- 1Department of Soil and Crop Sciences, Colorado State University, Fort Collins, CO, United States
- 2Sustainable Soils and Crops, Rothamsted Research, Harpenden, Hertfordshire, United Kingdom
Introduction: Polyphenol oxidases (PPO) are dual activity metalloenzymes that catalyse the production of quinones. In plants, PPO activity may contribute to biotic stress resistance and secondary metabolism but is undesirable for food producers because it causes the discolouration and changes in flavour profiles of products during post-harvest processing. In wheat (Triticum aestivum L.), PPO released from the aleurone layer of the grain during milling results in the discolouration of flour, dough, and end-use products, reducing their value. Loss-of-function mutations in the PPO1 and PPO2 paralogous genes on homoeologous group 2 chromosomes confer reduced PPO activity in the wheat grain. However, limited natural variation and the proximity of these genes complicates the selection of extremely low-PPO wheat varieties by recombination. The goal of the current study was to edit all copies of PPO1 and PPO2 to drive extreme reductions in PPO grain activity in elite wheat varieties.
Results: A CRISPR/Cas9 construct with one single guide RNA (sgRNA) targeting a conserved copper binding domain was used to edit all seven PPO1 and PPO2 genes in the spring wheat cultivar ‘Fielder’. Five of the seven edited T1 lines exhibited significant reductions in PPO activity, and T2 lines had PPO activity up to 86.7% lower than wild-type. The same construct was transformed into the elite winter wheat cultivars ‘Guardian’ and ‘Steamboat’, which have five PPO1 and PPO2 genes. In these varieties PPO activity was reduced by >90% in both T1 and T2 lines. In all three varieties, dough samples from edited lines exhibited reduced browning.
Discussion: This study demonstrates that multi-target editing at late stages of variety development could complement selection for beneficial alleles in crop breeding programs by inducing novel variation in loci inaccessible to recombination.
Introduction
Polyphenol oxidases (PPO) are di-copper metalloenzymes found in all land plants except the Arabidopsis genus (Tran et al., 2012). PPOs are dual activity enzymes, catalysing the hydroxylation of monophenols to diphenols (tyrosinase activity, Enzyme Commission (EC) 1.14.18.1) and the oxidation of o-diphenols to o-quinones (catechol oxidase activity, EC 1.10.3.1) (van Gelder et al., 1997). Quinones react non-enzymatically with cellular thiol and amine groups to produce melanin pigments, causing browning and discolouration of plant tissues. The active site in the PPO proteins for these reactions includes two highly conserved copper binding domains (CuA and CuB) each with three histidine residues that coordinate interactions between phenols and molecular oxygen (Demeke and Morris, 2002). While their physiological function remains unclear, there is indirect evidence that PPO contributes to biotic stress resistance. Many PPO proteins are localized in the chloroplast and come into contact with their phenolic substrates only following senescence, wounding, or physical disruption. In several plant species, PPO genes are upregulated in response to wounding or pathogen infection, and variation in PPO activity is associated with resistance to bacterial and fungal pathogens (Zhang and Sun, 2021). PPO may also play a role in plant secondary metabolism (Araji et al., 2014; Sullivan, 2014).
For the food industry, PPO activity is generally undesirable because it causes the discolouration of plant tissues and changes in flavour profile during post-harvest processing. A readily observed example is the browning of fresh fruit and vegetables following cutting. In common wheat (Triticum aestivum L.) PPO enzymes released from the aleurone layer of the grain during milling catalyse biochemical reactions that result in the time-dependent darkening and discolouration of flour, dough, and end-use products such as noodles, an undesirable trait for consumers (Taranto et al., 2017). Although this can be mitigated by reducing the flour extraction rate during milling or by using food additives, a more cost-effective approach is to breed wheat varieties with low PPO activity in their grains.
PPO activity in the grain is an amenable trait for wheat breeders, with a broad sense heritability of 0.97 (Baik et al., 1994; Liu et al., 2020). Genetic linkage and association studies consistently find that homoeologous loci on group 2 chromosomes are the most important sources of genetic variation for PPO grain activity (Beecher et al., 2012; Liu et al., 2020; Zhai et al., 2020). Underlying these loci are paralogous PPO1 and PPO2 genes that encode PPO enzymes. The genome of the wheat landrace ‘Chinese Spring’ contains a total of 20 PPO genes, among which the PPO1 and PPO2 genes are notable for their high expression levels during grain development (Liu et al., 2020). Both PPO1 and PPO2 have single homoeologous copies on chromosomes 2A and 2D, but the number of PPO1 and PPO2 genes on chromosome 2B ranges from one to three in different wheat varieties (Table S1). In ‘Chinese Spring’, PPO1 is duplicated on chromosome 2B (PPO1-B1 and PPO1-B2), giving seven PPO1 and PPO2 genes in total at these loci (Liu et al., 2020). On each chromosome, these genes are separated by short physical distances (Figure 1).
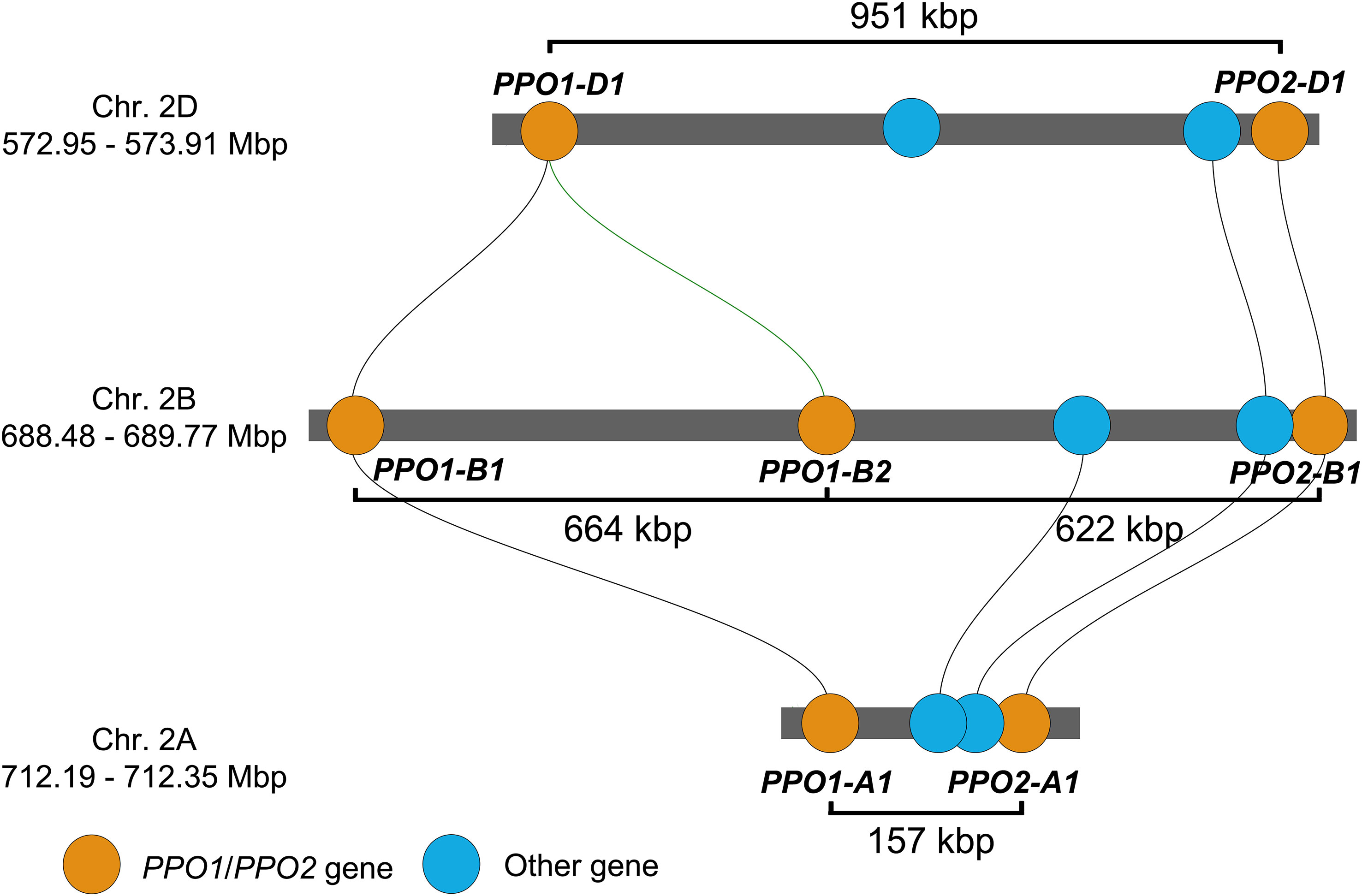
Figure 1 Position of PPO1 and PPO2 genes on homoeologous group 2 chromosomes of the wheat landrace ‘Chinese Spring’ IWGSC RefSeq v1.1 genome assembly. Gene positions are drawn to scale and homologous genes linked by lines determined using the Triticeae Gene Tribe microhomology tool (Chen et al., 2020). Physical distances between the start of each gene are labelled. PPO genes are named according to guidelines endorsed by the Wheat Initiative (Boden et al., 2023).
