
Among the common endogenous hormones in plants, ABA is a growing-inhibiting hormone, while IAA, ZA and GA3 are growing-promoting hormones. IAA and ZA synergistically affect the formation and development of buds, and ZA is the determinant of bud germination and growth. Therefore, IAA/ZA and (IAA+ZA+GA3)/ABA are the two more referential ratios. The results showed that the ratios of IAA+ZA+GA3/ABA were greater than 1 for both the secondary trunk and lateral branches at the germination stage, and the ratio of IAA+ZA+GA3/ABA in the secondary trunk was significantly greater than that of the lateral branch (t <0.01), and the ratio of IAA/ZA was significantly smaller than that of the lateral branch (t <0.01). During the elongation growth period, the ratios of IAA+ZA+GA3/ABA were greater than 1 for both the secondary trunk and lateral branches, and the ratio of IAA+ZA+GA3/ABA in the secondary trunk was greater than that of the lateral branches, while the ratio of IAA/ZA was smaller than that of the lateral branches. The ratio of (IAA+ZA+GA3)/ABA decreased during the elongation period compared to the germination period and the growth trend slowed down, while the ratio of IAA+ZA+GA3/ABA increased slightly in the lateral branches (Table 2; Figure 3).
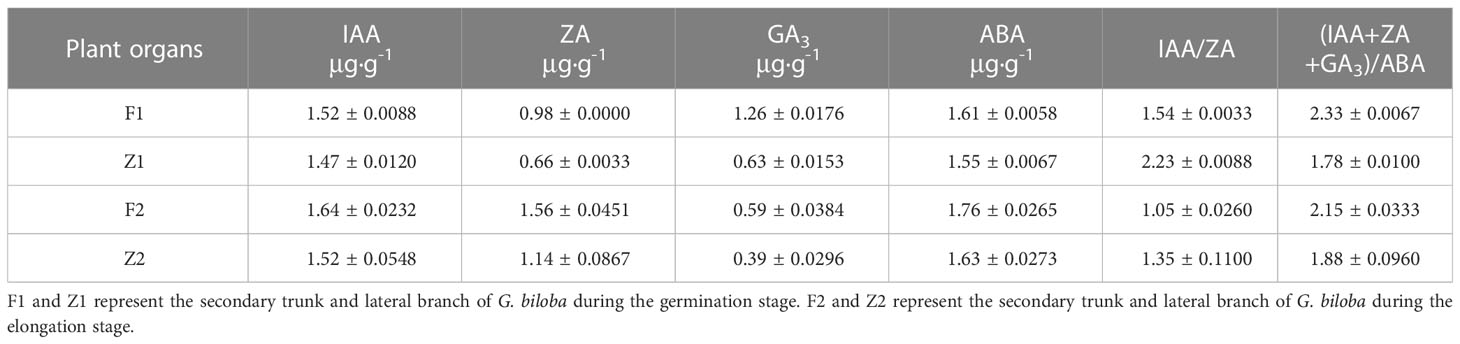
Table 2 Hormonal changes at different developmental stages in secondary trunk and lateral branch of G. biloba.
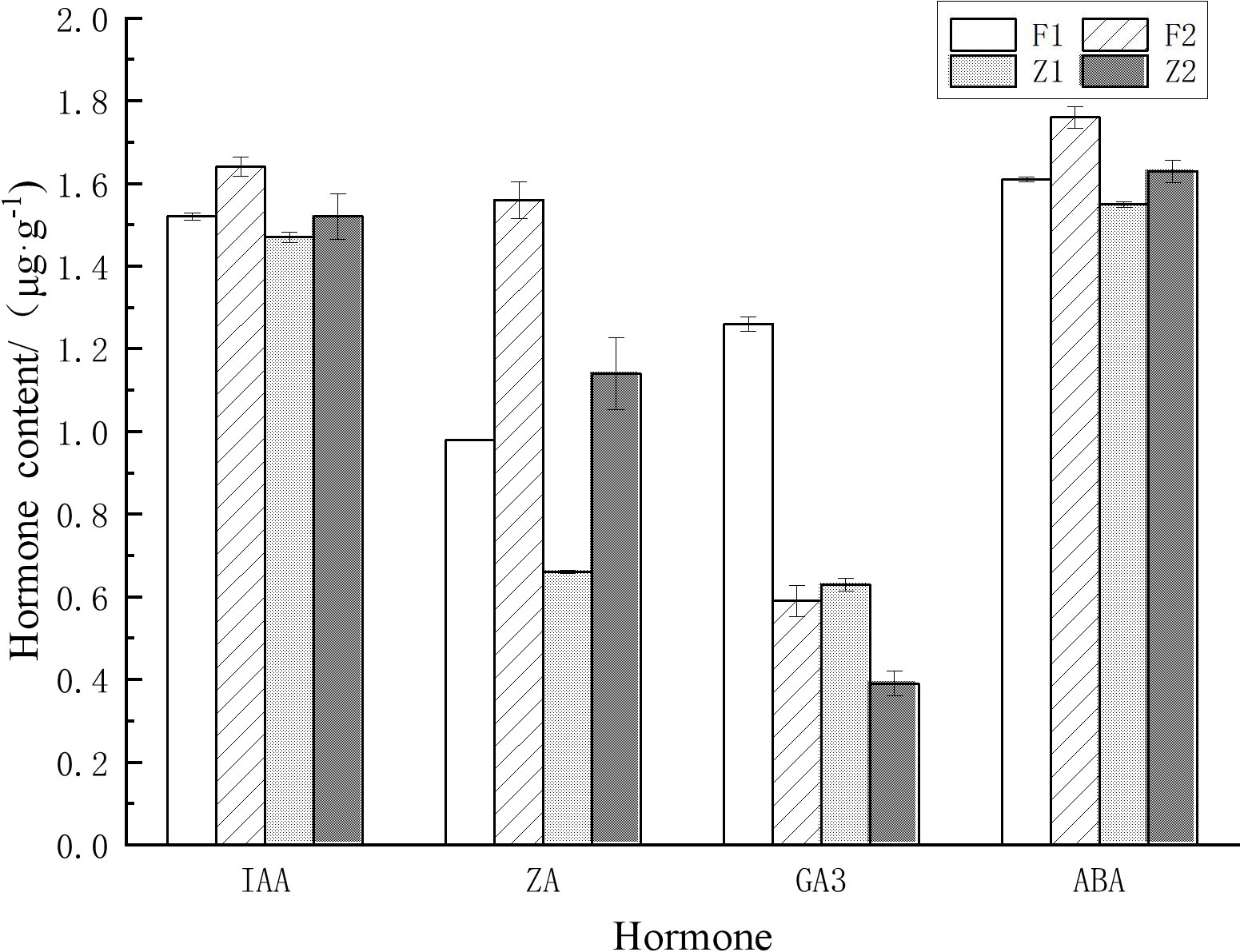
Figure 3 Hormonal changes at different developmental stages in the secondary trunk and the lateral branch of G. biloba.
