- 1Department of Plant Science, School of Agriculture and Biology, Shanghai Jiao Tong University, Shanghai, China
- 2Grape and Wine Institute, Guangxi Academy of Agricultural Sciences, Nanning, Guangxi, China
- 3College of Chemistry and Chemical Engineering, Zhongkai University of Agricultural Engineering, Guangzhou, China
Volatile compounds including terpenes, aldehyde, phenol, and alcohol are significantly contributed floral and fruity aromas to the Muscat variety. ‘Ruidu Hongyu’ grapevine is one of the newly developed grape varieties, and cultivation of this variety has been extended across China due to unique quality traits and taste. In this study, HS-SPME/GC−MS and transcriptome sequencing analysis were performed to evaluate the impact of exogenous 2,4-epibrassinolide (EBR), jasmonic acid (JA), and their signaling inhibitors brassinazole (Brz)/sodium diethyldithiocarbamate (DIECA) on the biosynthesis of aroma substances in ‘Ruidu Hongyu’ grapevine. According to the results, exogenous BR and JA promoted the accumulation of various aroma substances, including hexenal, 2-hexenal, nerol oxide, vanillin, hotrienol, terpineol, neral, nerol, geraniol, and geranic acid. After EBR and JA treatments, most of the genes responsible for terpene, aldehyde, and alcohol biosynthesis expressed at a higher level than the CK group. Relatively, EBR treatment could not only promote endogenous BR biosynthesis and metabolism but also elevate BR signaling transduction. JA treatment contributed to endogenous JA and MeJA accumulation, as well. Through transcriptome sequencing, a total of 3043, 903, 1470, and 607 DEGs were identified in JA vs. JD, JA vs. CK, BR vs. CK, and BR vs. Brz, respectively. There were more DEGs under both EBR and JA treatments at late fruit ripening stages. The findings of this study increase our understanding regarding aroma substances biosynthesis and endogenous BR/JA metabolism in response to exogenous EBR and JA signals.
Introduction
Aroma is one of the most eminent indices to gauge grape wine quality (Pinu et al., 2019). Though the overall composition of most wine grape varieties is quite similar, the differences in aroma composition and flavor between them are quite remarkable (Ruiz et al., 2019). The aroma of high-quality wines is usually rich, balanced, and typically outstanding (Rinaldi et al., 2020). There are eight categories represented volatile compounds in grape berries, namely monoterpenes, norisoprenoids, aliphatics, higher alcohols, esters, phenylpropanoids, and methoxypyrazines (Wu et al., 2019) and they mainly contribute to the fragrance of flowers, citrus, tropical fruit, violet, and green pepper, respectively, which together constituted the complex and elegant aroma of high-quality wine (Richmond et al., 2019). Terpenes are one of the most important components of aroma substances, which exist as free and combined forms in wine grapes. Because of its low threshold and great contribution to the grape aroma, it has been widely studied (Jin et al., 2022). The majority of terpenes found in wine grapes are monoterpenes, including linalool, geraniol, citronellol, nerol, α-terpinol, menthol and citral (Chigo-Hernandez et al., 2022), which have a significant impact on the aroma profile of the wine (Yue et al., 2022). Compared with monoterpenoids, sesquiterpenes are present at lower levels in grape berries and wine but they play a vital role in wine aroma (Luo et al., 2019). Moreover, alcohols, esters, and aldehydes all play a significant role in the aroma composition of mature grape berries. Furthermore, different compounds have different sensory thresholds and their interaction impart the characteristic flavor to grapevines (Wu et al., 2020).
Till now, the pathways of terpenes biosynthesis and metabolism have been systematically paraphrased (Englund et al., 2018). Plant terpenes are mainly synthesized independently by two pathways (Qiao et al., 2021). The mevalonic acid (MVA) pathway located in the cytoplasm, and the 1-deoxy-D-xylulose 5-phosphate (DXP)/methylerythritol phosphate (MEP) pathways located in the plastid (Zhang et al., 2021). In MVA pathway, there are seven rate-limiting enzymes, including acetyl-CoA C-acetyltransferase (AACT), 3-hydroxy-3-methylglutaryl-CoA synthetase (HMGS), 3-hydroxy-3-methylglutaryl coenzyme-A reductase (HMGR), phosphomevalonate kinase (PMK), geranyl diphosphate synthase (GPPS), farnesyl diphosphate synthase (FPPS) and terpene synthases (TPS) (Mukherjee et al., 2022). Moreover, A total of seven key rate-liming enzymes are responsible for participating in the MEP pathway, namely Deoxy-D-xylulose 5-phosphate synthase (DXS), DXP reducto-isomerase (DXR), 2-C-methyl-D-erythritol 4-phosphate cytidylyltransferase (MCT), 4-hydroxy-3-methylbut-2-en-1-yl diphosphate synthase (HDS), geranyl geranyl pyrophosphate synthase (GGPPS), GPPS and TPS (Wan et al., 2021). After a series of enzymatic reactions, sesquiterpene and geranyl linalool are finally biosynthesized in the cytoplasm, while diterpene and monoterpene are biosynthesized in the plastid (Lv et al., 2022b).
The accumulation of aroma substances could be regulated by many factors, including the environment, the cultivation pattern, and exogenous plant growth regulators (Kou et al., 2021; Yang et al., 2022). Recently, brassinolide (BR), was recognized as the sixth-largest phytohormone due to its promoting effects on the formation of multiple biochemical properties in grapevine (Vitis vinifera L.) (Li et al., 2023). In terms of terpenes contents, 100 μmol L-1 BR was reported to increase β-pinene and D-limonene in ‘Kyoho’ grape berries. Furthermore, 3-hydroxy-3-methyl glutaryl coenzyme A reductase (HMGR) activity was increased by the induction of exogenous BR (Zheng et al., 2021). It is noteworthy that MeJA also had a great impact on the increment of aroma compounds such as monoterpenes in ‘Muscat Hamburg’ grape pericarp, especially linalool, α-terpineol, and oxides (Yue et al., 2021). Furthermore, the application of MeJA was also responsible to raise the content of 1-hexanol, hexanal, and 2-heptanol in ‘Cabernet Sauvignon’ grapevine. Overall, BR and JA both played enormous roles in aroma substance accumulation in the grapevine. However, further research work needs to be conducted specifically for the understanding of the molecular mechanism.
‘Ruidu Hongyu’ grapevine, as a table grape cultivar in China, has various advantageous traits including high-yield, pink coloring and precocity (Xu et al., 2014). Previously, the effect of EBR and JA on berry quality was evaluated, and they were responsible for improving anthocyanin, fatty acid, and sugar levels (Li et al., 2022). However, the mechanism of exogenous BR and JA regulation on aroma volatilization of ‘Ruidu Hongyu’ grapevine has yet to be addressed. Therefore, a co-analysis of metabolite and transcriptome was conducted in this study. After that, we assessed how EBR, JA, and their signaling inhibitors (Brz and DIECA), influenced the aroma substances biosynthesis in the ‘Ruidu Hongyu’ grapevine. Moreover, a molecular study at the transcriptional level was implemented to understand the potential mechanism. Above findings aimed to suggest exogenous EBR or JA application was a suitable strategy to improve the flavor of table grapes, which could be carried out in future grapevine cultivation.
Materials and methods
Vineyard, EBR and JA treatments, and sampling set
Six-year-old ‘Ruidu Hongyu’ grapevine was cultivated in the Grape and Wine Institute, Guangxi Academy of Agricultural Sciences (22.61°N, 108.24°E, Nanning, Guangxi, China) in 2021. In this study, five treatments group such as the CK, 0.5 mg L-1 EBR (BR), 0.5 mg L-1 EBR + 1 mg L-1 Brz (BR signaling inhibitor; BB), 100 μmol L-1 JA, and 100 μmol L-1 JA + 10 mmol L-1 DIECA (JA signaling inhibitor; JD) were selected to evaluate the aroma and their mechanism in ‘Ruidu Hongyu’ grapevine. Applications were administered to the grape berries at DAA 30 (days after anthesis), and three sampling intervals—DAA 30 (0 days after treatment), DAA 50 (20 days after treatment), and DAA 70 (40 days after treatment) were defined. A total of 300 berries were collected from 20 vines of each treatment and CK group at every sampling stage. All berries were immediately transported with dry ice to the Department of Plant Science, School of Agriculture and Biology, Shanghai Jiao Tong University (121°29’ W, 31°11’ N, Shanghai, China). Furtherly, berry size and berry weight were determined by a vernier caliper (Mitutoyo, TKY, Japan) and an analytical balance (Sartorius, German), respectively. Then, grape berries were ground using a vacuum grinder (IKA, GER). Powder was frozen under liquid nitrogen and stored at −80°C for further analysis, including the determination of free-form aroma substances, RNA extraction and qRT-PCR analysis.
Chemicals
Chemicals used for the detection of VOCs including D-gluconolactone and 4-methyl-2-pentanol were purchased in Sigma-Aldrich (SHH, CHN), and PVPP, NaCl used for extraction of VOCs were purchased in Sangon Biotech (SHH, CHN). Moreover, TB Green® Fast qPCR Mix used for qRT-PCR was purchased in TaKaRa (Dalian, China).
Free-form volatiles detected by HS-SPME/GC−MS
The previously established method of our research group (Li et al., 2017; Javed et al., 2018; Javed et al., 2019) for the determination of free-form volatiles was applied in this study. Approximately 100 grape berries without seeds from each treatment group were crushed, and 1 g each of polyvinylpyrrolidone (PVPP) and D-gluconolactone were added. The powder was naturally thawed at a 4°C (refrigerator) for 4 h, followed by a 10 min centrifugation process at 4°C with 8,000 g.
Next, took 5 mL grape juice supernatant to a 15 mL vial containing 1 g of sodium chloride (NaCl) and then 10 μL of 4-methyl-2-pentanol (internal standard, concentration was 1.0018 g L-1) was mixed. The vial was equilibrated at 40°C for 30 min with agitation at 500 rpm. Before sample extraction, activated 2 cm DVB/CAR/PDMS 50/30 μm solid-phase micro-extraction (SPME) fiber (Supelco, Bellefonte, PA, USA) at 250°C. Following that, under the same agitation condition, the activated SPME fiber was inserted into the headspace at 40°C for 30 min. In the end, inserted it into the GC injector port for 8 min to desorb the volatiles.
The determination of volatile compounds in grape juice was carried out on an Agilent 7890 gas chromatography (GC) system (Santa Clara, CA, USA), with an autosampler system in conjunction with an Agilent 5975C mass spectrometer (Santa Clara, CA, USA). Helium was equipped in the system and supplied at a flow rate of 1 mL min-1. The specific procedure was as follows: after maintaining the temperature at 40°C for five minutes at a rate of 5°C per minute, it was raised to 240°C at a rate of 20°C per minute. Finally, the temperature was raised to 260°C and held there for 5 minutes. Three replicates were set in this study, and the final value of each free-form volatile was reported in mg L-1 and shown in the heat map. The information and retention time of terpenes detected in this study was shown in Table S2.