Breeding programs can use marker assisted selection to introgress null PPO1 and PPO2 alleles to help develop low-PPO varieties. For example, the ppo-A1i and ppo-D1c alleles are non-functional and confer reduced PPO activity in the wheat grain (He et al., 2007; Hystad et al., 2015). However, to date no natural null alleles have been described for PPO2-A1, PPO2-D1 or for any of the PPO1 or PPO2 genes on chromosome 2B that also contribute to PPO activity (Beecher et al., 2012; Taranto, 2015; Liu et al., 2020). In addition to the close physical distances between genes at these loci, this limited natural variation complicates the recombination of non-functional natural variants for each PPO1 and PPO2 gene using marker assisted selection.
The genome editing tool CRISPR/Cas9 is now routinely used to induce novel variation at specific genetic loci in crop genomes (Gao, 2021). This technology is particularly useful when multiple simultaneous gene knockouts are required. Recently, CRISPR/Cas9 was used to edit three PPO1 homoeologues in the spring wheat variety ‘Fielder’ resulting in significant reductions in PPO activity in the T2 and T3 generations of edited plants (Zhang et al., 2021). The goal of the current study was to edit all seven PPO1 and PPO2 genes in different elite wheat cultivars to drive extreme reductions in grain PPO activity. Long-read genome assemblies were used to inform the design of a CRISPR/Cas9 construct with one sgRNA targeting a region conserved in all PPO1 and PPO2 genes. ‘Fielder’ plants transformed with this construct exhibited significant reductions in PPO grain activity in the T1 and T2 generations. The same construct was used to edit two elite winter wheat varieties, resulting in a reduction in PPO activity of more than 90%, including several individuals with undetectable PPO activity. This study demonstrates that carefully designed CRISPR/Cas9 constructs can be used to edit multi-gene families in polyploid crop species and that direct editing of beneficial alleles during the late stages of elite variety development could complement traditional breeding methods for crop improvement.
Results
PPO1 and PPO2 genes are highly expressed in developing wheat grains
An analysis of a developmental RNA-seq dataset from the wheat landrace ‘Chinese Spring’ showed that among the 20 PPO genes, the seven paralogous PPO1 and PPO2 genes on group 2 chromosomes are predominantly expressed in developing grain tissues (Figure 2). Some PPO1 and PPO2 genes were also expressed in other plant tissues; PPO1-D1 was highly expressed in stem and spike tissues during anthesis while PPO1-B1 transcripts were detected in leaf tissues post-anthesis (Figure 2). By contrast, transcript levels of other members of the PPO family were low in the developing grain and were more highly expressed in vegetative tissues (Figure 2). These results are consistent with previous studies (Liu et al., 2020), demonstrating that wheat PPO genes are developmentally regulated and that PPO1 and PPO2 genes contribute the majority of PPO transcripts in the grain.
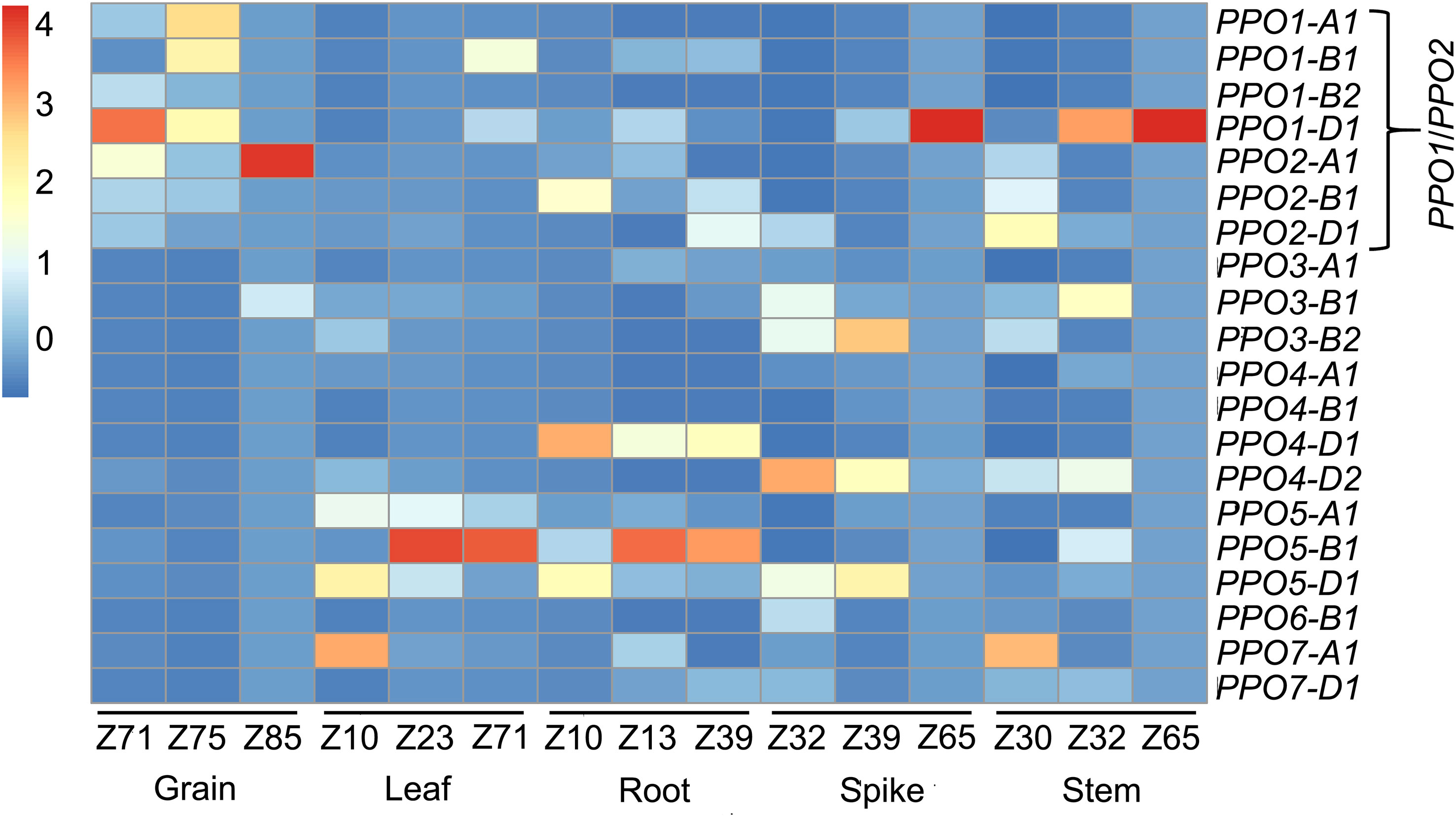
Figure 2 Expression profiles of 20 wheat PPO genes in different wheat tissues. RNA-seq reads from a hexaploid wheat developmental timecourse (Choulet et al., 2014) were mapped to the IWGSC RefSeq v1.1 wheat reference genome. The developmental stage in each tissue is presented in the Zadoks scale (Zadoks et al., 1974). Expression in transcript per million (TPM) values are scaled for each timepoint using the scale(Data_num) function in the R package pheatmap.