3.3 Transcriptome sequencing
3.3.1 Quality evaluation of sequencing libraries and comparison results with reference genomes
In this study, transcriptome sequencing was performed on 12 G. biloba samples (including secondary trunk and control of two periods, three biological replicates respectively). The results showed that the Clean Reads Rate in each sample was above 96%, and after filtering, the Clean Q30 Bases Rate was all above 92.99%. The percentage of gene sequences of each sample aligned to reference genome sequence was above 88%. The results of gene sequence alignment showed that all samples met the sequencing requirements, providing quality assurance for subsequent bioinformatics analysis (Table 3; Figure S1). The clustering results of differentially expressed genes showed that the differentially expressed genes could be roughly divided into four categories, namely, the secondary trunk (TF) and control (CF, the part without the secondary trunk) at the germination stage as well as the secondary trunk (TS) and control (CS, the part without the secondary trunk) at the elongation growth stage. All repetition groups were clustered into the same category, with good repeatability within the group (Figure S2A).
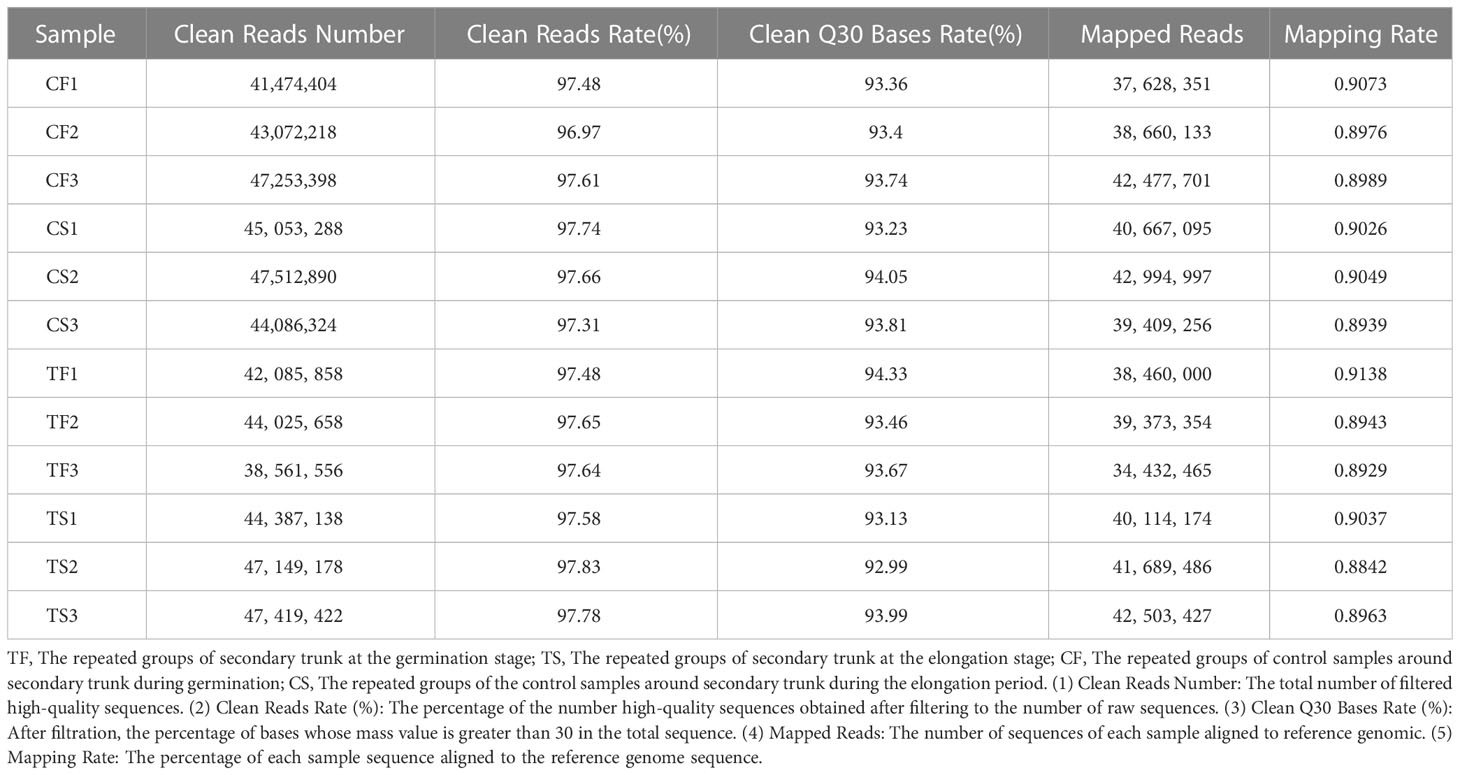
Table 3 The quality of sequencing library and comparison of cDNA library of sample and reference genome of G. biloba.