RNA extraction, illumina sequencing, and transcriptome data analysis
The RNA extraction of berry samples was carried out on an RNA prep Pure Plant Plus Kit (TaKaRa, Dalian, China). A BIO-RADXR gel imaging analysis system (Bio-Rad, CA, United States) was used to assess the quality and integrality of the extracted RNA. A total of 24 libraries were established using Illumina HiSeq X Ten (Illumina Inc., San Diego, CA, USA), and produced 150 bp paired-end reads. At least three biological replicates should be used in each treatment
The sequencing was performed at Shanghai APPLIED PROTEIN TECHNOLOGY (SHN, China). Trimming adaptor sequences were used to clean raw reads, and the clean reads were aligned in the Tophat v2.0.943 program, referring to a grapevine reference genome (https://www.ncbi.nlm.nih.gov/genome/?term=grape). The raw RNA sequencing data has been uploaded and deposited into the National Center for Biotechnology Information (NCBI) BioProject database, and the accession number was PRJNA943117.
In this research, the absolute value of the log2 (fold change) with fragments per kilobase million (FPKM) §1 was used as a threshold for the discrimination of the significant differentially expressed genes (DEGs) throughout the detection of DEGs. Based on the calculated FPKM values, DEGs between different samples were analyzed. The main screening steps were as follows: (1) DESeq software was used to standardize the number of gene counts in each sample, and different expression fold-changes were computed. (2) A negative binomial distribution test was carried out to determine the significance of different readouts. Two criteria were used to determine the differential expression of the same gene between the compared samples. One is based on the expression fold change, i.e. the fold change of the same gene expression level between two samples. The other is based on the FDR (false discovery rates) value, which is calculated by first calculating the p-value for each gene and then using the FDR error control method to correct the p-value for multiple hypothesis testing. The criteria for DEGs of the final screen were p < 0.05 and a fold change in expression greater than 2. GO (Gene Ontology) enrichment analysis was performed after obtaining DEGs. Gene ontology (GO) enrichment and KEGG pathway analysis were performed for the differentially expressed genes (DEGs), through an online website (http://cloud.aptbiotech.com/#/product-list).
qRT-PCR determination
To further verify the sequencing results obtained from RNA sequencing, A PrimeScript™ RT Reagent Kit in combination with gDNA Eraser (Perfect Real Time) (TaKaRa, Dalian, China) was used to extract one microgram of total RNA. After that, the first-strand cDNA was obtained. The total final volume was 10 μl in total, made up of 5 μl of TB Green® Fast qPCR Mix, 3 μl of ddH2O, 1 μl of a forward and reverse primer mixture, and 1 μl of cDNA. Then, qRT−PCR was carried out in a CFX-connected Real-Time PCR Detection System (Bio-Rad, CA, USA). The sequences of all genes and transcription factors identified in this study were acquired from the NCBI database (https://www.ncbi.nlm.nih.gov/). The primers were designed by the qPrimerDB-qPCR Primer Database (https://biodb.swu.edu.cn/qprimerdb/browse_plants). All primer sequence information was shown in Table S1. The following procedure was used to conduct the reactions: 95°C for 20 s, followed by 39 cycles of 95°C for 15 s, 55°C for 15 s, and 60°C for 15 s. The determination of relative gene expression was according to the 2-ΔΔCt method.
Statistical analysis
One-way ANOVA and Duncan’s multiple range test in SPSS version 19.0 (IBM Corp., Armonk, NY, USA), and the principal component analysis (PCA) were used for data analysis at the p ≤ 0.05 level. Heatmaps of metabolite concentrations as well as gene expressions were constructed by GraphPad Prism 9.0 (GraphPad Software, Inc., San Diego, CA, USA) and Visio 2020 (Microsoft, SEA, USA).
Results and discussion
Exogenous EBR and JA ameliorate physiological parameters of grape berries
Physiological parameters such as longitudinal diameter, transverse diameter, single berry weight, total soluble solid (TSS), and titratable acids (TA) were measured in CK, BR, JA, BB, and JD treatment groups throughout the grapevine development and ripening stages (Figure S1). Exogenous EBR and JA were found to be increased berry size and TSS while decreased TA contents (Figures S1A, B). Furthermore, lower TSS content was observed in the BB and JD applications at DAA 50 and DAA 70. TA levels were higher in both the BB and JD treatments throughout the study.
Exogenous EBR and JA regulated aroma compounds formation of grape berries
In this study, a total of 24 kinds of free-form compounds including 1 alcohol (1-Hexanol); 2 aldehydes (Hexenal, 2- Hexenal) and 19 terpenes were detected successfully. In all, BR and JA treatments have more VOCs (Volatile Organic Compounds) than the CK group. Furthermore, the concentration of free-form terpene, alcohol, and aldehyde increased under all treatments throughout the grapevine development and ripening. Especially at DAA 70, the increasing trend becomes noticeable (Figures 1A, B). Interestingly, the exogenous application of EBR at DAA 50 and DAA 70 and JA at DAA 70 increased the content of aldehydes (hexanal and 2-hexenal) and terpenes (hotrienol, terpineol, nerol, geraniol, linalool, and citral). Compared to the BR and JA groups, their contents dropped in both BB and JD treatments, respectively.
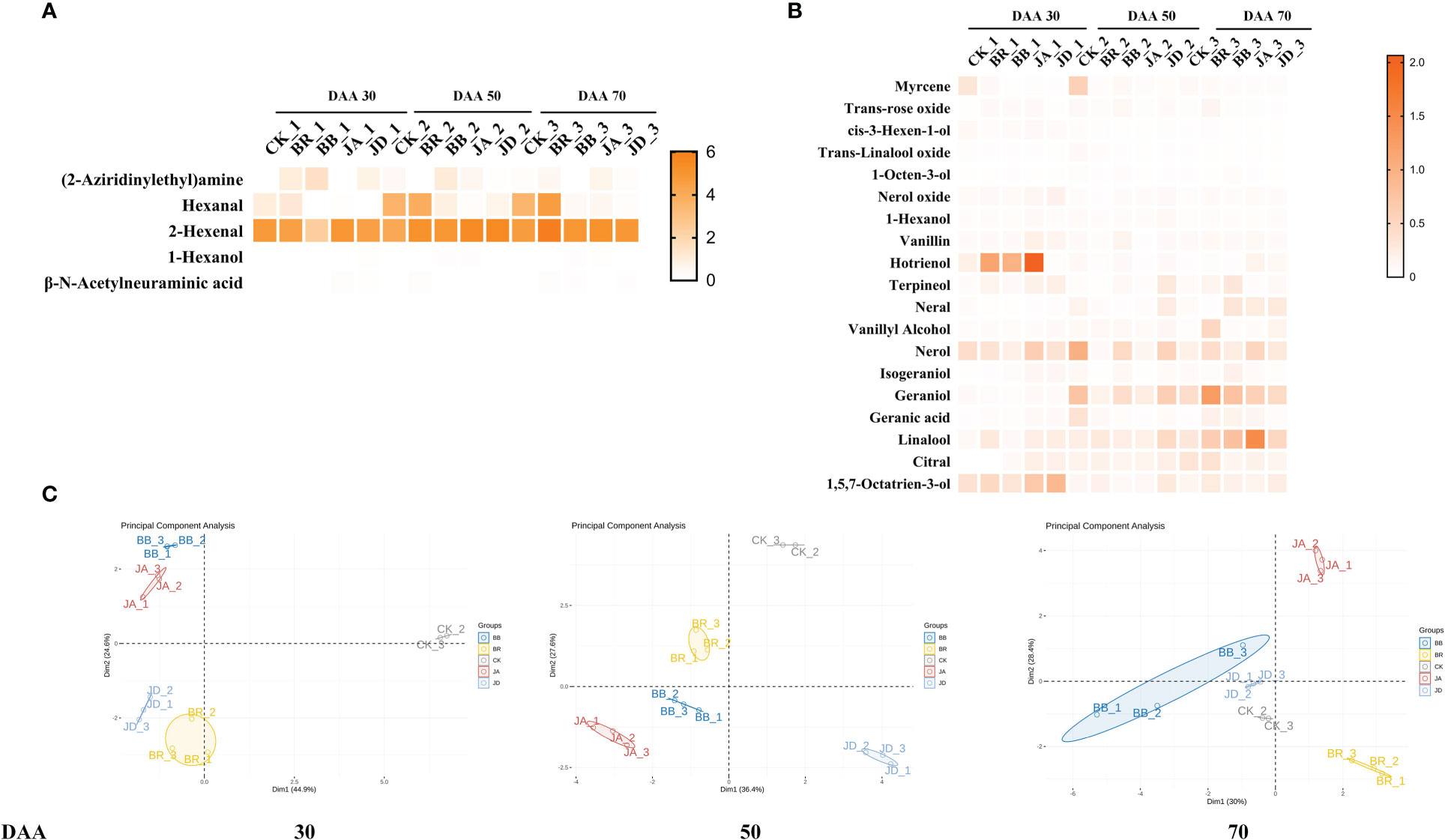
Figure 1 Effects of nutrient solution of different concentrations on grape berry free-form aroma volatiles formation. (A) Alcohol and aldehyde levels. (B) Terpenes levels. (C) PCA analysis. The contents of each aroma substance were shown as heatmap.
To further explore the variability between and within groups of treatments, the PCA analysis was implemented (Figure 1C). The results showed that the degree of parameter’s variation within each treatment group was not significant. However, the degree of parameter’s variation among the CK, BR, JA, BB and JD treatment groups was evident. Thus, we concluded that a high reproducibility was indicated within the groups, and good discrimination was shown between the groups.
After EBR and JA treatment, we found that the concentration of alcohols, aldehyde, and terpenes compounds at fruit ripening stages (DAA 50 and 70) was higher than those in the CK group. Furthermore, a radar map was conducted to display the effect of EBR and JA treatment on the grape aroma. At DAA 30, we found that JA treatment contributed the most to violet aroma (Figure 2A). But at DAA 50, the citrus and rose aromas were hugely promoted by CK treatment (Figure 2B). Moreover, we noticed that BR treatment mainly contributed to rose aroma while JA treatment was responsible for potent citrus and floral aromas at DAA 70 (Figure 2C). Furthermore, BB and JD treatments had different degrees of inhibition effect on the formation of different aromas (Figure 2). For example, at the early fruit ripening stage (DAA 30), both BB and JD treatments inhibited the increasement of citrus and rose aromas (Figure 2A). In case of the late fruit ripening stage (DAA 50 and DAA 70), citrus and flower aromas were decreased when BB and JD application was applied on grapevine (Figures 2B, C).
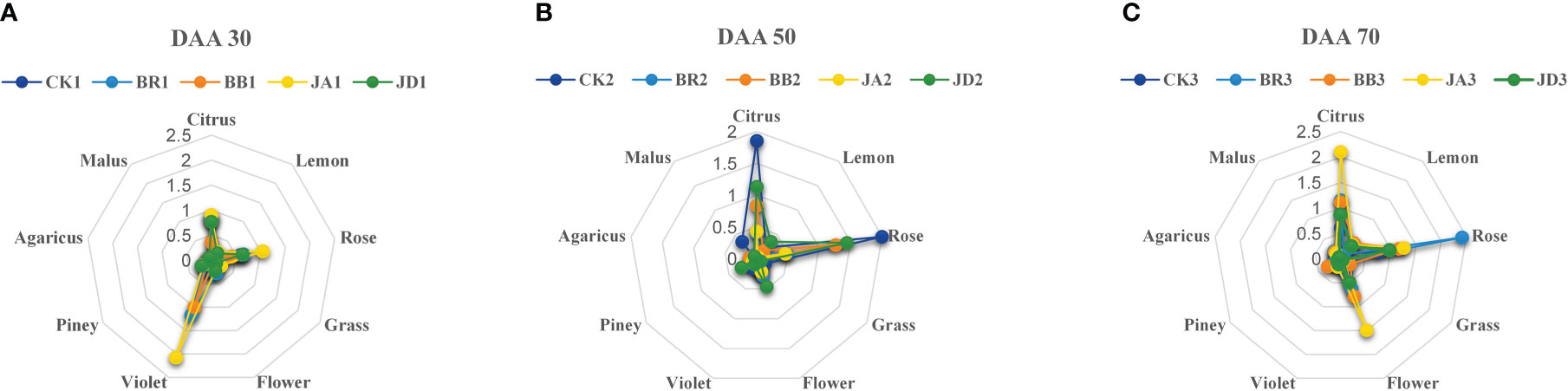
Figure 2 Aromatic series values of grape berries for the different developmental stages under EBR, JA, BB and JD treatments and CK treatments (CK) (n = 3). (A) Aromatic series values at DAA 30. (B) Aromatic series values at DAA 50. (C) Aromatic series values at DAA 70.