Multi-target PPO1 and PPO2 editing confers significant reductions in PPO grain activity
Based on their expression profile and known association with PPO activity in the grain, all seven PPO1 and PPO2 genes were targeted for knockout by genome editing in the spring wheat variety ‘Fielder’. The ‘Fielder’ genome contains 25 PPO genes (defined by the presence of tyrosinase, DWL and KWDV domains in their encoded proteins), including all 20 PPO genes described in ‘Chinese Spring’ and expansions in PPO gene number on chromosomes 3A and 6B (Table S2). Note that PPO genes have been named based on their phylogenetic relationships in accordance with guidelines endorsed by the Wheat Initiative (Boden et al., 2023) and do not necessarily match earlier publications. Of the seven PPO1 and PPO2 genes in ‘Fielder’, three are predicted to encode non-functional proteins, including a PPO1-A1 allele with a 54-nucleotide deletion in exon 3 not previously described (Table S3).
Alignment of all 25 PPO genes revealed a 38-nucleotide region within the CuB binding domain that shared 100% identity in all seven PPO1 and PPO2 target genes, which was used to design a CRISPR/Cas9 construct to edit all seven genes (See Experimental procedures).
All seven T0 plants regenerated from embryos transformed with the CRISPR/Cas9 construct exhibited different types of induced variation in PPO1 and PPO2 genes 3-4 nucleotides upstream of the PAM, consistent with the typical cleavage sites of Cas9-induced double stranded breaks. Of the seven derived T1 lines, five exhibited significant reductions in PPO activity compared to wild-type ‘Fielder’ (P < 0.01), ranging from a 45.1% reduction in line 81.5a to an 80.7% reduction in line 81.12a (Figure 3A, Table S6). Grain PPO activity in lines 81.8b and 81.16a were not significantly different from wild-type ‘Fielder’ (P >0.05), with the latter line exhibiting higher mean PPO activity than in wild-type ‘Fielder’ (Figure 3A, Table S6).
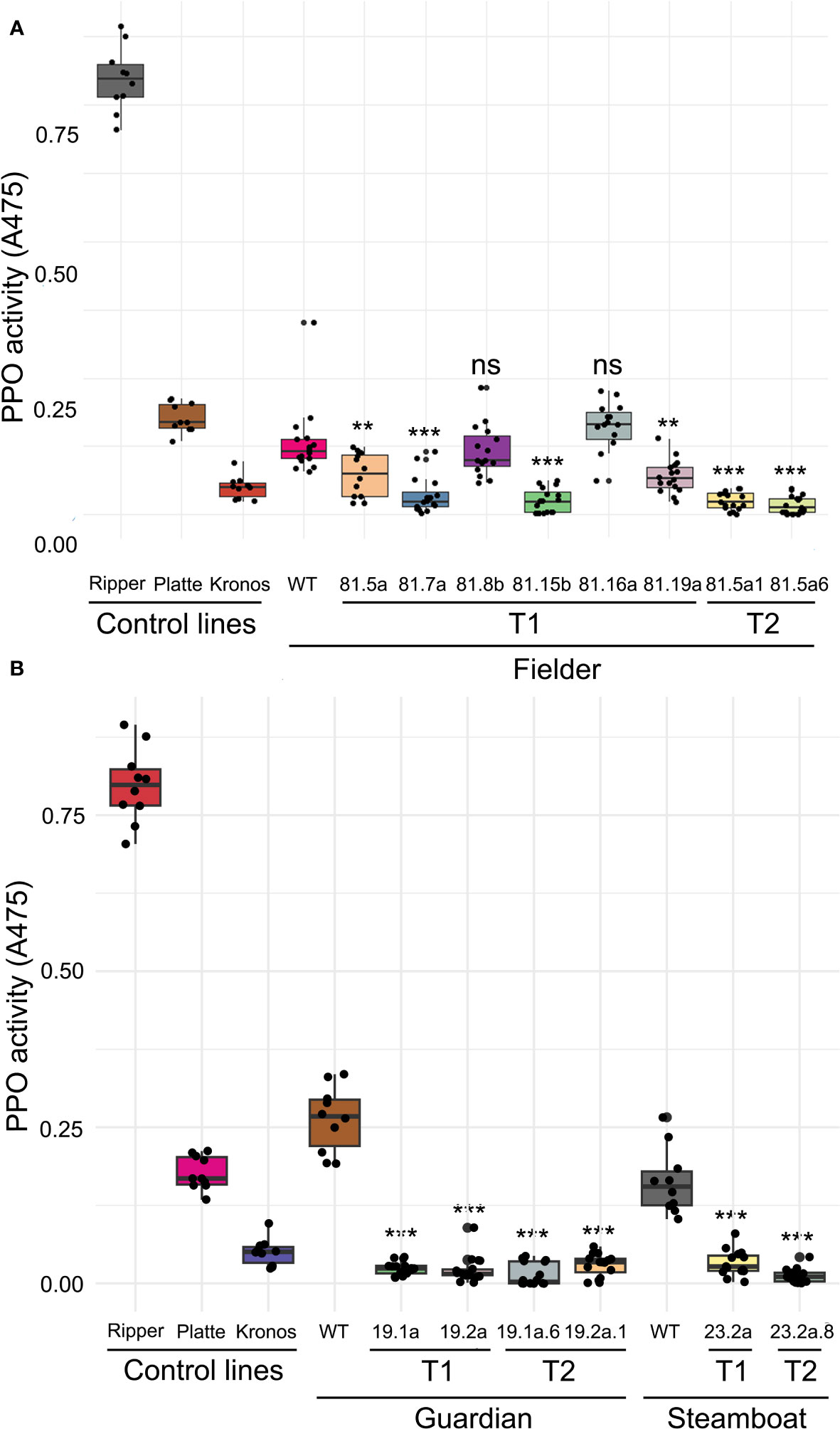
Figure 3 PPO activity in wild-type and edited wheat lines. Mean PPO activity from wild-type, T1 and T2 lines in (A) the spring variety ‘Fielder’ (n = 12 to 18) and (B) the winter varieties ‘Steamboat’ and ‘Guardian’. ‘Ripper’ (high-PPO common wheat), ‘Platte’ (low-PPO common wheat) and ‘Kronos’ (low-PPO durum wheat) were included as control lines (n = 10). **P < 0.01. ***P < 0.001. ns, not significant.