3.3.2 Differential expression analysis
The statistical results of differentially expressed genes showed that a total of 74 significantly different genes were screened out in the TF vs. CF comparison group (3 up-regulated and 71 down-regulated expressions). A total of 104 significantly different genes (76 up-regulated and 28 down-regulated expressions) were screened out in the TF vs. TS comparison group. A total of 2,151 significantly different genes (1368 up-regulated and 783 down-regulated expressions) were screened out in the TS vs. CS comparison group (Table 4; Figures S2B, C).
3.3.3 GO function analysis of differentially expressed genes
All DEGs were annotated in the KEGG database, NR database, Swissprot database, KOG database, GO database, Pfam database, etc. The functional enrichment analysis of the annotated DEGs in the GO database could reflect the cell state of the bud of the secondary trunk and the control group at different stages. GO analysis was performed on the differential genes produced at two stages of secondary trunk development compared with the control group. It was found that during the process of secondary trunk development, the differential genes in the two stages were involved in the metabolic process, cell process, single organism process, cell part, membrane part, catalytic activity and protein binding in biological processes. It was speculated that the above aspects were closely related to the germination and growth of the buds of the secondary trunk (Figures S3 –S5).
3.3.4 KEGG pathway analysis
All differentially expressed genes were enriched into 126 KEGG pathways. In all comparison groups, pathways enriched to the top 15 in the number of differential genes were shown in Table 5. 42 differentially expressed genes were enriched in plant hormone signal transduction pathways (32 up-regulated and 10 down-regulated genes) (Figure S6; Table 5).
3.3.5 The regulation of the germination and development of the secondary trunk of G. biloba
Based on the results of endogenous hormone determination and transcriptomic data analysis, the molecular regulatory pathways related to the germination and development of the secondary trunk of G. biloba were plotted. The results showed that the germination and development of secondary trunk were closely related to plant hormone signal transduction, phenylpropane biosynthesis, phenylalanine metabolism, glycolysis and other metabolic pathways, and there were complex mutual regulatory relationships, among which the synthesis and regulation of IAA and GA3 played an important role in the development of the secondary trunk (Figures 4A, B).
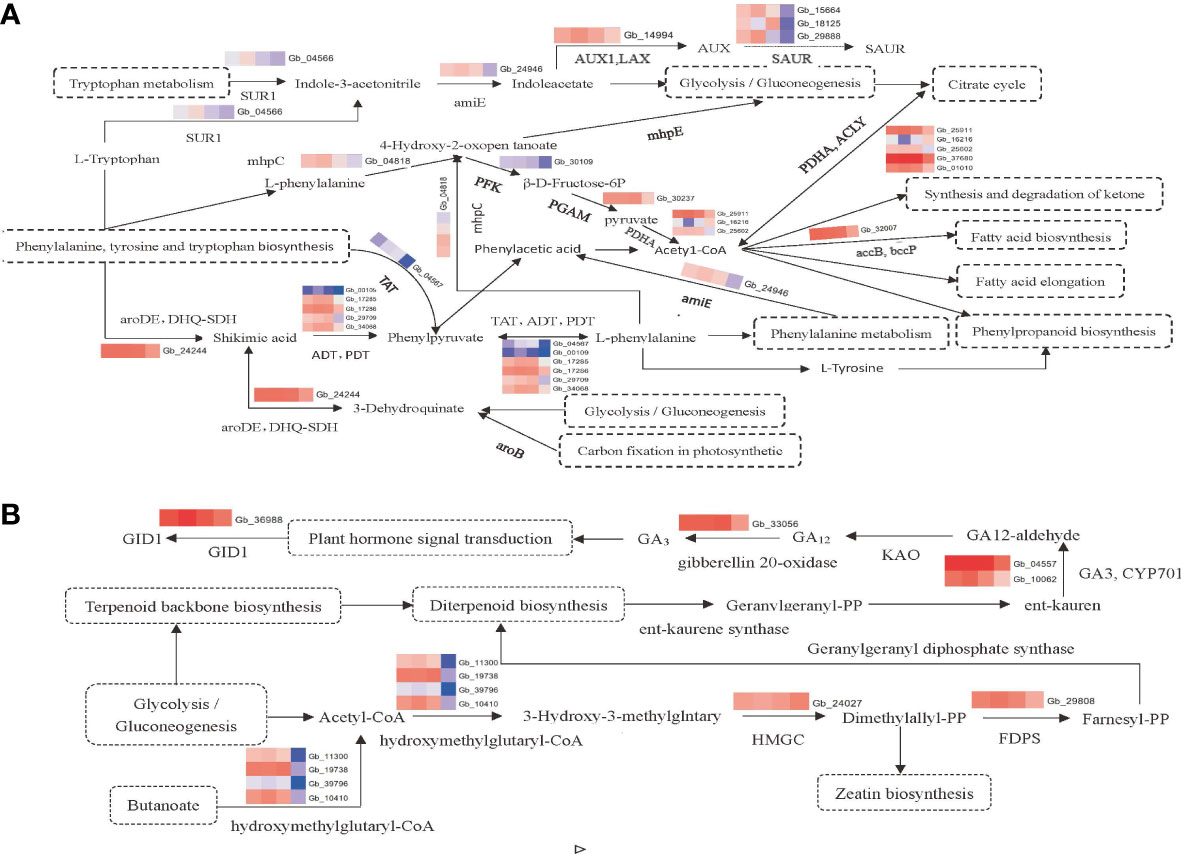
Figure 4 (A) Pathway map of differential gene expression related synthesis and regulation of IAA. (B) Pathway map of differential gene expression related synthesis and regulation of gibberellin.