Transcriptome analysis of BR and JA treatments on grape berries
As Table 1 showed, 45 cDNA libraries of ‘Ruidu Hongyu’ grape berries used for the RNA-seq, yielded a total of 481.02 G clean data. Also, the range of alignment efficiency was from 92.63% to 96.47%, and the raw reads ranged from 55124564 to 105458290 in JD3_3.
There was a total of 81 DEGs in JA_2 vs. JD_2, a total of 26 DEGs in JA_2 vs. CK_2, a total of 117 DEGs in JA_1 vs. JD_1, a total of 50 DEGs in JA_1 vs. CK_1, a total of 2845 DEGs in JA_3 vs. JD_3, a total of 827 DEGs in JA_3 vs. CK_3, a total of 1336 DEGs in BR_3 vs. CK_3, a total of 438 DEGs in BR_3 vs. Brz_3, a total of 82 DEGs in BR_2 vs. CK_2, a total of 169 DEGs in BR_2 vs. Brz_2, a total of 52 DEGs in BR_1 vs. CK1, a total of 0 DEGs in BR_1 vs. Brz_1. Among them, we found there were more down-regulated DEGs than up-regulated DEGs, except for JA_1 vs. JD_1 (19 down-regulated, and 98 up-regulated), BR_3 vs. CK_3 (600 down-regulated, and 736 up-regulated) and BR_2 vs. Brz_2 (79 down-regulated, and 90 up-regulated) groups (Figure 3A). As shown in Figures 3B, C, the Venn diagram further explained that at the grape ripening stage (DAA 70), the EBR and JA treatment groups had more DEGs than the other groups.
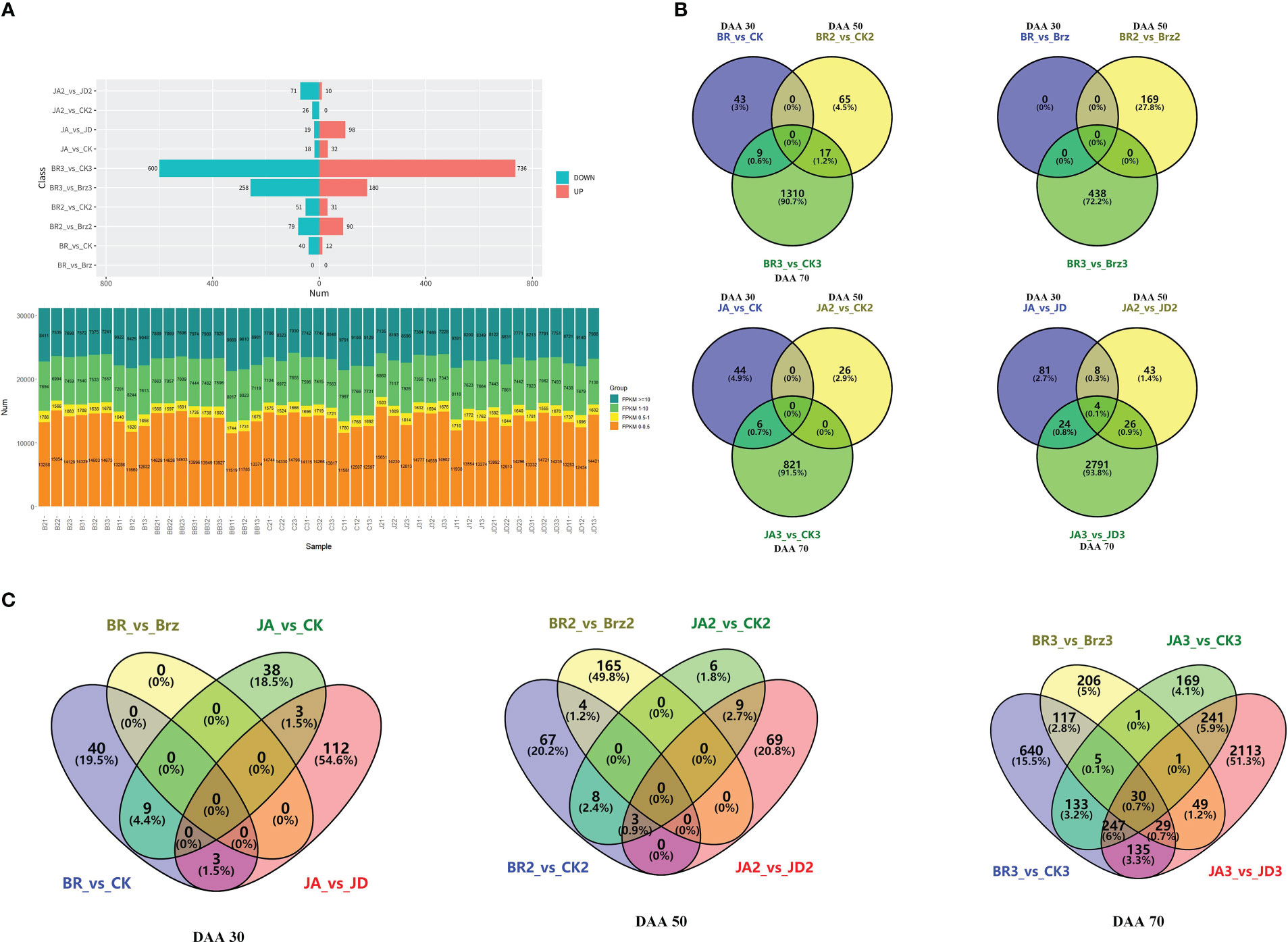
Figure 3 Summary of the number of DEGs identified by RNA-seq analysis under BR/JA/BB/JD treatments vs. CK groups. (A) Up-regulated DEGs and down-regulated DEGs in each comparison group. (B, C) Up-regulated DEGs and down-regulated DEGs in each comparison group are presented by Venn diagrams.
The volcano plots could show the distribution of gene expression level differences between the two groups of samples. There were few up-regulated and down-regulated DEGs observed at DAA30 (Figures 4A, D, G, J), whereas DAA 50 (Figures 4B, E, H, K) and DAA 70 (Figures 4C, F, I, L) had more up-regulated. Moreover, the number of down-regulated DEGs was redundant with that of up-regulated DEGs. Consistent with the results of previous results (Figure 3A), there were also more up-regulated DEGs in the groups of JA_1 vs. JD_1, BR_3 vs. CK_3, and BR_2 vs. Brz_2.
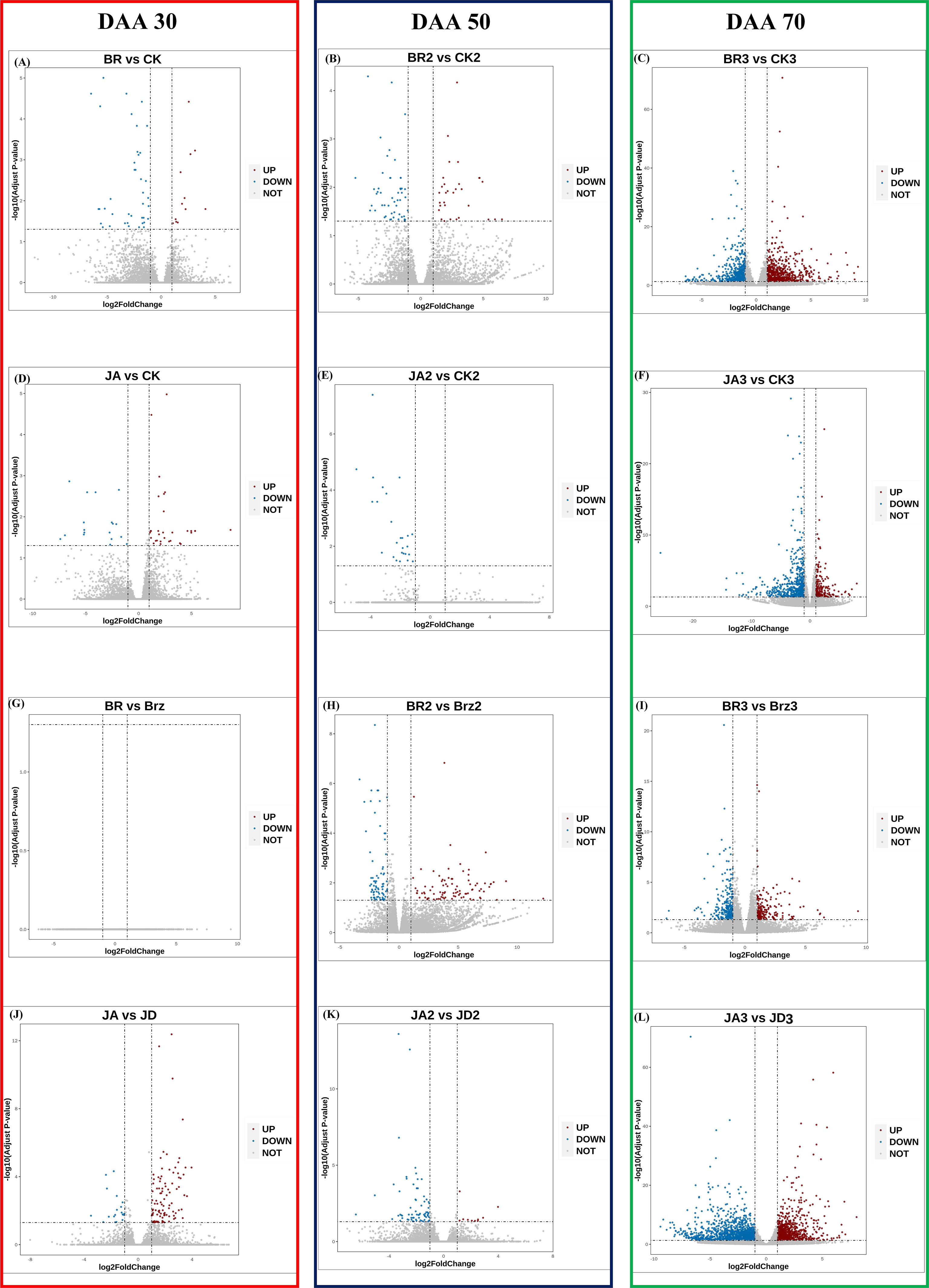
Figure 4 Differential expression volcano map of BR/JA/BB/JD vs. CK at three stages (DAA 30, 50, 70). Each point in the differential expression volcano map represents a gene. The blue dotsrepresented down-regulated DEGs, the red dots represented up-regulated DEGs, and the gray dots represented non-differentially expressed genes. (A, D, G, J) Aromatic series values at DAA 30. (B, E, H, K) Aromatic series values at DAA 50. (C, F, I, L) Aromatic series values at DAA 70.