The variation in PPO activity both between and within T1 lines suggests a complex segregation pattern of edited alleles for each target gene. This was reflected in genotypic data from Sanger sequencing of each PPO1 and PPO2 gene. Two different T1 individuals from line 81.5a exhibited examples of different edited alleles of the same target gene (PPO1-D1), biallelic edits (PPO1-A1), and the absence of edits in some target genes (PPO1-D2) (Table S7). Two individuals that exhibited the lowest PPO activity in T1 line 81.5a were selfed to generate T2 lines. Genotyping of selected T2 individuals revealed that they carry a greater number of fixed, non-functional induced alleles than T1 plants (Table S7). Mean PPO activity in these T2 lines was 80.9% and 86.7% lower than in wild-type ‘Fielder’ (P < 0.001) which was greater than the reduction in the corresponding T1 lines and with a lower standard deviation (Figure 3A, Table S6).
Genome editing reduces PPO activity in two elite winter wheat cultivars
The sequence of the sgRNA-PAM is 100% identical in all PPO1 and PPO2 genes in fifteen wheat varieties with assembled genomes (Walkowiak et al., 2020), suggesting this construct can be used to edit PPO1 and PPO2 genes in diverse wheat germplasm. To test this, the editing construct was transformed into the elite winter wheat cultivars ‘Guardian’ and ‘Steamboat’. No genome assembly is available for these cultivars, so PCR amplification was used to confirm the presence of each PPO1 and PPO2 gene. Homoeolog-specific PCR assays for PPO1-B1 and PPO1-B2 consistently failed to generate an amplicon in either ‘Guardian’ and ‘Steamboat’, suggesting the absence of these genes in these varieties. PPO1-B1 and PPO1-B2 were also absent from eight other common wheat genomes (Table S1) likely because the progenitors of these lines did not carry the duplication event that originated these genes (Figure S1).
Two independent T0 ‘Guardian’ plants and one T0 ‘Steamboat’ plant exhibited edits in all five target PPO1 and PPO2 genes, determined by Sanger sequencing. Derived T1 populations from each of these plants all exhibited significant reductions in PPO activity (P < 0.001) ranging from an 80.2% reduction in ‘Steamboat’ line 23.2b to a 91.6% reduction in ‘Guardian’ line 19.2a compared to their respective wild-type controls (Figure 3B, Table S6). The reduction in grain PPO activity was even greater in T2 lines derived from T1 plants exhibiting the lowest PPO activity, including a 96.0% reduction compared to wild-type in ‘Guardian’ line 19.2a.6 and a 92.4% reduction in ‘Steamboat’ line 23.2a.8 (Figure 3B, Table S6). Genotyping of selected individuals confirmed that both T1 and T2 plants carried induced edits in each target gene (Tables S8, S9). Mean PPO activity in these T1 and T2 lines is lower than in the durum wheat ‘Kronos’ (Figure 3B), a genotype that is commonly included as an extremely low-PPO control line and in which only PPO1-A1 and PPO2-A1 encode functional PPO enzymes (Table S10). Furthermore, multiple individuals within these T2 populations exhibited undetectable PPO activity (Table S6), demonstrating that by editing PPO1 and PPO2 genes, it is possible to eliminate grain PPO activity in elite wheat varieties.
Low PPO grain activity confers reduced dough browning
To determine the association between PPO grain activity and browning, the color of dough samples produced from whole-grain flour was evaluated across a 24-hour time course. Dough samples produced from the high-PPO control line ‘Ripper’ exhibited stronger browning than other genotypes, illustrated by low quantitative brightness values across the time course (Figure 4A, Table S11) and by visual assessment at 0 h and 24 h time points (Figure 4D). Dough browning was less pronounced in wild-type ‘Fielder’, consistent with the lower levels of grain PPO activity in this variety (Figure 4A). The ‘Fielder’ T2 edited lines 81.5a.1 and 81.5a.6, which exhibit the greatest reductions in PPO grain activity (Figure 3A), exhibited significantly (P < 0.01) higher brightness values at every time point compared to the wild-type (Figure 4A, Table S11) and at the 24h time point, dough samples were noticeably lighter in edited lines (Figure 4D). Similarly, T2 edited lines in ‘Steamboat’ (Figure 4B) and ‘Guardian’ (Figure 4C) backgrounds exhibited significantly (P < 0.01) higher brightness values at 24 h (Table S11) and less pronounced dough browning compared to their respective wild-type (Figure 4D).
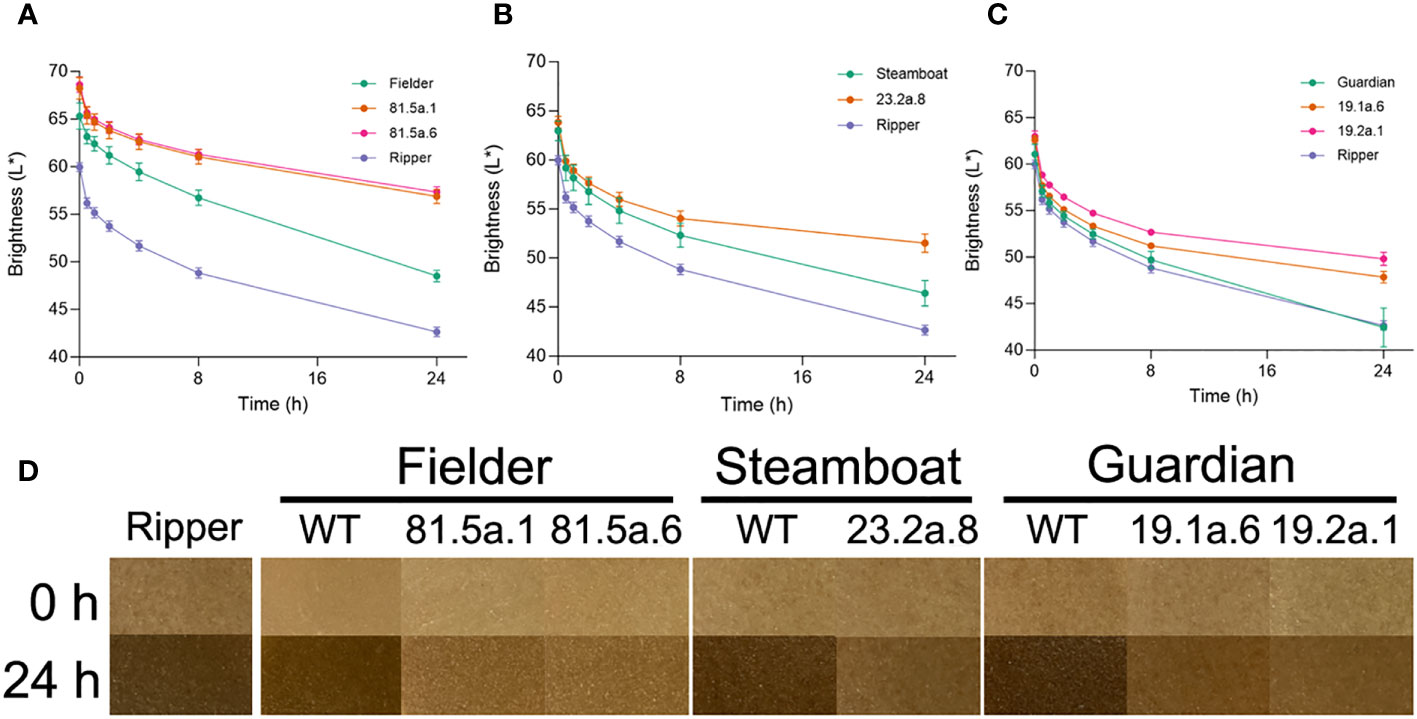
Figure 4 Reduced PPO activity is associated with lower dough browning. Brightness (L*) values of dough samples produced from grains of different genotypes in (A) Fielder, (B) Steamboat and (C) Guardian. Wild-type ‘Ripper’ was included as a high-PPO control. (D) Photos of representative dough samples at 0 h and 24 h timepoints from different genotypes.