3.3.6 Verification of differential gene expression
In this study, 15 differentially expressed genes were randomly selected for qPCR verification. The results showed that the expression trend of these 15 differentially expressed genes was generally consistent with the transcriptome sequencing data. Gb36988, Gb33056, Gb10062, Gb29808, Gb04557, Gb04566, Gb24946, Gb14994, Gb25911, Gb01010, Gb25602, Gb32007, Gb37680, and Gb24244 were up-regulated during the two development stages of the secondary trunk and down-regulated in the control group. Gb24027 was down-regulated in the two developmental stages of the secondary trunk and up-regulated in the control group. The results showed that the transcriptome sequencing data was accurate (Figure 5).
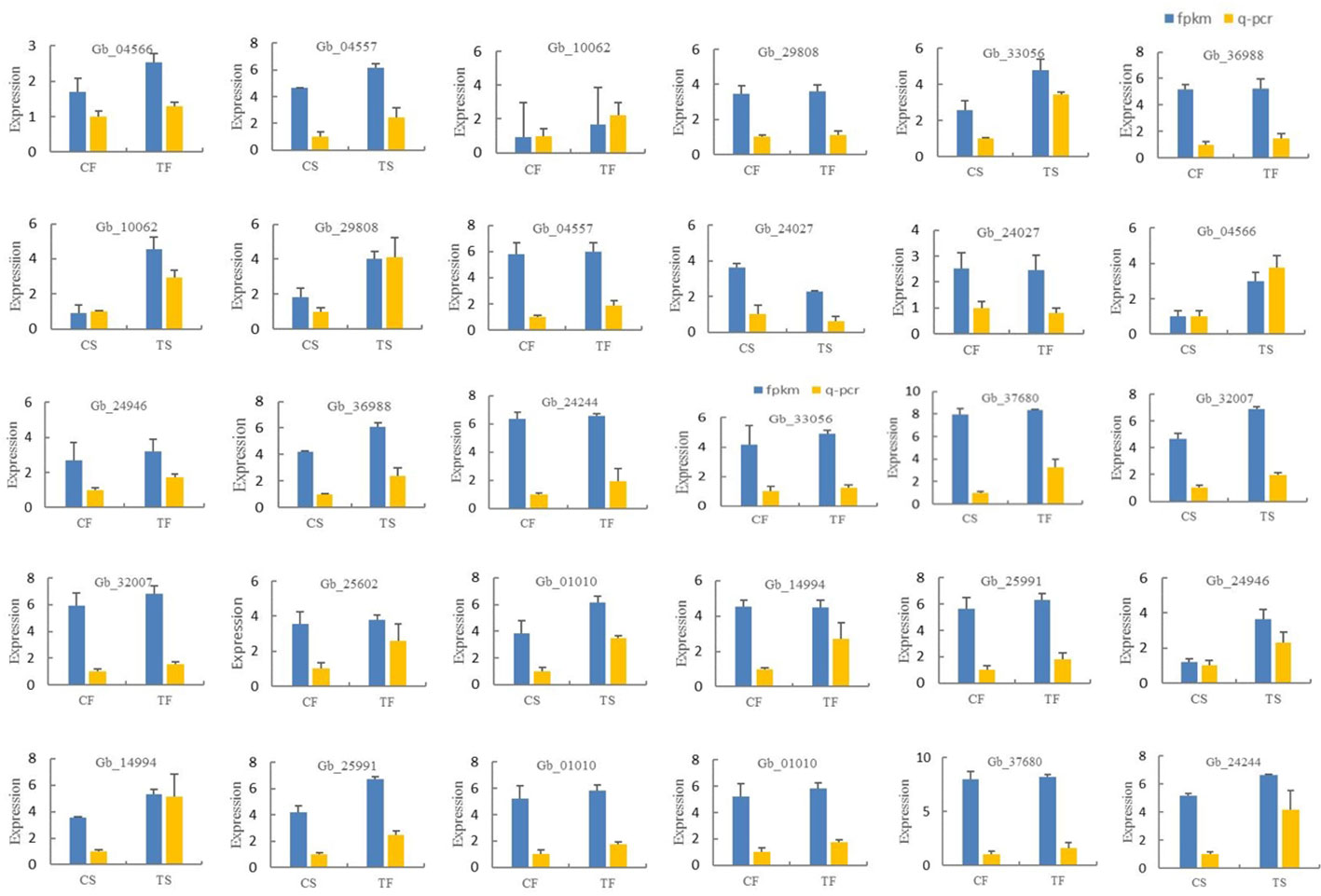
Figure 5 Expression verification of differentially expressed genes in different samples of G. biloba.