The functional annotation of databases for DEGs may be better understood through GO functional enrichment analysis. In this study, GO enrichment was displayed in three main categories: biological process (BP), molecular function (MF), and cellular component (CC) (Figure 5). Specifically, more functional action was observed at late fruit ripening stages (DAA 70). The catalytic activity of DEGs was primarily enriched in the BR_3 vs. CK_3 and BR_3 vs. Brz_3 groups, whereas the cell periphery was mainly enhanced in the JA_3 vs. CK_3 and JA_1 vs. JD_1 groups. Moreover, the fact is that EBR and JA encouraged the accumulation of secondary metabolites in grapevine in the form of alcohols, aldehydes, and terpenes. Above results from the side confirmed that at the late fruit ripening stage, EBR and JA promoted a large amount of volatiles accumulation.
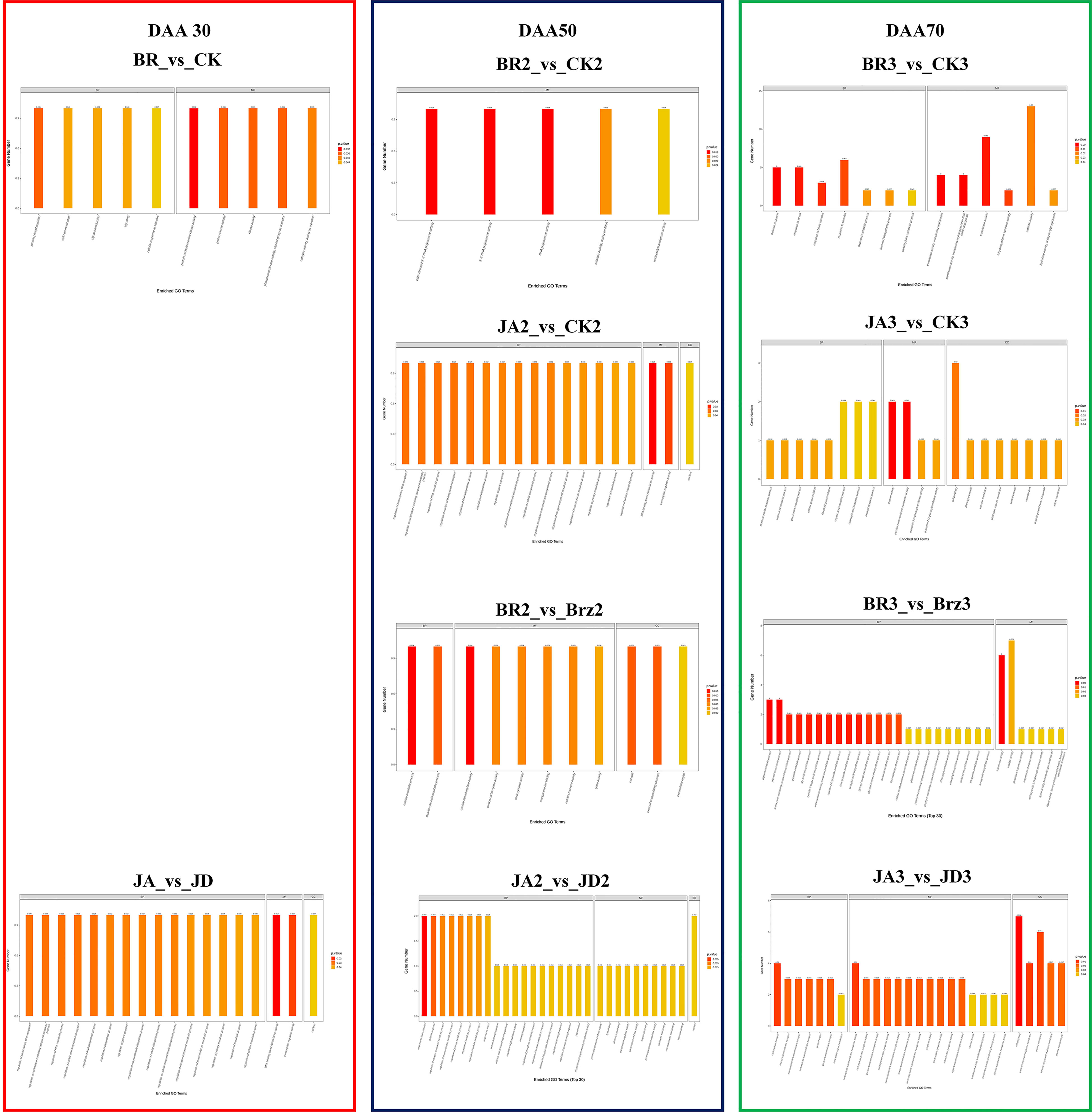
Figure 5 Gene ontology (GO) enrichment of DEGs among different comparison groups (BR/JA/BB/JD treatments vs. CK). The DEGs were enriched into three classifications of biological process (BP), molecular function (MF), and cellular components (CC).
Unlike GO enrichment analysis, KEGG not only has gene sets but also defines the complex interrelationship between related genes and metabolites. In accordance with Figure 6, the RNA polymerase process and metabolic pathway, plant hormone signal transduction, and biosynthesis of secondary metabolites were the main areas where DEGs were enriched in the BR_2 vs. CK_2 group and BR_3 vs. CK_3 group, respectively. Regarding JA_2 vs. CK_2 group, DEGs were mainly enriched in the ribosome pathway. In addition, there were found more DEGs that were enriched in metabolic pathways and secondary metabolite biosynthesis in the JA_3 vs. CK_3 group. These findings further demonstrated that EBR and JA could promote the accumulation of multiple secondary metabolites and the occurrence of multiple metabolic activities in grapevines. And both EBR and JA treatments might promote the metabolism of fatty acids or amino acids at the late stage of fruit ripening.
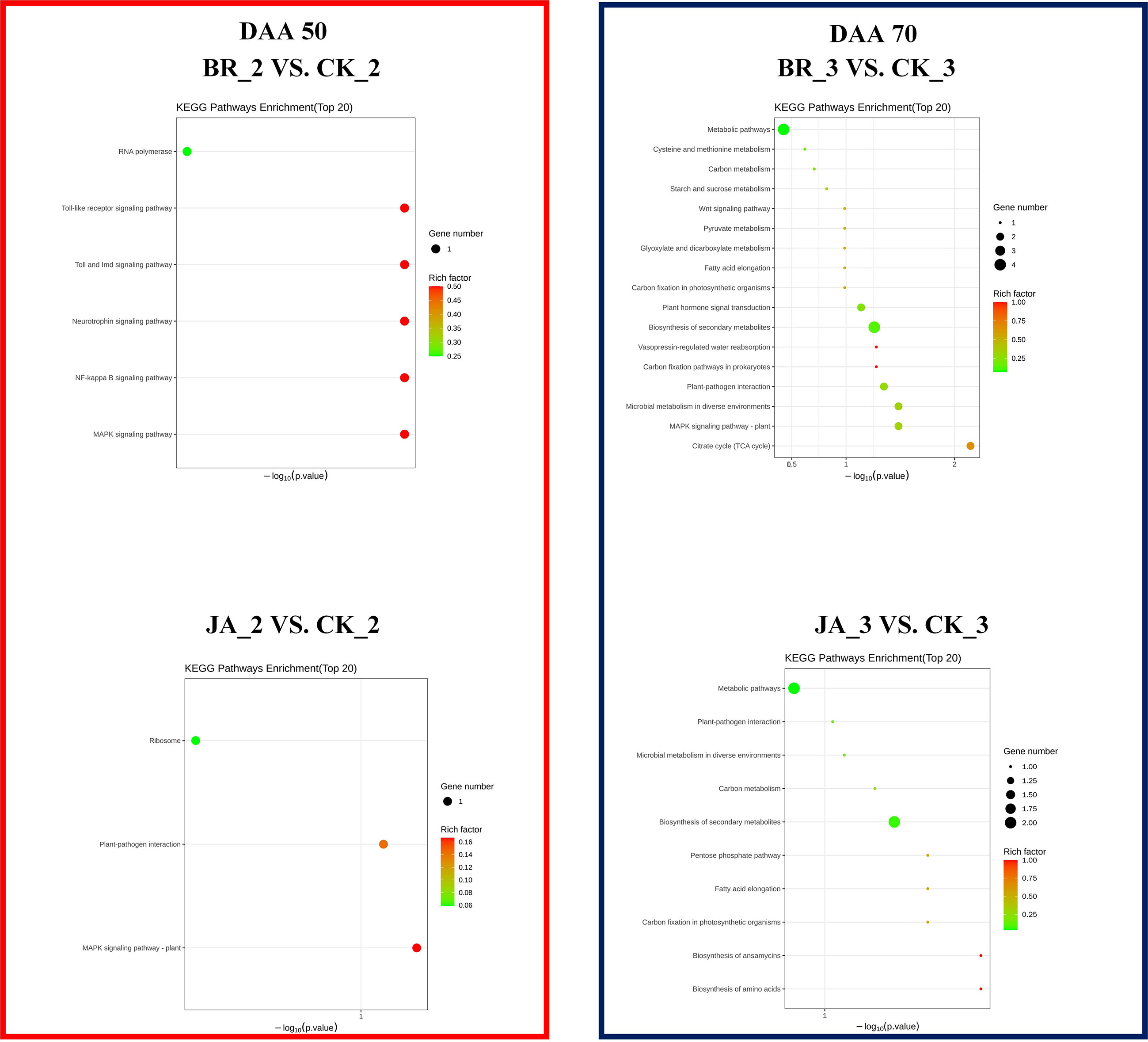
Figure 6 KEGG enrichment analysis of the DEGs among BR_2 vs. CK_2, BR_3 vs. CK_3, JA_2 vs. CK_2, JA_3 vs. CK_3 treatments.
DEGs involved in terpene, alcohol and aldehyde biosynthesis and metabolism
In this study, exogenous EBR and JA had a significant impact on the biosynthesis of terpenes, alcohols, and aldehydes. To further understand the effect of exogenous EBR and JA on aroma compounds biosynthesis and metabolism-related genes at the transcription level, heat map analysis was carried out based on the results of the transcriptome analysis (Figure 7A). The information of specific key gene selected in this study was shown in Table 2. In this study, the pattern of gene expression by performing a K-means cluster analysis on the time series for genes related to terpene, alcohol, and aldehyde biosynthesis. There were two clusters formed, and cluster 1 was characterized by a significant increase in transcript levels at DAA 30 for both the EBR and JA treatments. Interestingly, terpene biosynthesis genes like LOC100247263, LOC100259617, LOC100232975, and LOC100855049 were upregulated by BB and JD treatments, which also contributed to terpene accumulation at DAA 30. Moreover, BB and JD treatments also contributed to terpene accumulation at DAA 30 and up-regulated the expression of genes related to terpene biosynthesis, including LOC100247263, LOC100259617, LOC100232975, and LOC100855049. When exposed to EBR and JA at DAA 70, the terpene biosynthesis genes in cluster 2 (LOC100247834, LOC104878767, and LOC100855133) showed a noticeably increased transcript level. These genes were also expressed more strongly in the BB and JD groups than in the CK group at DAA 70.