Discussion
Multi-target genome editing in polyploid wheat
One application of the genome editing tool CRISPR/Cas9 is to simultaneously induce novel genetic variation at multiple loci, including those in the same linkage block. This is especially powerful when targeting multi-gene families such as PPO that are subject to a high rate of gene expansion (Tran et al., 2012). Another recent example is the use of CRISPR/Cas9 to edit multiple ω- and γ-gliadin genes arranged in tandemly duplicated gene clusters (Yu et al., 2023). A growing set of wheat genomes assembled using long-read sequencing data (Walkowiak et al., 2020) facilitates the characterization of this variation and ensures the appropriate design of CRISPR/Cas9 constructs for each target variety. The goal of the current study was to edit all PPO1 and PPO2 genes in different elite wheat varieties to reduce PPO activity in the grain. The ‘Fielder’ genome (Sato et al., 2021) was used to design a sgRNA targeting a region of the highly conserved CuB binding domain that is 100% identical between all seven target PPO1 and PPO2 genes (Figure 5). In addition to facilitating multi-target editing, designing protospacers in a conserved domain increases the likelihood that in-frame deletions or insertions will disrupt gene function. For example, the 15-bp deletion in PPO1-B2 in ‘Fielder’ (Table S7) eliminates the highly-conserved His and Phe amino acid residues that likely play a critical role in PPO enzyme function (Tran et al., 2012). This contrasts with an earlier CRISPR/Cas9 study to edit PPO1 genes that used a sgRNA targeting a genomic region between the conserved CuA and CuB binding domains (Zhang et al., 2021). There are polymorphisms between this protospacer sequence and four of the seven PPO1/PPO2 genes from ‘Fielder’, including four mismatches with PPO2-A1, PPO2-B1, and PPO2-D1 (Table S12). These genes are expressed during grain development (Figure 2) and likely contribute to PPO activity in this tissue (Beecher et al., 2012; Taranto, 2015; Liu et al., 2020) suggesting that null alleles in all PPO1 and PPO2 genes will be required to maximize reductions in PPO activity by editing.
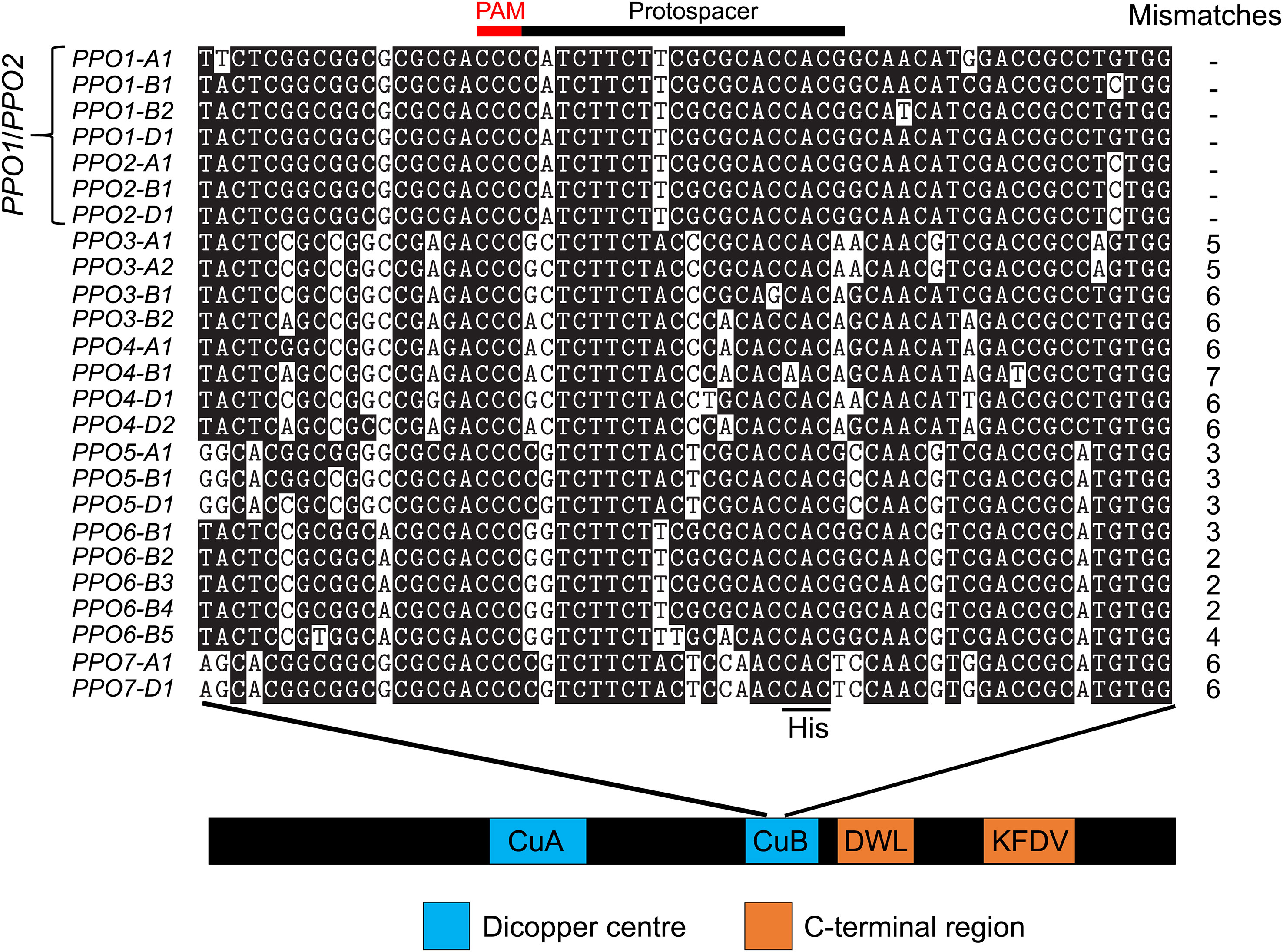
Figure 5 A sgRNA designed to target seven PPO1 and PPO2 genes in wheat. The 20-nucleotide protospacer sequence and three nucleotide protospacer adjacent motif (PAM) are indicated. The targeted region encodes the conserved copper binding site II (CuB) domain required for PPO protein function. The codon encoding the third conserved histidine residue (CAC or CAT) is highlighted within the CuB binding domain. Sequence alignments are displayed in 5’-3’ orientation to show the position of the sgRNA, which is designed to the antisense strand. The displayed region is from nucleotides 1,442 to 1,501 based on the distance from the ATG start of the genomic DNA of PPO1-A1 in ‘Fielder’. The protospacer position is 1,462-1,481 on the reverse strand and is preceded by a ‘GGG’ PAM site. Number of mismatches between the protospacer and each target gene is shown to the right of the alignment.