4 Discussion
The origin of the secondary trunk of G. biloba has been controversial (Fujii, 1985; Tredici, 1992b). Some studies suggested that the secondary trunk of G. biloba was produced by the chichi which was another special organ of G. biloba. Latent adventitious buds at the apex of rooted chichi could germinate into upgrowing branches under certain conditions and then developed into the secondary trunk (Xing, 1996; Fu, 2014). The chichi could produce roots and branches after it touched the ground (Fujii, 1985; Tredici, 1992a). It was also believed that the secondary trunk of G. biloba originated from the latent buds in the cortex of the stem at the junction of the root and stem. The secondary trunk was ‘shoots from the stem’ rather than shoots from the roots, part of the ontogenetic development of G. biloba, but no clear anatomical evidence has been found (Xing, 1996). This study confirmed the result and, in conjunction with previous studies, suggested that the origin of the secondary trunk should be divided into two categories. One type is produced by adventitious buds in the chichi, which can produce adventitious roots to provide nutrients for the secondary trunk, with a high germination rate. The other type is directly developed from the latent buds that exist in the cortex of the stem at the junction of the root and stem, which relies on the roots of the main stem to transport nutrients for development. The secondary trunk itself can not produce roots, retaining the characteristics of the main stem. The germination rate of the secondary trunk in the natural state is low and a large amount of germination does not occur until external stimulation. The secondary trunk of G. biloba may belong to autologous inhibitory dormancy, which allows plants to devote resources to controlling plant structure and reproductive growth, and allows regeneration when shoots are damaged (Horvath et al., 2003). The occurrence of the secondary trunk may be related to the age of G. biloba. With the increase of age, the secondary trunk will increase significantly after the senescence of the tops of the tree (Xing, 1996; Men et al., 2021). The secondary trunk is a reproductive strategy of G. biloba to sustain a life system when the tree reaches its growth limit or to resist bad environments and is the key to the preservation of G. biloba (Xiang et al., 2000; Lin and Zhang, 2004).
The secondary trunks are produced by breaking the dormancy of the dormant buds within the cortex at the junction of the root and stem of G. biloba, and are “branches from the stem”, but the secondary trunks are different from normal lateral branches in many aspects. The secondary trunk has obvious characteristics of “return to juvenile”, with an upright shape and a significantly smaller angle to the main stem than that of the lateral branches. Usually, the growth rate of the secondary trunk is significantly higher than that of the main stem, which can eliminate the position effect (Xing and Miao, 1996). This study found that the development of the secondary trunk was closely related to the regulation of endogenous hormones. At the germination stage, the content of each hormone in the secondary trunk was higher than that in the lateral branches, and the content of ZA and GA3 was significantly higher than that in the lateral branches. ZA could directly promote the growth of the lateral buds and played an important role in the germination stage of the secondary trunk (Lin and Zhang, 2004; Müller and Leyser, 2011).
GA3 might play a negative regulatory role in regulating the growth of lateral buds in Arabidopsis (Silverstone et al., 1997), rice (Qi et al., 2011) and hybrid aspen (Mauriat et al., 2011). However, for perennial G. biloba, the content of GA3 was significantly increased at the early developmental stage of the secondary trunk, and with the elongation growth, the content of IAA and ZA increased significantly and the GA3 content decreased significantly but was still higher than that of normal lateral branches. This is because the secondary trunk buds are in a dormancy state at the early stage of germination and the higher content of GA3 is conducive to breaking the dormancy and promoting germination. When the secondary trunk began to grow high, the GA3 gradually decreased and the content of each hormone was still higher than that of the lateral branches, indicating that the growth rate of the secondary trunk is higher than that of the lateral branches. ABA is an inhibitor of dormancy, and GA3 can counteract the inhibitory effect of ABA (Faust et al, 1997). GA3 can also promote the growth of lateral buds of papaya and mulberry (Ni, 2015). It indicates that GA3 is not unique to G. biloba in promoting the development of secondary trunk buds. Although GA3 has a negative regulatory effect on lateral buds in a few plants, GA3 promotes the growth of lateral buds for many woody plants (Ni, 2015).
The development of buds is usually closely related to the ratio of IAA to ZA in the primary tissue (Beveridge et al., 2003). At the germination stage, the (IAA+ZA+GA3)/ABA value of the secondary trunk and lateral branches were greater than 1, and the value of the secondary trunk was significantly greater than that of the lateral branches. It indicates that they are all growing and the growth trend of the secondary trunk is more obvious than that of lateral branches. The smaller IAA/ZA value is more favorable to the germination of the secondary trunk. In the elongation growth period, the (IAA+ZA+GA3)/ABA value of the secondary trunk decreased compared with the germination period and the growth trend slowed down, while the ratio of lateral branches increased slightly, which was due to the difference in the time between the secondary trunk and lateral branches entering the rapid growth period. The secondary trunk responds quickly to internal and external environmental signals, while the lateral branches respond relatively slowly.
Through transcriptome analysis, the GO enrichment analysis of the screened differentially expressed genes revealed that the differentially expressed genes related to the secondary trunk development were mainly enriched in the metabolic process, cell process, cell part, membrane part, catalytic activity and protein binding in the biological processes. It was generally consistent with the research results of Yang Lili about the release of natural dormancy of grape winter buds (Yang et al., 2020).
The secondary trunk is a unique organ of G. biloba. The dormancy of secondary trunk buds is controlled by the environment and genes. Combined with the endogenous hormone determination and sequencing analysis, it was found that endogenous hormones may be an important internal cause for the development of the secondary trunk. The development of the secondary trunk is closely related to several metabolic pathways and has complex interrelationships, especially with the synthesis and metabolism of hormones. The genes related to phenylalanine metabolism and phenylpropane biosynthesis were significantly enriched at the early developmental stage of adventitious bud in Arabidopsis, and phenylalanine significantly inhibited the development of adventitious bud in Arabidopsis (Wang et al., 2013).