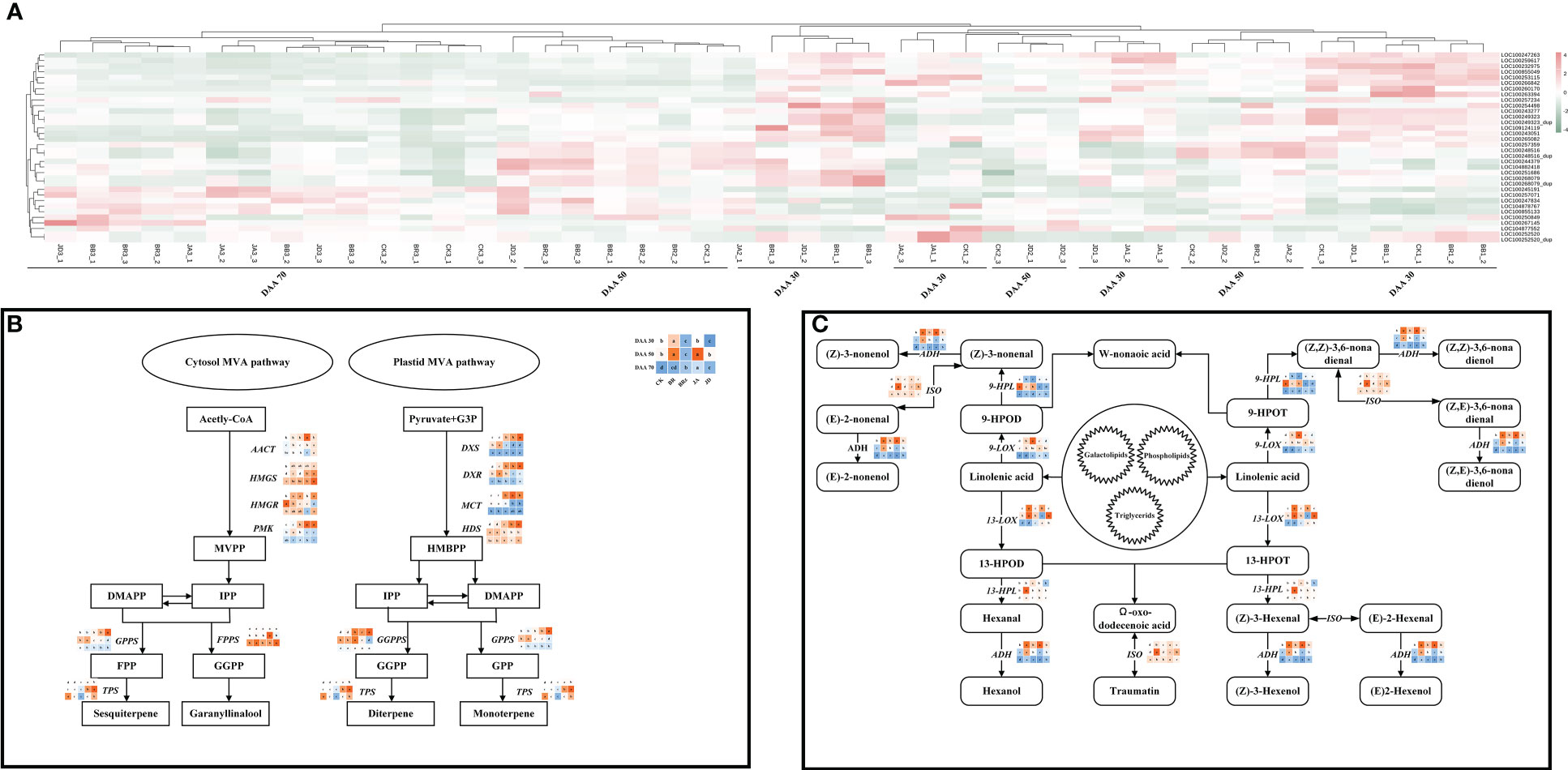
Figure 7 Heatmap and key gene expression profiles involved in the terpene/alcohol/aldehyde biosynthesis pathways. (A) Heat map for key genes involved in the biosynthesis of terpene compounds. (B) Key gene expression profiles involved in the terpene biosynthesis pathways. (C) Key gene expression profiles involved in the alcohol and aldehyde biosynthesis pathways.
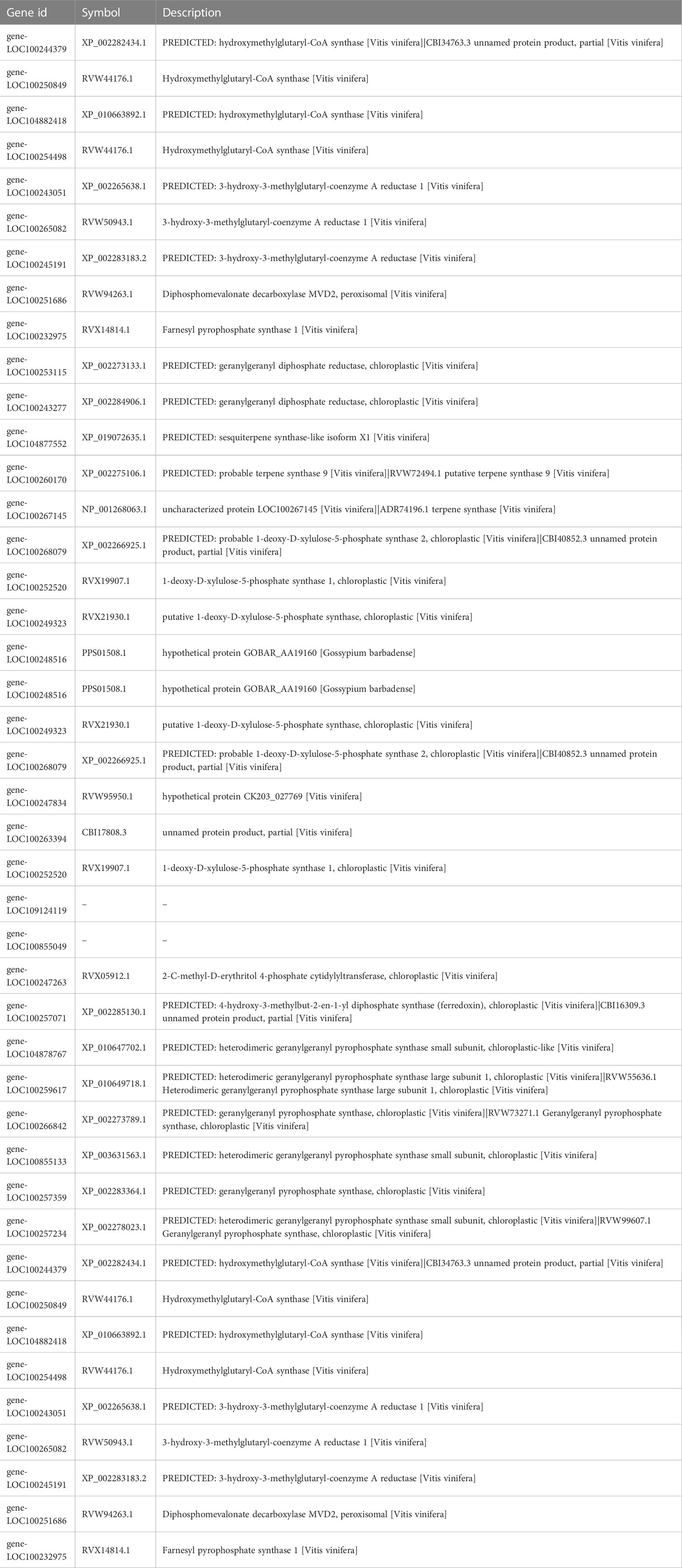
Table 2 The information of key genes in aroma volatiles (terpenes, alcohol, aldehyde) biosynthesis pathways.
The qRT-PCR analysis was conducted to further confirm the regulation mechanism of EBR and JA on aroma substance biosynthesis. The expression of AACT, HMGS (responsible for MVPP accumulation), FPPS (responsible for geranyl linalool accumulation), and TPS (responsible for sesquiterpene accumulation) genes in the MVA pathway (cytosol) were up-regulated under EBR and JA treatments at DAA 30 and DAA 50 (Figure 7B). Moreover, the higher expression of DXS, DXR, MCT, HDS (responsible for HMBPP accumulation), GGPPS, and TPS (responsible for diterpene accumulation) were also found in the MVA pathway (plastid) under EBR and JA treatments at DAA 30. It is noteworthy that BB and JD treatments down-regulated the expression of these genes throughout the study. There was a total of six genes whose expressions were found in relation to the patterns of alcohol and aldehyde biosynthesis and metabolism shown in Figure 7C. According to the findings, EBR and JA treatments were responsible to increase the expression of ADH, 9-LOX, and 13-HPL at DAA 30 and DAA 50, whereas the expression of 9-HPL and 13-LOX was found higher at DAA 70. In addition, we found EBR and JA treatments promoted ISO expression at all sampling stages. Overall, BB and JD application consistently decreased the expressions of these genes.
DEGs involved in BR and JA biosynthesis, metabolism and signaling
Using exogenous EBR and JA application must influence endogenous BR and JA biosynthesis. In this study, the gene expression patterns of BR and JA biosynthesis and metabolism were investigated in the basis of K-means value. The specific information of key gene selected in this study was shown in Table 3. As Figure 8A displayed, there were also 2 clusters generated in BR biosynthesis pathway, cluster 2 was identified by an increase in transcript (LOC100257818, LOC100259184, LOC100263507, LOC100263507, LOC100261294, LOC100241065, LOC100250571, BR6OX1, LOC100260924, LOC100257474, LOC100259945, LOC100261207, LOC100262602, LOC100243702, LOC100259060, LOC100245678) under both EBR and JA treatments at DAA 30. Differently, cluster 1 was determined by an increase in transcript (LOC100248934, LOC100249741, LOC100258692) under EBR and JA treatments at DAA 70. Moreover, we found treatments containing signaling inhibitors (BB and JD) could effectively inhibit these gene expressions at all sampling stages. As Figure 8B displayed, there were also 2 clusters generated in JA biosynthesis pathway. Cluster 1, which contained LOC100249741, LOC104879884, LOC100854662, was identified by an increase of transcript in EBR and JA treatment groups at DAA 70. Furthermore, cluster 2, which included LOC100253078, LOC104880706, LOC109122580, LOC104878952, was defined by an increase of transcript in EBR treatment group at DAA 30.