In the current study, wheat plants with extremely low PPO activity in their grains were developed for all three target varieties that carried disruptive mutations in all PPO1 and PPO2 genes, including three individual T2 plants with undetectable PPO activity. These observations suggest that despite the presence of PPO4-D2 transcripts in the developing grain (Figure 2) and genetic studies that identified QTL associated with PPO activity overlapping with PPO3A-1 and PPO7D-1 (Liu et al., 2020; Zhai et al., 2020), other PPO genes do not make a major contribution to PPO activity in the wheat grain. It is interesting to note that while dough brightness was significantly higher in edited lines compared to the wild-type in all varieties, darkening occurred in all samples (Figure 4). This might be accounted for by the presence of residual PPO activity in edited samples, or the involvement of additional factors driving dough browning which will require further investigation (Bhattacharya et al., 1999).
CRISPR/Cas9 design in wheat
It is important to note that while a high rate of editing was achieved using the sgRNA described in the main text, two other sgRNAs targeting a region approximately 150 nucleotides upstream that is also conserved in all seven PPO1 and PPO2 genes exhibited zero editing efficiency in 15 T0 plants screened for edits (Table S4). It is possible that these CRISPR/Cas9 constructs may have induced transgenerational editing in the T1 generation, as observed in previous studies (Wang et al., 2018; Zhang et al., 2021), but this was not evaluated due to the high rate of editing observed with the selected sgRNA. These results strongly suggest major differences in editing efficiencies for sgRNAs targeting DNA sequences in close proximity and that the protospacer sequence composition is critically important for editing efficiency. This is despite the sgRNAs exhibiting comparable “Rule Set 2” (RS2) scores (Table S4), a metric predicting on-target editing efficiency used in CRISPR design tools (Liu et al., 2017a; Cram et al., 2019). This score is derived from models built on empirical editing data from hundreds of constructs used in animal studies (Doench et al., 2016). It is possible that these models do not capture factors that influence editing efficiency in plant species. As the use of CRISPR/Cas9 across different plant species becomes increasingly common, it would be valuable for the plant research community to coordinate editing datasets to develop genus- or species-specific models that can more accurately predict editing efficiency. Highly predictive models would be especially useful when designing editing strategies with a limited number of potential protospacer sequences, such as in multi-target editing or when a highly specific target edit is required.
Physiological role of PPO in wheat
Despite indirect evidence from some species, the physiological role of PPOs in the plant kingdom remains unclear. Unusually for oxidative enzymes, the size of the PPO gene family is highly variable between species and might be driven by clade-specific responses to diverse environmental stresses (Tran et al., 2012). The absence of PPO genes from the Arabidopsis genome shows they are not essential and are unlikely to play a role in primary metabolism, but might instead be involved in either environmental responses or secondary metabolism (Tran et al., 2012). In cereals, it has been suggested that PPO activity in grain tissues may contribute to the biochemical resilience to decay in the dormant seed (Fuerst et al., 2014) and in reducing the incidence of black-point, a condition that reduces the quality and aesthetics of wheat products (Liu et al., 2017b). The edited lines exhibiting extremely low PPO activity are ideal near-isogenic materials to test these hypotheses and to characterize the role of PPO1 and PPO2 genes in field conditions. In addition, lines carrying different combinations of PPO1 and PPO2 null alleles would help determine the extent to which genes in this family exhibit functional redundancy.
Applications in breeding
The protospacer sequence used in the current study is 100% conserved in all PPO1 and PPO2 genes from 17 wheat genome assemblies screened and conferred significant reductions in PPO activity in all three varieties tested (Figure 3), suggesting this approach can be applied in diverse wheat germplasm. This protospacer sequence is also 100% conserved in orthologous PPO1 and PPO2 genes from barley (Hordeum vulgare) (Taketa et al., 2010) and rye (Secale cereale) (Table S13), so could likely be applied in these species by cloning the sgRNA into an appropriate transformation construct. However, the orthologous PPO1 and PPO2 genes from rice (Oryza sativa) (Yu et al., 2008), maize (Zea mays), and millet (Sorghum bicolor) all contained multiple polymorphisms in the protospacer sequence (Table S13). The high conservation of the CuB binding domain in PPO proteins make this region an excellent target for multi-target gene editing in other species, including to generate non-transgenic low-PPO varieties of crops such as mushrooms, potatoes and apples for which RNAi and amiRNA have previously been applied to reduce PPO activity (Murata et al., 2001; Chi et al., 2014; Waltz, 2016; González et al., 2019). This approach may also find application in pea (Pisum sativum) and faba bean (Vicia faba L.) breeding, where natural null PPO alleles conferring a pale hilum colour have been selected in cultivated varieties for their preference by consumers (Balarynová et al., 2022; Jayakodi et al., 2023).
Advances in genotype-independent transformation technologies facilitates genome editing directly in elite wheat cultivars (Debernardi et al., 2020; Wang et al., 2022). Editing PPO1 and PPO2 genes in the late stages of variety development would eliminate the need to select for PPO activity in earlier generations, saving breeders time and resources, expand access to high-PPO wheat germplasm, and maximise profits for growers by ensuring high flour yield and quality for all markets. This trait is likely to be especially desirable for applications using whole white wheat flour which retains a higher proportion of aleurone tissue that is removed during white flour refining. It will be necessary to comprehensively phenotype low-PPO edited wheat plants in the field for any undesirable pleiotropic phenotypes, including biotic stress resistance or secondary metabolism. Crosses have been initiated between edited and wild-type plants to generate individuals segregating for the transgene insertion to select edited, non-transgenic lines to phenotype these materials in replicated field trials.
As the application of different CRISPR-derived tools becomes more efficient in different crops and as laws and regulations in some key markets show some signs of loosening (for example, the recent passage of the Genetic Technology (Precision Breeding) Act 2023 through the UK parliament), the question of which alleles to edit becomes more urgent. Evolutionary selection favours mutations in genes with low pleiotropy, that are expressed in a small number of tissues, and which are predicted to be associated with few biological processes (Stern and Orgogozo, 2008). Reported applications of CRISPR/Cas9 in wheat including the PPO1 and PPO2 genes described here, as well as TaASN2 (Raffan et al., 2023) and glutenin genes (Yu et al., 2023) match this profile. An underexplored source of adaptive mutations are gain-of-function alleles that affect transcriptional regulation (Martin and Orgogozo, 2013) as demonstrated previously (Rodríguez-Leal et al., 2017; Song et al., 2022). Identifying beneficial, non-pleiotropic allelic variants that can be directly edited into elite varieties will be essential to fully exploit the power of genome editing for crop improvement.