However, these two pathways were also significantly enriched in this study. Several genes related to the biosynthetic pathways of phenylalanine, tyrosine and tryptophan were enriched in pathways related to the synthesis and regulation of IAA. For example, genes related to L-phenylalanine synthesis, such as Gb24244(aroDE, DHQ-SDH), Gb00109(ADT, PDT), Gb17285(ADT, PDT), Gb17286(ADT, PDT), Gb29709(ADT, PDT), Gb34068(ADT, PDT), Gb04567(TAT), were up-regulated in the secondary trunk group. The synthesis of shikimic acid could be promoted by DHQ-SDH, and then L-phenylalanine could be synthesized by tyrosine transaminase (TAT) and aromatic acid dehydrase (ADT, PDT), which inhibited the germination of the secondary trunk at the initial stage of germination. Phenylalanine was involved in phenylpropane biosynthesis and phenylalanine metabolism. In addition, the tryptophan metabolism-related genes Gb04566(SUR1)and Gb24946(amiE), and IAA signal response-related genes Gb14994(AUX1), Gb15664(SAUR), and Gb29888(SAUR) in plant hormone signal transduction pathway were also enriched, all up-regulated in the secondary trunk group. Tryptophan is the substrate of IAA synthesis. When G. biloba is stimulated by the certain internal and external environment, the up-regulated expression of Gb04566 (SUR1) promotes the accumulation of indole-3-acetonitrile, which increases the content of indole-3-acetic acid and upregulates the expression of IAA inflow vector gene in the tryptophan metabolic pathway. Finally, the IAA response gene SAUR receives and responds to the IAA signal to stimulate the development of the secondary trunk. In addition, the biosynthetic pathway of phenylalanine, tyrosine, and tryptophan can also produce L-tryptophan, which facilitates the accumulation of indole-3-acetonitrile and thus the synthesis of indole-3-acetic acid. At the same time, indole-3-acetic acid can indirectly promote the glycolysis pathway, which uses starch and sucrose metabolism as substrates to provide energy for the development of the secondary trunk.
The biosynthesis pathways of phenylalanine, tyrosine and tryptophan are important pathways linking tryptophan and phenylalanine, promoting the synthesis of acetyl-CoA through the intermediate products such as phenylpyruvate, phenylacetic acid, phenylalanine, and 4-hydroxy-2-oxy valerate. Acetyl-CoA is involved in phenylalanine metabolism, ketone body biosynthesis, phenylpropane biosynthesis, fatty acid synthesis and fatty acid prolongation. In addition, the carbon fixation pathway in glycolysis and photosynthetic organisms can also produce phenylalanine through the shikimic acid pathway (Sun, 2019).
GA3 can break the dormancy of buds and promote secondary trunk germination (Mäkilä et al., 2023), and the same results were obtained in the transcriptome. Genes related to gibberellin synthesis including Gb33056(gibberellin 20-oxidase), Gb04557(GA3, CYP701)and Gb10062(GA3, CYP701), and genes related to plant hormone signal transduction including Gb36988(GID1)and genes promoting diterpenoid biosynthesis including Gb11300, Gb19738, Gb39796, Gb10410, Gb24027(HMGCR) and Gb29808(FDPS) were enriched in the diterpenoid biosynthesis pathway. The terpene skeleton biosynthesis pathway is based on glycolysis to promote diterpene biosynthesis. Firstly, acetyl-CoA is synthesized, and the synthesis of isoamyl diphosphate is promoted by hydroxy methyl glutaryl-CoA synthase and hydroxy methyl glutaryl-CoA reductase (HMGCR). And then Farnesyl pyrophosphate synthase (FDPS) which is the key enzyme related to diterpenoids synthesis promotes the synthesis of farnesyl pyrophosphate and further promotes the diterpenoid biosynthetic pathway. In the diterpene biosynthesis pathway, the terpene skeleton biosynthesis is used as the substrate to synthesize geranylgeranyl pyrophosphate which is the substrate of gibberellin to further synthesized kaurene. Through the up-regulated expression of Gb04557 and Gb10062, kaurene oxidase (GA3, CYP701) promotes the synthesis of GA12 aldehyde and further synthesizes various gibberellins, including GA3. The accumulation of gibberellin can promote the phytohormone signal transduction pathway to up-regulate the expression of gibberellin receptor GID1 (Gb36988). And it can break the dormancy of secondary trunk buds in the cortex of the G. biloba stem and promote the germination and growth of the secondary trunk. In addition, isoamyl diphosphate, an intermediate product in the terpene skeleton biosynthetic pathway, can also promote zeatin biosynthesis and may play a role in the development of the secondary trunk.
The secondary trunk may be a reproductive strategy of G. biloba to sustain the life system when G. biloba reached its growth limit or to resist the adverse environment. It is a natural clonal multi-generation reproduction phenomenon. G. biloba can continuously produce new secondary trunks to make the mother tree that has reached its life limit rejuvenate and improve adaptability. Reproduction and renewal through secondary trunks is the key to the survival of G. biloba after catastrophes (Xiang et al., 2000; Fu et al., 2013).