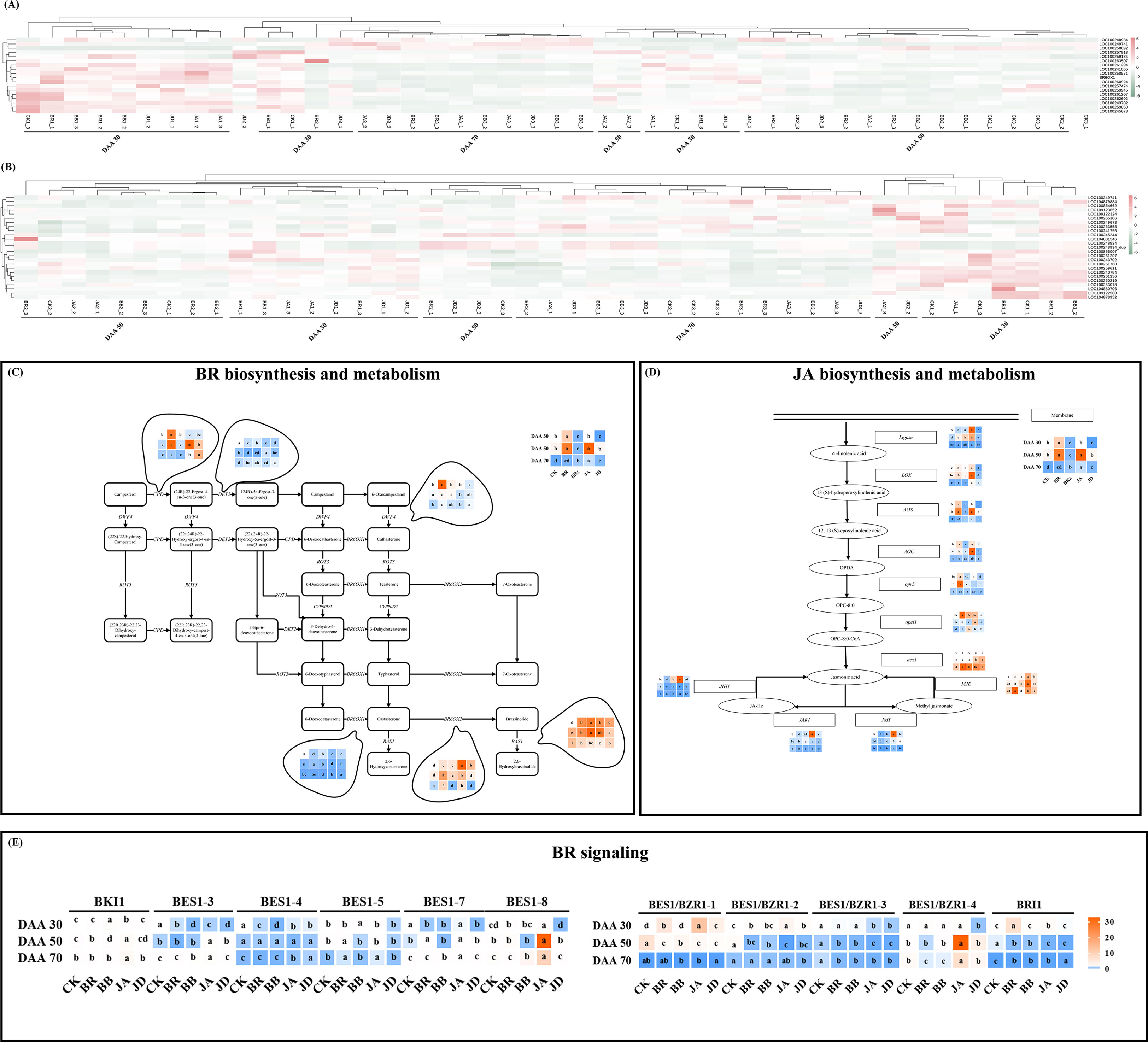
Figure 8 Heatmap and key gene expression profiles involved in the BR/JA biosynthesis, metabolism/signaling pathways. (A) Heat map for key genes involved in the biosynthesis of endogenous BR. (B) Heat map for key genes involved in the biosynthesis of endogenous JA. (C) Key gene expression profiles involved in the BR biosynthesis and metabolism pathways. (D) Key gene expression profiles involved in JA biosynthesis and metabolism pathways. (E) Key transcription factors expression profiles involved in BR signaling pathways.
In addition, qRT-PCR was carried out to further verify the effect of EBR and JA on BR/JA biosynthesis and metabolism at transcription level. There are six genes involved in BR biosynthesis and metabolism, and eleven transcription factors related to BR signaling. We found DET2 and DWF4 (upstream genes for BR biosynthesis), BR6OX1 and BR6OX2 (downstream genes for BR biosynthesis), were up-regulated under EBR and JA treatments at DAA 70. CPD (upstream gene for BR biosynthesis) and BAS1 (key gene for BR metabolism) were up-regulated under EBR and JA treatments at DAA 50 (Figure 8C). Moreover, as far as BR signaling is concerned, BKI1/BES1-3/BES1-5/BES1-7/BES1-8 were up-regulated under EBR and JA treatments at DAA 70, while BES1/BZR1-1/2/4, as well as, BRI1 was up-regulated under EBR and JA treatments at DAA 30 (Figure 8E). In addition, we found BB and JD treatments inhibited most of the above gene expressions, especially at early fruit ripening stages (DAA 30).
In this study, we also quantified the expression patterns of genes for JA biosynthesis and metabolism. The results illustrated that Ligase, LOX, AOS, AOC, opcl1, acx1 (genes for JA biosynthesis) were expressed at a higher level in EBR and JA groups at DAA 50. At DAA 30, the expression levels of opr3 and MJE, which contributed to JA biosynthesis, also increased under EBR and JA application. Moreover, exogenous EBR down-regulated the expressions of JAR1 and JMT (genes for JA metabolism) at DAA 30 and DAA 70, while exogenous JA significantly up-regulated them at DAA 30 (Figure 8D). More consistently, the above gene expression was inhibited by BB and JD treatments, especially at late fruit ripening stages (DAA 50 or DAA 70).
Discussion
Exogenous EBR and JA promoted biochemical properties of grape berries
Exogenous phytohormones are used to improve the berries’ quality while the grapevine is growing and developing. According to prior research, EBR could significantly increase grape berry size and sugar content as well as improved grape color and flavor formation (Li et al., 2022; Lv et al., 2022a). Similarly, JA could also contribute to berry expansion, sugar accumulation, and anthocyanins formation (Yu et al., 2020; Gao et al., 2022; Wang et al., 2022). In agreement with the results of previous research (Li et al., 2022), this study further verified the results that EBR and JA also promoted the biochemical properties of ‘Ruidu Hongyu’ grapevine. We investigated more closely than in the previous study to assess the effect of EBR and JA on the biosynthesis of grape berry free-form aroma volatiles. The results revealed that exogenous EBR and JA had a significant promoting effect on free-form aroma substances (aldehydes, hotrienol, terpineol, nerol, geraniol, linalool, and citral) at late fruit ripening stages (DAA 70). Moreover, it was found that exogenous EBR and JA were primarily responsible for the production of rose-aroma characteristics of grape berries. The aforementioned findings supported earlier research that showed exogenous EBR and JA promoted aroma volatilization and flavor improvement (Xi et al., 2013; Li et al., 2022; Wang et al., 2022).
Transcriptome sequencing revealed exogenous EBR and JA accelerated aroma substances accumulation of grape berries
Sequencing quality revealed sequencing depth and repeatability (Barbitoff et al., 2020). In this study, the amount of high-quality data of the sequenced sample was more than 481.02 G worth, the Q30 base distribution was more than 92%, clean reads rate mapped to the grapevine genome was more than 90%. In all, we concluded that the quality and depth of the sequenced samples were high. Moreover, the outstanding repeatability among the samples was illustrated by the correlation coefficient (greater than 0.99). During the whole development of grapevine, there was a total of 3043 DEGs in JA vs. JD, a total of 903 DEGs in JA vs. CK, a total of 1470 DEGs in BR vs. CK, and a total of 607 DEGs in BR vs. Brz. Also, we found that only BR vs. CK and JA vs. CK groups at DAA 70 had more DEGs. In addition, there were more DEGs were down-regulated in JA_2 vs. JD_2, JA_2 vs. CK_2, BR_3 vs. Brz_3, BR_2 vs. CK2, BR vs. CK groups. The volcano plots further intuitively corroborated our conclusion.
GO enrichment analysis revealed the process of activities to which multiple DEGs responded (Yan et al., 2019; Zhu et al., 2019). Previous researchers’ findings suggested that EBR and JA promoted the accumulation of several terpenes and aldehydes (Li et al., 2021; Liu et al., 2022). The formation of secondary metabolites and catalytic activity were the main areas of enrichment for DEGs in comparison groups, and a similar result could be obtained by combining transcriptome data analysis (GO enrichment analysis). In the process of bioinformatics analysis, KEGG pathway enrichment analysis is often applied to the functional annotation of differentially expressed genes. Thus, the related functions and action pathways of differentially expressed genes were constructed (Yang et al., 2018; Jie et al., 2020). In this study, multiple secondary metabolites formation and the occurrence of various metabolic activities in grapevines were affected by exogenous EBR and JA. These further authenticated that the increase of terpenes and aldehydes contents might be regulated by exogenous EBR and JA signals. The outcomes were more consistent with previous findings that the exogenous EBR and JA were involved in regulating the biosynthesis of several metabolites, including monosaccharides, anthocyanins, fatty acids, amino acids, and so on, with significantly higher levels than the CK group (Mao et al., 2017; Vergara et al., 2018; Nakajima et al., 2021; Chen et al., 2022; Li et al., 2022; Lv et al., 2022a). Results of this study provided reference information for verifying the interaction of EBR and JA in regulating aroma substances biosynthesis. Therefore, DEGs identified in this study were worthy of further studies on the function of genes interactions to mediate various life activities in grapevine.
Exogenous EBR and JA up-regulated the expressions of key genes related to aroma substances biosynthesis
EBR was reported as an effective phytohormone that had a significant impact on the aroma substances by up-regulating the gene expressions responsible for terpenes biosynthesis (VvHMGR) (Zheng et al., 2020). Moreover, the scientist explained that amino acid volatilization was accelerated by up-regulating the expression of the genes involved in amino acid biosynthesis (Lv et al., 2022b). Regarding JA, it also increased the genes expressions of PgIPPI and PgFPS, which promoted the accumulation of terpenes such as geraniolene, limonene, panasinsene, elemene, farnesane, alpha carophyllene, beta carophyllene, germacrene, bulsenol, citronellal, and falcrinol (Balusamy et al., 2015). Furtherly, to better understand how EBR and JA control the enrichment of aroma metabolites, qRT-PCR assays were used to measure the expression of key genes involved in the biosynthesis and metabolism of terpenes, alcohol, and aldehydes. In the early stage, most genes related to terpenes and aldehyde biosynthesis and metabolism could be divided into two clusters through transcriptome sequencing. At DAA 30 and 70, EBR and JA increase the expressions of most key genes involved in aroma substances biosynthesis. Through qRT-PCR, we further confirmed that EBR and JA had a facilitation effect on the expression of terpene and aldehyde biosynthetic genes. Genes involved in the MVA pathway (AACT, HMGS, FPPS) were up-regulated under both EBR and JA treatments at late fruit ripening stages (DAA 50). In the MVA pathway, both EBR and JA treatments increased the expression of genes such as DXS, DXR, MCT, HDS, FPPS, and GGPPS at the start of fruit ripening (DAA 30). The ISO, 9-LOX, and 13-HPL genes responsible for the formation of alcohol and aldehydes were also expressed at a higher level at DAA 50 in response to EBR and JA treatments. The relative expression levels were consistent with the overall trend of deep sequencing data. Further, the validity of the RNA Seq gene expression profiles was confirmed by qRT-PCR tests. Therefore, we concluded exogenous EBR and JA promoted the accumulation of aroma substances at late stages of fruit ripening. Moreover, the expression of most structural genes related to the biosynthesis of terpenes, alcohol and aldehydes were up-regulated. Previous studies illustrated that 100 μmol L-1 of exogenous BR promoted HMGR activity at veraison stages of five-year-old ‘Kyoho’ grapevine (Zheng et al., 2020) while 10 mM application of MeJA significantly up-regulated DXS, HMGCR, TPS14 in the monoterpene biosynthesis pathway in grapevine (Li et al., 2020). Interestingly, we found that EBR and JA signaling inhibitors effectively reversed the gain effect of EBR and JA on the accumulation of aroma volatiles in grape berries. Therefore, we inferred that exogenous EBR and JA might accelerate the accumulation of aroma volatiles and grape flavor by enhancing the expression of several transcription factors, which were related to signal transduction of terpenes and other aroma compounds.