Conclusions
Seven PPO genes were edited using one sgRNA in hexaploid wheat to generate plants with extremely low grain PPO activity and reduced dough browning. Directly editing these genes in the late stages of elite variety development may be a complementary approach to accelerate crop improvement, reducing the burden of selecting for multiple loci during early stages of selection. Before these alleles can be deployed in breeding programs, it will be important to assess the performance of low-PPO edited wheat lines in replicated field experiments to understand the impacts on wheat physiology and performance.
Experimental procedures
Plant materials and growth conditions
The common wheat (Triticum aestivum L.) varieties ‘Fielder’, ‘Guardian’, ‘Steamboat’, ‘Ripper’ and ‘Platte’, and the durum wheat (Triticum turgidum subsp. durum Desf.) variety ‘Kronos’ were used in this study. Seeds of ‘Fielder’ were provided by Dr. David Garvin (USDA-ARS, St. Paul, MN) and seeds of all other varieties were provided by the Colorado State University Wheat Breeding Program. Seeds were germinated in Anchor Paper Co. germination paper for 7 days until emergence, then sown into 1-gallon pots, 2 seedlings per pot, containing water-saturated Promix HP Plus Biofungicide and Mycorrhizae potting mix and Osmocote Plus 15-9-12. Two-week-old seedlings of ‘Guardian’ and ‘Steamboat’ were first transferred to plastic bags and vernalized for 6 weeks at 4°C before being transferred to 1-gallon pots. All plants were grown in greenhouse conditions supplemented by light to maintain a 16 h photoperiod. Temperatures were maintained between 22°C and 25°C during the day and between 18°C and 22°C during the night. Plants were treated with pesticides as required.
PPO sequence analysis
Genomic DNA sequence of the 20 PPO genes previously described (Liu et al., 2020) were extracted from the ‘Chinese Spring’ IWGSC v2.0 reference genome (IWGSC, 2018; Zhu et al., 2021) and used as BLASTn queries to identify PPO genes in the assemblies of the spring wheat variety ‘Fielder’ (Sato et al., 2021) and 14 other common wheat varieties (Walkowiak et al., 2020). All PPO genes, including those absent from 'Chinese Spring' but identified in ‘Fielder’, were named following the guidelines endorsed by the Wheat Initiative (Boden et al., 2023). HMMscan was used to confirm the presence of tyrosinase, DWL and KWDV domains in each encoded protein. Microhomology was determined and visualized using the online Triticiae Gene Tribe tool (Chen et al., 2020). The coding sequences of TaPPO1-A1 and TaPPO2-A1 were used as queries in BLASTn searches to identify orthologous PPO sequences from the genomes of Hordeum vulgare (version: MorexV3_pseudomolecules_assembly), Secale cereale (version: Rye_Lo7_2018_v1p1p1), Oryza sativa japonica (version: IRGSP-1.0), Zea mays (version: Zm-B73-REFERENCE-NAM-5.0), and Sorghum bicolor (version: Sorghum_bicolor_NCBIv3).
Expression levels of all PPO genes were calculated from mapping a developmental timecourse RNA-seq dataset (Choulet et al., 2014) to the IWGSC v1.2 genome assembly as previously described (IWGSC, 2018) (Pearce et al., 2015). Derived transcript per million (TPM) values were displayed as a heatmap using the R package “pheatmap” and scaled for each timepoint using the function “scale(Data_num)”.
CRISPR/Cas9 plasmid assembly and transformation
The CRISPR design tools CRISPR-P (Liu et al., 2017a) and wheatCRISPR (Cram et al., 2019) were used to support sgRNA design, incorporating “Rule Set 2” scores to estimate editing efficiency (Doench et al., 2016) and scanning the wheat genome to identify potential off-target editing effects. The protospacer was selected based on its high RS2 score, 100% identity to all seven target PPO1 and PPO2 genes and low predicted off-target activity in other genes in the wheat genome (Figure 5, Table S4). No off-target effects are predicted in the protein-coding region of any other gene in the wheat genome and while the promoters of eight genes are potentially targeted, each had at least three mismatches with the protospacer sequence (Table S5). A single G nucleotide was added to the start of the 20 nucleotide protospacer sequence, and the 21-nucleotide sequence (GCGTGGTGCGCGAAGAAGATG) was synthesized as overlapping, complementary oligos with overhanging 5’ and 3’ ends complementary to the insertion site of the target vector. The JD633 vector (Debernardi et al., 2020) was digested with AarI and the hybridized oligos were inserted by Golden Gate cloning. This sgRNA was integrated immediately downstream of the U6 promoter. The vector also contains ZmUbi1::SpCas9 and TaGRF4:TaGIF1 coding sequences which confer improved regeneration rates in transformed callus tissue (Debernardi et al., 2020). Ligated vectors were confirmed by Sanger sequencing and transformed into DH5-α Escherichia coli cells from which purified plasmid DNA was extracted. After confirming sequence insertion and integrity by Sanger sequencing, plasmid DNA was transformed into Agrobacterium tumefaciens strain AGL1 by heat shock and transformed into each wheat genotype using embryo transformation as described previously (Hayta et al., 2021).
Genotyping
Leaf tissue was harvested from regenerated plants after they had been moved to wheat rooting and growth media (Hayta et al., 2021) and had developed a minimum of 3 leaves at least 4 cm in length. DNA was extracted using the standard CTAB extraction method (Allen et al., 2006) and normalized to 200 ng/µL. Putative transgenic plants were validated by PCR assays to amplify two fragments of the transformed plasmid using the primers listed in Table S14. To characterize induced edits, homoeolog-specific PCR assays were designed to amplify each of the seven target PPO1 and PPO2 genes (Table S14). Because of variation in the target sequence, assays for PPO1-D1 and PPO2-D1 were customized for different genotypes.
Each PCR consisted of 2.5 µL 10X Standard Taq Reaction Buffer (NEB, Ipswich, MA, USA), 0.5 µL 10 mM dNTPs (Invitrogen, Life Technologies, Carlsbad, CA, USA), 0.5 µL 10 µM Forward Primer, 0.5 µL 10 µM Reverse Primer, 5 µL Template DNA (50 ng/µl), 0.125 µL Taq DNA Polymerase (NEB, Ipswich, MA, USA) and nuclease-free water to a total reaction volume of 25 µL. For some reactions, HotStarTaq DNA polymerase (Qiagen, Hilden, Germany) was used, using the appropriate buffers and heat activation thermocycler steps recommended by the manufacturer. Reactions were run on a thermocycler using the following conditions: 95°C 5 min; 40 cycles of 95°C 30 s, 55-65°C 30 s, 68°C 30 s – 2 min; 72°C 7 min. Annealing temperature and extension time varied by PCR assay and are described with the corresponding primers in Table S14. Selected PCR amplicons were purified using ExoSAP-IT™ PCR Product Cleanup Reagent (Thermo Fisher Scientific, Waltham, MA, USA) and sequenced with Sanger sequencing (Genewiz, Azenta Life Sciences).