5 Conclusions
The development process of secondary trunk of G. biloba can be divided into four stages, namely, dormancy stage, differentiation stage, conduction tissue formation stage, and germination stage. The contents of IAA, ZA, and GA3 in the budding stage of secondary trunk development were higher than those in the lateral branches, breaking dormancy of dormant buds and promoting secondary trunk germination. After the germination stage, the content of GA3 significantly decreased, while the content of IAA and ZA significantly increased, and the secondary trunk entered the elongation growth stage. In secondary trunk, the ratio of (IAA+ZA+GA3)/ABA is greater than that of lateral branches, while the ratio of IAA/ZA is extremely smaller than that of lateral branches, so the growth speed of secondary trunk is higher than that of lateral branches. Through transcriptome sequencing, the differentially expressed genes were screened out, and the key regulatory pathways for the occurrence and development of secondary trunk was sorted out. Phenylalanine metabolism and phenylpropane biosynthesis may inhibit the germination of early secondary trunk buds. After being stimulated by the certain internal and external environment, G. biloba synthesizes gibberellin using terpene skeleton biosynthesis as the substrate, which can promote the plant hormones signal transduction pathway and upregulate the expression of gibberellin receptor (GID1) to break the dormancy state of secondary trunk buds. At the same time, genes related to IAA synthesis are upregulated and indole-3-acetic acid content is increased, leading to the up-regulated expression of IAA intracellular vector genes. The IAA response gene (SAUR) receives and responds to IAA signals to promote the development of the secondary trunk.
Data availability statement
The data presented in the study are deposited in the NCBI Sequence Read Archive (SRA), accession numbers SRR23730290, SRR23730291, SRR23730292, SRR23730293, SRR23730294, SRR23730288, SRR23730289, SRR23730295, SRR23730296, SRR23730297, SRR23730298, SRR23730299.
Author contributions
L-MS designed the research; Z-YC and L-MS carried out the experiments; Z-YC and L-NS analyzed the data and wrote the manuscript; L-NS revised the manuscript. Conceptualization, L-MS. Writing—original draft preparation, Z-YC and L-NS. Writing—review and editing, L-MS. Data curation, X-YZ and X-JK. Experiments preformation, Z-YC, L-NS, QZ, and X-LH. Supervision, funding acquisition, and project administration, L-MS. All authors contributed to the article and approved the submitted version.
Funding
This study was supported by the Subject of Key R & D Plan of Shandong Province (Major Scientific and Technological Innovation Project) “Mining and Accurate Identification of Forest Tree Germplasm Resources (2021LZGC023)”. The Youth Fund of Natural Science Foundation of Shandong Province (ZR2020QC067). Introduction and training plan of young creative talents in universities of Shandong Province: Research group of forest tree biotechnology.
Acknowledgments
Thanks to Metware Biotechnology Co., Ltd. (Wuhan, China) for the testing services.
Conflict of interest
The authors declare that the research was conducted in the absence of any commercial or financial relationships that could be construed as a potential conflict of interest.
Publisher’s note
All claims expressed in this article are solely those of the authors and do not necessarily represent those of their affiliated organizations, or those of the publisher, the editors and the reviewers. Any product that may be evaluated in this article, or claim that may be made by its manufacturer, is not guaranteed or endorsed by the publisher.
Supplementary material
The Supplementary Material for this article can be found online at: https://www.frontiersin.org/articles/10.3389/fpls.2023.1161693/full#supplementary-material
Supplementary Figure 1 | Q30 quality control chart.
Supplementary Figure 2 | (A) Cluster Map of DEGs. (B) Statistics of differentially expressed genes in each comparison group of G. biloba. (C) Volcano map of DEGs in each comparison group of G. biloba. The abscissa is the expression multiple, and the ordinate is the significance degree of the change of expression quantity (P <0.05). Orange indicates genes that are significantly up-regulated in differential genes. Green indicates genes that are significantly down-regulated, and gray indicates genes that are not significantly different.
Supplementary Figure 3 | Distribution of q-values for enriched GO entries in each comparison group of G. biloba. Take the enriched GO entries in all samples for analysis, the ordinate is the GO entry and the abscissa is the name of different comparison groups. Different colors represent different degrees of enrichment.
Supplementary Figure 4 | Histogram of GO statistics of differentially expressed genes in each comparison group of G. biloba. The abscissa is each major category under GO, which represents various biological processes, cell components and molecular functions. The left ordinate is the proportion of this category, and the right ordinate is the specific number of genes in this category. Different colors represent different groups (differentially expressed genes are up-regulated and down-regulated).
Supplementary Figure 5 | Q-value enrichment chart of GO items in each comparison group of G. biloba. GO items enriched in all comparison groups were taken for analysis, and Q-value enrichment analysis was conducted for the three categories respectively. The ordinate is the secondary entry of GO and the abscissa is the degree of enrichment. Each point represents the degree of enrichment of the GO entry. The closer the color is to red, the higher the degree of enrichment. The size of each point indicates the number of genes enriched in the GO entry. The larger the point, the more genes are enriched in the GO entry, and vice versa.
Supplementary Figure 6 | Distribution of q-values of enrichment pathways in each comparison group of G. biloba.
References
Beveridge, C. A., Weller, J. L., Singer, S. R., Hofer, J. M. I. (2003). Axillary meristem development. budding relationships between networks controlling flowering, branching, and photoperiod responsiveness. Plant Physiol. 131, 927–934. doi: 10.1104/pp.102.017525
Faust, M., Erez, A., Rowland, L. J., Wang, S. Y., Norman, H. A. (1997). Bud dormancy in perennial fruit trees: physiological basis for dormancy induction, maintenance, and release. Hort Sci. 32, 623–629. doi: 10.21273/HORTSCI.32.4.623
Fu, Z. (2014). Study on the ontogeny of ginkgo biloba rooted chichi (Shandong Province (Taian: Shandong Agricultural University).