Exogenous EBR and JA up-regulated the expressions of key genes related to BR/JA biosynthesis, metabolism and signaling
The application of exogenous phytohormones causes changes in the content of endogenous phytohormones, as well as the enhancement of phytohormones signal transduction (Fahad et al., 2015). It had been reported that EBR treatment up-regulated the expression of BR biosynthesis-related gene expressions (CYP85A1, CYP85A3). Concurrently, the expressions of BR signaling receptor proteins (BZR1-1D) were enhanced (Liu et al., 2014). In addition, exogenous JA is also responsible for boosting the accumulation of endogenous JA due to the up-regulated expression of JA synthetic structural genes and JA signaling receptors (Qiu et al., 2020). On the basis of transcriptome data, we concluded that exogenous EBR and JA significantly contribute to the rise in endogenous BR and JA contents at the late stage of berry ripening. Furthermore, qRT-PCR demonstrated that EBR and JA treatments at DAA 70 increased the expression of two key genes (BR6OX1 and BR6OX2) that control BR biosynthesis. Six essential genes for JA biosynthesis, including ligase, LOX, AOS, AOC, opcl1 and acx1, were expressed at higher levels in the EBR and JA groups at DAA 50. Moreover, BKI1, BES1-3, BES1-5, BES1-7, and BES1-8, which were recognized as key transcription factors for BR signaling, were up-regulated under EBR and JA treatments at DAA 70. The relative expression levels were consistent with the overall trend of deep sequencing data, as well. Previous studies illustrated that 0.40 mg L-1 of 24-Epibrassinolide treatment altered endogenous BR accumulation in ‘Cabernet Sauvignon’ grapevine. The expressions of BRs biosynthetic enzymes (VvBR6OX1 and VvDWF1) were down-regulated, while the expression of BR receptor gene (VvBRI1) was up-regulated (Xu et al., 2015). Moreover, 10 mM of MeJA significantly up-regulated expression levels of LOX2S, AOS, OPR, and JMT in the JA biosynthesis pathway (Li et al., 2020). These findings were accordant with the results obtained in this study. These findings led us to the conclusion that exogenous EBR and JA enhanced the expression of key genes involved in BR/JA biosynthesis, metabolism, and signaling, resulting in the enrichment of endogenous BR and JA in the grapevine. Furthermore, we found that inhibitors of EBR and JA signaling efficiently reversed the gain effect of EBR and JA on the biosynthesis of endogenous BR and JA in grape berries. We can summarize from this that EBR and JA may influence BR and JA signal transduction in a manner that causes endogenous BR and JA levels to rise at the late stages of berry ripening.
Conclusion
Overall, exogenous EBR and JA application not only promoted improvement of external qualities (berries expansion and coloration) but also significantly increased the content of free-form aroma substances (terpenes, alcohol, aldehyde). The mechanism by which EBR and JA control the accumulation of aroma volatiles has been better understood through transcriptome sequencing. A total of 3043, 903, 1470 and 607 DEGs were identified in JA vs. JD, JA vs. CK, BR vs. CK, BR vs. Brz, respectively. There were more DEGs under both EBR and JA treatments at late fruit ripening stages than at early fruit ripening stages. Moreover, qRT-PCR revealed that the expression of various genes involved in the biosynthesis of terpenes, alcohol, and aldehydes was increased in response to the EBR and JA treatments. Most of the genes involved in BR and JA biosynthesis and signaling were up-regulated concurrently. In conclusion, these findings provide new insight for the investigation of the DEGs (responsible for aroma substances enrichment) under EBR and JA treatments. Further investigation is required into the molecular mechanisms affecting the interaction of phytohormones in improving grape flavor.
Data availability statement
The datasets presented in this study can be found in online repositories. The names of the repository/repositories and accession number(s) can be found in the article/Supplementary Material.
Author contributions
JL carried out all experiments, data analyses, draft composed and figures drawn. ZW and YQ ground samples. JH and YZ provided all experimental materials. CM, SJ, CZ reviewed the manuscript. LW and HJ designed the experiments and provided ideas. SW supervised this study. All authors contributed to the article and approved the submitted version.
Funding
This study was supported by the Action Plan for Science and Technology Innovation of Shanghai Science and Technology Commission (Grant No. 22015820400); Ningxia Hui Autonomous Region Key Project of Research and Development Plan (Grant No.2022BBF02013); The Work Station for characteristic grape industries in Wenping Xu plateau, Yunnan (Grant No. YSZJGZZ-2021061); The China Agriculture Research System of MOF and MARA (CARS-29-ZP-7).
Acknowledgments
All authors sincerely appreciated the reviewers and editors for their careful revising and selfless assistance. In addition, we were also appreciated to those who selflessly provide help with this research. And, we show grateful to Shanghai Jiao Tong University, who provide facility to help in sampling and in experiment.
Conflict of interest
The authors declare that the research was conducted in the absence of any commercial or financial relationships that could be construed as a potential conflict of interest.
Publisher’s note
All claims expressed in this article are solely those of the authors and do not necessarily represent those of their affiliated organizations, or those of the publisher, the editors and the reviewers. Any product that may be evaluated in this article, or claim that may be made by its manufacturer, is not guaranteed or endorsed by the publisher.
Supplementary material
The Supplementary Material for this article can be found online at: https://www.frontiersin.org/articles/10.3389/fpls.2023.1185049/full#supplementary-material
Supplementary Figure 1 | Berry phenotype and physicochemical parameters from different sampling stages under different treatments. (A) Berry phenotype. Berries were collected at DAA 30, 50, and 70, taken by Canon camera (TKY, JPN). (B) External and internal quality parameters, including longitudinal diameter, transverse diameter, single berry weight, total soluble solid (TSS), and titratable acids (TA).
Supplementary Table 1 | Primers for qRT-PCR. The sequences of key genes in BR/JA biosynthesis and aroma substances biosynthesis pathways were shown in the table.
Supplementary Table 2 | The information and retention time of free monoterpenes detected in this study.
References
Balusamy, S. R. D., Rahimi, S., Sukweenadhi, J., Kim, Y. J., Yang, D. C. (2015). Exogenous methyl jasmonate prevents necrosis caused by mechanical wounding and increases terpenoid biosynthesis in panax ginseng. Plant Cell Tissue Organ Culture (PCTOC) 123, 341–348. doi: 10.1007/s11240-015-0838-8
Barbitoff, Y. A., Polev, D. E., Glotov, A. S., Serebryakova, E. A., Shcherbakova, I. V., Kiselev, A. M., et al. (2020). Systematic dissection of biases in whole-exome and whole-genome sequencing reveals major determinants of coding sequence coverage. Sci. Rep. 10, 2057. doi: 10.1038/s41598-020-59026-y
Chen, C., Wu, X. M., Pan, L., Yang, Y. T., Dai, H. B., Hua, B., et al. (2022). Effects of exogenous α-naphthaleneacetic acid and 24-epibrassinolide on fruit size and assimilate metabolism-related sugars and enzyme activities in giant pumpkin. Int. J. Mol. Sci. 23, 13157. doi: 10.3390/ijms232113157
Chigo-Hernandez, M. M., DuBois, A., Tomasino, E. (2022). Aroma perception of rose oxide, linalool and α-terpineol combinations in gewürztraminer wine. Fermentation 8, 30. doi: 10.3390/fermentation8010030
Englund, E., Shabestary, K., Hudson, E. P., Lindberg, P. (2018). Systematic overexpression study to find target enzymes enhancing production of terpenes in synechocystis PCC 6803, using isoprene as a model compound. Metab. engineering 49, 164–177. doi: 10.1016/j.ymben.2018.07.004
Fahad, S., Hussain, S., Matloob, A., Khan, F. A., Khaliq, A., Saud, S., et al. (2015). Phytohormones and plant responses to salinity stress: a review. Plant Growth regulation 75, 391–404. doi: 10.1007/s10725-014-0013-y
Gao, Y., Wang, X., Liu, X., Liang, Z. (2022). Hormone biosynthesis and metabolism members of 2OGD superfamily are involved in berry development and respond to MeJA and ABA treatment of vitis vinifera l. BMC Plant Biol. 22, 1–17. doi: 10.1186/s12870-022-03810-7
Javed, H. U., Wang, D., Shi, Y., Wu, G. F., Xie, H., Pan, Y. Q., et al. (2018). Changes of free-form volatile compounds in pre-treated raisins with different packaging materials during storage. Food Res. Int. 107, 649–659. doi: 10.1016/j.foodres.2018.03.019
Javed, H. U., Wang, D., Wu, G. F., Kaleem, Q. M., Duan, C. Q., Shi, Y., et al. (2019). Post-storage changes of volatile compounds in air-and sun-dried raisins with different packaging materials using HS-SPME with GC/MS. Food Res. Int. 119, 23–33. doi: 10.1016/j.foodres.2019.01.007
Jie, K., Feng, W., Boxiang, Z., Maofeng, G., Jianbin, Z., Zhaoxuan, L., et al. (2020). Identification of pathways and key genes in venous remodeling after arteriovenous fistula by bioinformatics analysis. Front. Physiol. 11. doi: 10.3389/fphys.2020.565240
Jin, X. Q., Feng, M. X., Guo, S. H., Chen, X. Y., Zheng, H. T., Dong, X. Y., et al. (2022). Evolution of aroma profiles and potential in shine Muscat grape berries during ripening. South Afr. J. Enol. Viticult. 43, 113–124. doi: 10.21548/43-2-5103
Kou, X., Feng, Y., Yuan, S., Zhao, X., Wu, C., Wang, C., et al. (2021). Different regulatory mechanisms of plant hormones in the ripening of climacteric and non-climacteric fruits: a review. Plant Mol. Biol. 107, 477–497. doi: 10.1007/s11103-021-01199-9
Li, S., Chen, K., Grierson, D. (2021). Molecular and hormonal mechanisms regulating fleshy fruit ripening. Cells 10, 1136. doi: 10.3390/cells10051136