Fu, Z., Xing, S., Li, Z., Liu, L., Ren, J., Liu, Y. (2013). Growth characteristics of secondary trunk Ginkgo biloba in shengsheng garden in linyi city. J. Plant Genet. Resour. 14, 764–770. doi: 10.13430/j.cnki.jpgr.2013.04.031
Fujii, K. (1985). On the nature and origin of so-called "chichi"(nipple) of Ginkgo biloba l. Bot. Mag(Tokyo). 9, 444–450. doi: 10.15281/jplantres1887.9.440
Horvath, D. P., Anderson, J. V., Chao, W. S., Foley, M. E. (2003). Knowing when to grow: signals regulating bud dormancy. Trends Plant Sci. 8, 534–540. doi: 10.1016/j.tplants.2003.09.013
Lin, X., Zhang, D. (2004). The origin analysis of Ginkgo biloba population in tianmu mountain. Forestry Sci. 2, 28–31. doi: 10.11707/j.1001-7488.20040205
Liu, X., Sun, L., Wu, Q., Men, X., Yao, L., Xing, S., et al. (2018). Transcriptome profile analysis reveals the ontogenesis of rooted chichi in Ginkgo biloba l. Gene 669, 8–14. doi: 10.1016/j.gene.2018.05.066
Mäkilä, R., Wybouw, B., Smetana, O., Vainio, L., Solé-Gil, A., Lyu, M, et al. (2023). Gibberellins promote polar auxin transport to regulate stem cell fate decisions in cambium. Nat. Plants, 1–14. doi: 10.1038/s41477-023-01360-w
Mauriat, M., Sandberg, L. G., Moritz, T. (2011). Proper gibberellin localization in vascular tissue is required to control auxin-dependent leaf development and bud outgrowth in hybrid aspen. Plant J. 67, 805–816. doi: 10.1111/j.1365-313X.2011.04635.x
Men, X., Sun, L., Li, Y., Li, W., Xing, S. (2021). Multi-omics analysis reveals the ontogenesis of basal chichi in Ginkgo biloba l. Genomics 113, 2317–2326. doi: 10.1016/j.ygeno.2021.05.027
Müller, D., Leyser, O. (2011). Auxin, cytokinin and the control of shoot branching. Ann. Bot. 107, 1203–1212. doi: 10.1093/aob/mcr069
Ni, J. (2015). Regulation of gibberellin on growth of lateral buds of jatropha curcas (Anhui Province (Hefei: China Science and Technology University).
Qi, W., Sun, F., Wang, Q., Chen, M., Huang, Y., Feng, Y., et al. (2011). Rice ethylene-response AP2/ERF factor OsEATB restricts internode elongation by down-regulating a gibberellin biosynthetic gene. Plant Physiol. 157, 216–228. doi: 10.1104/pp.111.179945
Seward, A. C. (1938). The story of the maidenhair tree. Sci. Prog. 32, 420–440. doi: 10.2307/43412201
Silverstone, A. L., Mak, P. Y. A., Martinez, E. C., Sun, T. (1997). The new RGA locus encodes a negative regulator of gibberellin response in Arabidopsis thaliana. Genetics 146, 1087–1099. doi: 10.1093/genetics/146.3.1087
Sun, L. (2019). Regulation mechanism analysis of the transcription, protein and metabolic of the flavonoids synthesis of the leaves of ginkgo biloba (Shandong Province (Taian: Shandong Agricultural University).
Tredici, P. D. (1992a). Natural regeneration of Ginkgo biloba from downward growing cotyledonary buds(basal chichi). Am. J. Bot. 79, 522–530. doi: 10.1002/j.1537-2197.1992.tb14588.x
Wang, X., Yang, Z., Zhang, S., Li, H., Li, S. (2013). Digital gene expression profile analysis of early adventitious buds in Arabidopsi. Chin. J. Bioengineering 29, 189–202. doi: 10.13345/j.cjb.2013.02.012
Xiang, Y., Xiang, B., Zhao, M., Wang, Z. (2000). Investigation report on natural forest and Ginkgo biloba populations in West tianmu mountain in zhejiang province. Guizhou Sci., 77–92. doi: CNKI:SUN:GZKX.0.2000-Z1-011
Xing, S., Miao, Q. (1996). Study on the biological characteristics of secondary trunk. Forestry Sci. Technol. Newsletter. 2, 6–9.
Yang, L., Chen, Z., Zhao, Y., Niu, Z., Niu, S., Shi, X., et al. (2020). The regulation of plant signal transduction on the release of dormancy of 'Xiahei' grape winter buds. Mol. Plant Breed. 18, 1438–1446. doi: 10.13271/j.mpb.018.001438
Keywords: Ginkgo biloba, secondary trunk, anatomy, endogenous hormones, transcriptome sequencing
Citation: Cao Z-y, Su L-n, Zhang Q, Zhang X-y, Kang X-j, Li X-h and Sun L-m (2023) The development and transcriptome regulation of the secondary trunk of Ginkgo biloba L.. Front. Plant Sci. 14:1161693. doi: 10.3389/fpls.2023.1161693
Received: 08 February 2023; Accepted: 25 April 2023;
Published: 30 May 2023.
Edited by:
Kai-Hua Jia, Shandong Academy of Agricultural Sciences, ChinaReviewed by:
Yaqiong Wu, Chinese Academy of Sciences, ChinaZihan Zhang, Chinese Academy of Forestry, China
Copyright © 2023 Cao, Su, Zhang, Zhang, Kang, Li and Sun. This is an open-access article distributed under the terms of the Creative Commons Attribution License (CC BY). The use, distribution or reproduction in other forums is permitted, provided the original author(s) and the copyright owner(s) are credited and that the original publication in this journal is cited, in accordance with accepted academic practice. No use, distribution or reproduction is permitted which does not comply with these terms.
*Correspondence: Li-min Sun, c3VubGltaW4wNkAxNjMuY29t
†These authors have contributed equally to this work