Li, J., Javed, H. U., Wu, Z., Wang, L., Han, J., Zhang, Y., et al. (2022). Improving berry quality and antioxidant ability in ‘Ruidu hongyu’grapevine through preharvest exogenous 2, 4-epibrassinolide, jasmonic acid and their signaling inhibitors by regulating endogenous phytohormones. Front. Plant Sci. 13. doi: 10.3389/fpls.2022.1035022
Li, W., Li, W., Yang, S., Ma, Z., Zhou, Q., Mao, J., et al. (2020). Transcriptome and metabolite conjoint analysis reveals that exogenous methyl jasmonate regulates monoterpene synthesis in grape berry skin. J. Agric. Food Chem. 68, 5270–5281. doi: 10.1021/acs.jafc.0c00476
Li, J., Quan, Y., Wang, L., Wang, S. (2023). Brassinosteroid promotes grape berry quality-focus on physicochemical qualities and their coordination with enzymatic and molecular processes: a review. Int. J. Mol. Sci. 24, 445. doi: 10.3390/ijms24010445
Li, X. Y., Wen, Y. Q., Meng, N., Qian, X., Pan, Q. H. (2017). Monoterpenyl glycosyltransferases differentially contribute to production of monoterpenyl glycosides in two aromatic vitis vinifera varieties. Front. Plant Sci. 8. doi: 10.3389/fpls.2017.01226
Liu, L., Jia, C., Zhang, M., Chen, D., Chen, S., Guo, R., et al. (2014). Ectopic expression of a BZR1-1D transcription factor in brassinosteroid signalling enhances carotenoid accumulation and fruit quality attributes in tomato. Plant Biotechnol. J. 12, 105–115. doi: 10.1111/pbi.12121
Liu, X., Li, D., Li, Y., Li, S., Zhao, Z. (2022). Brassinosteroids are involved in volatile compounds biosynthesis related to MdBZR1 in ‘Ruixue’(Malus× domestica borkh.) fruit. Postharvest Biol. Technol. 189, 111931. doi: 10.1016/j.postharvbio.2022.111931
Luo, J., Brotchie, J., Pang, M., Marriott, P. J., Howell, K., Zhang, P. (2019). Free terpene evolution during the berry maturation of five vitis vinifera l. cultivars. Food Chem. 299, 125101. doi: 10.1016/j.foodchem.2019.125101
Lv, J., Dong, T., Zhang, Y., Ku, Y., Zheng, T., Jia, H., et al. (2022a). Metabolomic profiling of brassinolide and abscisic acid in response to high-temperature stress. Plant Cell Rep. 41, 935–946. doi: 10.1007/s00299-022-02829-2
Lv, M., Sun, X., Li, D., Wei, G., Liu, L., Chen, F., et al. (2022b). Terpenoid biosynthesis in dendrobium officinale: identification of (E)-β-caryophyllene synthase and the regulatory MYB genes. Ind. Crops Products 182, 114875. doi: 10.1016/j.indcrop.2022.114875
Mao, J., Li, W., Mi, B., Dawuda, M. M., Calderón-Urrea, A., Ma, Z., et al. (2017). Different exogenous sugars affect the hormone signal pathway and sugar metabolism in “Red Globe”(Vitis vinifera l.) plantlets grown in vitro as shown by transcriptomic analysis. Planta 246, 537–552. doi: 10.1007/s00425-017-2712-x
Mukherjee, M., Blair, R. H., Wang, Z. Q. (2022). Machine-learning guided elucidation of contribution of individual steps in the mevalonate pathway and construction of a yeast platform strain for terpenoid production. Metab. Engineering 74, 139–149. doi: 10.1016/j.ymben.2022.10.004
Nakajima, N., Inoue, H., Koshita, Y. (2021). Effects of exogenous methyl jasmonate and light condition on grape berry coloration and endogenous abscisic acid content. J. pesticide science 46, 322–332. doi: 10.1584/jpestics.D21-027
Pinu, F. R., Tumanov, S., Grose, C., Raw, V., Albright, A., Stuart, L. (2019). Juice index: an integrated sauvignon blanc grape and wine metabolomics database shows mainly seasonal differences. Metabolomics 15, 1–18. doi: 10.1007/s11306-018-1469-y
Qiao, Z., Hu, H., Shi, S., Yuan, X., Yan, B., Chen, L. (2021). An update on the function, biosynthesis and regulation of floral volatile terpenoids. Horticulturae 7, 451. doi: 10.3390/horticulturae7110451
Qiu, X., Xu, Y., Xiong, B., Dai, L., Huang, S., Dong, T., et al. (2020). Effects of exogenous methyl jasmonate on the synthesis of endogenous jasmonates and the regulation of photosynthesis in citrus. Physiol. plantarum 170, 398–414. doi: 10.1111/ppl.13170
Richmond, R., Bowyer, M., Vuong, Q. (2019). Australian Native fruits: potential uses as functional food ingredients. J. Funct. Foods 62, 103547. doi: 10.1016/j.jff.2019.103547
Rinaldi, A., Moine, V., Moio, L. (2020). Astringency subqualities and sensory perception of Tuscan sangiovese wines. Oeno One 54, 75–85. doi: 10.20870/oeno-one.2020.54.1.2523
Ruiz, J., Kiene, F., Belda, I., Fracassetti, D., Marquina, D., Navascués, E., et al. (2019). Effects on varietal aromas during wine making: a review of the impact of varietal aromas on the flavor of wine. Appl. Microbiol. Biotechnol. 103, 7425–7450. doi: 10.1007/s00253-019-10008-9
Vergara, A. E., Díaz, K., Carvajal, R., Espinoza, L., Alcalde, J. A., Pérez-Donoso, A. G. (2018). Exogenous applications of brassinosteroids improve color of red table grape (Vitis vinifera l. Cv.”Redglobe”) berries. Front. Plant science 9. doi: 10.3389/fpls.2018.00363
Wan, H., Zhang, X., Wang, P., Qiu, H., Guo, Y., Cheng, Y., et al. (2021). Integrated multi-omics analysis of developing ‘Newhall’orange and its glossy mutant provide insights into citrus fragrance formation. Hortic. Plant J. 8, 435–449. doi: 10.1016/j.hpj.2021.12.002
Wang, J., VanderWeide, J., Yan, Y., Tindjau, R., Pico, J., Deluc, L., et al. (2022). Impact of hormone applications on ripening-related metabolites in gewürztraminer grapes (Vitis vinifera l.): the key role of jasmonates in terpene modulation. Food Chem. 388, 132948. doi: 10.1016/j.foodchem.2022.132948
Wu, Y., Zhang, W., Song, S., Xu, W., Zhang, C., Ma, C., et al. (2020). Evolution of volatile compounds during the development of Muscat grape ‘Shine Muscat’(Vitis labrusca× v. vinifera). Food Chem. 309, 125778. doi: 10.1016/j.foodchem.2019.125778
Wu, Y., Zhang, W., Yu, W., Zhao, L., Song, S., Xu, W., et al. (2019). Study on the volatile composition of table grapes of three aroma types. Lwt 115, 108450. doi: 10.1016/j.lwt.2019.108450
Xi, Z. M., Zhang, Z. W., Huo, S. S., Luan, L. Y., Gao, X., Ma, L. N., et al. (2013). Regulating the secondary metabolism in grape berry using exogenous 24-epibrassinolide for enhanced phenolics content and antioxidant capacity. Food Chem. 141, 3056–3065. doi: 10.1016/j.foodchem.2013.05.137
Xu, F., Xi, Z. M., Zhang, H., Zhang, C. J., Zhang, Z. W. (2015). Brassinosteroids are involved in controlling sugar unloading in vitis vinifera ‘Cabernet sauvignon’berries during véraison. Plant Physiol. Biochem. 94, 197–208. doi: 10.1016/j.plaphy.2015.06.005
Xu, H., Zhang, G., Yan, A., Sun, L. (2014). Table grape breeding at the Beijing institute of forestry and pomology. Acta Hortic. 1082, 43–46. doi: 10.17660/ActaHortic.2015.1082.3
Yan, H., Zheng, G., Qu, J., Liu, Y., Huang, X., Zhang, E., et al. (2019). Identification of key candidate genes and pathways in multiple myeloma by integrated bioinformatics analysis. J. Cell. Physiol. 234, 23785–23797. doi: 10.1002/jcp.28947
Yang, L., Yan, Y., Zhao, B., Xu, H., Su, X., Dong, C. (2022). Study on the regulation of exogenous hormones on the absorption of elements and the accumulation of secondary metabolites in the medicinal plant artemisia argyi leaves. Metabolites 12, 984. doi: 10.3390/metabo12100984
Yang, X., Zhu, S., Li, L., Zhang, L., Xian, S., Wang, Y., et al. (2018). Identification of differentially expressed genes and signaling pathways in ovarian cancer by integrated bioinformatics analysis. OncoTargets and Therapy, 1457–1474. doi: 10.2147/OTT.S152238
Yu, J., Zhu, M., Wang, M., Xu, Y., Chen, W., Yang, G. (2020). Transcriptome analysis of calcium-induced accumulation of anthocyanins in grape skin. Scientia Hortic. 260, 108871. doi: 10.1016/j.scienta.2019.108871
Yue, X., Ju, Y., Zhang, H., Wang, Z., Xu, H., Zhang, Z. (2022). Integrated transcriptomic and metabolomic analysis reveals the changes in monoterpene compounds during the development of Muscat Hamburg (Vitis vinifera l.) grape berries. Food Res. Int. 162, 112065. doi: 10.1016/j.foodres.2022.112065
Yue, X., Shi, P., Tang, Y., Zhang, H., Ma, X., Ju, Y., et al. (2021). Effects of methyl jasmonate on the monoterpenes of Muscat Hamburg grapes and wine. J. Sci. Food Agric. 101, 3665–3675. doi: 10.1002/jsfa.10996
Zhang, Y., Yan, H., Li, Y., Xiong, Y., Niu, M., Zhang, X., et al. (2021). Molecular cloning and functional analysis of 1-deoxy-D-xylulose 5-phosphate reductoisomerase from santalum album. Genes 12, 626. doi: 10.3390/genes12050626
Zheng, T., Dong, T., Haider, M. S., Jin, H., Jia, H., Fang, J. (2020). Brassinosteroid regulates 3-hydroxy-3-methylglutaryl CoA reductase to promote grape fruit development. J. Agric. Food Chem. 68, 11987–11996. doi: 10.1021/acs.jafc.0c04466
Zheng, T., Guan, L., Yu, K., Haider, M. S., Nasim, M., Liu, Z., et al. (2021). Expressional diversity of grapevine 3-Hydroxy-3-methylglutaryl-CoA reductase (VvHMGR) in different grapes genotypes. BMC Plant Biol. 21, 1–13. doi: 10.1186/s12870-021-03073-8
Keywords: grapevine, EBR, JA, HS-SPME/GC−MS, aroma, ‘Ruidu Hongyu’, transcriptome sequencing
Citation: Li J, Quan Y, Wu Z, Han J, Zhang Y, Javed HU, Ma C, Jiu S, Zhang C, Wang L and Wang S (2023) EBR and JA regulate aroma substance biosynthesis in ‘Ruidu Hongyu’ grapevine berries by transcriptome and metabolite combined analysis. Front. Plant Sci. 14:1185049. doi: 10.3389/fpls.2023.1185049
Received: 13 March 2023; Accepted: 23 May 2023;
Published: 06 June 2023.
Edited by:
Xin Li, Chinese Academy of Agricultural Sciences, ChinaReviewed by:
Yanlun Ju, Northwest A&F University, ChinaShahid Iqbal, University of Florida, United States
Copyright © 2023 Li, Quan, Wu, Han, Zhang, Javed, Ma, Jiu, Zhang, Wang and Wang. This is an open-access article distributed under the terms of the Creative Commons Attribution License (CC BY). The use, distribution or reproduction in other forums is permitted, provided the original author(s) and the copyright owner(s) are credited and that the original publication in this journal is cited, in accordance with accepted academic practice. No use, distribution or reproduction is permitted which does not comply with these terms.
*Correspondence: Hafiz Umer Javed, qaziumerjaved@cau.edu.cn; Lei Wang, leiwang2016@sjtu.edu.cn