Phenotyping
PPO content was assessed using the L-DOPA method (AACC International Method 22-85.01) using mature harvested wheat grains from greenhouse-grown plants. For each genotype, 5 kernels were placed into a 2 mL microcentrifuge tube before adding 1.5 mL of a solution of 5 mM L-DOPA solution in 50 mM MOPS (pH 6.5). The tubes were sealed, then rotated at 10 rpm for two hours to allow oxygen into the reaction. Absorbance of the resulting solution was measured using 1 mL of sample in a spectrophotometer set to measure at 475 nm using L-DOPA solution as a zero sample. Grains from three other wheat varieties harvested from field experiments in the Colorado State University wheat breeding program were included as controls: ‘Kronos’, as an extremely low PPO sample, ‘Ripper’, as a high PPO control, and ‘Platte’, as a low PPO control. Seeds from untransformed wild-type plants of ‘Guardian’, ‘Steamboat’ and ‘Fielder’ were used as comparisons for the corresponding edited lines. The number of replications used for each genotype is listed in Table S6. To determine the significance of differences between lines, pairwise two-tailed Student’s t-tests were applied.
To evaluate the association between PPO grain activity and browning, the color of dough samples produced from whole grain flour were evaluated across a 24-hour time course based on the protocol described by Brütsch et al. (2018). For selected lines, five biological replicates were used, each consisting of grain from three different T3 individual plants. Grains were milled using a Cyclone sample mill (UDY Corporation, Fort Collins, CO, USA) to produce 4 g of whole-grain flour which was mixed with sterile water to 40% wb for three minutes using a Mixograph (National Manufacturing, Lincoln, NE, USA). The dough was pressed between two petri dishes to obtain a uniform, 2 mm thick disc. Dough color was measured at seven timepoints (0 h, 30 min, 1 h, 2 h, 4 h, 8 h and 24 h) using a Minolta CR-300 chromameter (Minolta Camera Co., Ltd., Tokyo, Japan) set to measure the International Commission on Illumination (CIE) L*a*b color space. Between measurements, the dough samples were kept in sterile petri dishes sealed with parafilm and stored at 22°C. Pairwise two-tailed Student’s t-tests were performed to determine differences in brightness (L) values between wild-type and edited lines of each variety. Photographs were taken of representative dough samples at 0 h and 24 h timepoints.
Data availability statement
The datasets presented in this study can be found in online repositories. The names of the repository/repositories and accession number(s) can be found in the article/Supplementary material.
Author contributions
Designed and performed the research: FW, CK, RA, KR, AK, JS. Funding acquisition: RM, SP. Project management: RM, SP. Wrote the first draft of the manuscript SP. All authors contributed to the article and approved the submitted version.
Funding
This project was supported by Competitive Grant 2022-68013-36439 (WheatCAP) from the USDA National Institute of Food and Agriculture and by funding from the Colorado Wheat Administrative Commission and the Colorado Wheat Research Foundation.
Acknowledgments
We are grateful to Dr. Mervin Poole (Heygates Ltd.) and Dr. Scott Haley (Colorado State University) for helpful discussions about PPO activity in wheat breeding and milling. This project was supported by Competitive Grant 2022-68013-36439 (WheatCAP) from the USDA National Institute of Food and Agriculture and by funding from the Colorado Wheat Administrative Commission and the Colorado Wheat Research Foundation.
Conflict of interest
This work is part of a pending U.S. patent application.
The authors declare that the research was conducted in the absence of any commercial or financial relationships that could be construed as a potential conflict of interest.
Publisher’s note
All claims expressed in this article are solely those of the authors and do not necessarily represent those of their affiliated organizations, or those of the publisher, the editors and the reviewers. Any product that may be evaluated in this article, or claim that may be made by its manufacturer, is not guaranteed or endorsed by the publisher.
Supplementary material
The Supplementary Material for this article can be found online at: https://www.frontiersin.org/articles/10.3389/fpls.2023.1247680/full#supplementary-material
References
Allen, G. C., Flores-Vergara, M. A., Krasynanski, S., Kumar, S., Thompson, W. F. (2006). A modified protocol for rapid DNA isolation from plant tissues using cetyltrimethylammonium bromide. Nat. Protoc. 1, 2320–2325. doi: 10.1038/nprot.2006.384
Araji, S., Grammer, T. A., Gertzen, R., Anderson, S. D., Mikulic-Petkovsek, M., Veberic, R., et al. (2014). Novel roles for the polyphenol oxidase enzyme in secondary metabolism and the regulation of cell death in walnut. Plant Physiol. 164, 1191–1203. doi: 10.1104/pp.113.228593
Baik, B. K., Czuchajowska, Z., Pomeranz, Y. (1994). Comparison of polyphenol pxidase activities in wheats and flours from Australian and U.S. cultivars. J. Cereal Sci. 19, 291–296. doi: 10.1006/jcrs.1994.1036
Balarynová, J., Klčová, B., Sekaninová, J., Kobrlová, L., Cechová, M. Z., Krejčí, P., et al. (2022). The loss of polyphenol oxidase function is associated with hilum pigmentation and has been selected during pea domestication. New Phytol. 235, 1807–1821. doi: 10.1111/nph.18256
Beecher, B. S., Carter, A. H., See, D. R. (2012). Genetic mapping of new seed-expressed polyphenol oxidase genes in wheat (Triticum aestivum L.). Theor. Appl. Genet. 124, 1463–1473. doi: 10.1007/s00122-012-1801-2
Bhattacharya, M., Luo, Q., Corke, H. (1999). Time-dependent changes in dough color in hexaploid wheat landraces differing in polyphenol oxidase activity. J. Agric. Food Chem. 47, 3579–3585. doi: 10.1021/jf990041i
Boden, S. A., McIntosh, R. A., Uauy, C., Krattinger, S. G., Dubcovsky, J., Rogers, W. J., et al. (2023). Updated guidelines for gene nomenclature in wheat. Theor. Appl. Genet. 136, 72. doi: 10.1007/s00122-023-04253-w
Brütsch, L., Rugiero, S., Serrano, S. S., Städeli, C., Windhab, E. J., Fischer, P., et al. (2018). Targeted inhibition of enzymatic browning in wheat pastry dough. J. Agric. Food Chem. 66, 12353–12360. doi: 10.1021/acs.jafc.8b04477
Chen, Y., Song, W., Xie, X., Wang, Z., Guan, P., Peng, H., et al. (2020). A collinearity-incorporating homology inference strategy for connecting emerging assemblies in the Triticeae tribe as a pilot practice in the plant pangenomic era. Mol. Plant 13, 1694–1708. doi: 10.1016/j.molp.2020.09.019
Chi, M., Bhagwat, B., Lane, W. D., Tang, G., Su, Y., Sun, R., et al. (2014). Reduced polyphenol oxidase gene expression and enzymatic browning in potato (Solanum tuberosum L.) with artificial microRNAs. BMC Plant Biol. 14, 62. doi: 10.1186/1471-2229-14-62
Choulet, F., Alberti, A., Theil, S., Glover, N., Barbe, V., Daron, J., et al. (2014). Structural and functional partitioning of bread wheat chromosome 3B. Science 345, 1249721. doi: 10.1126/science.1249721
Cram, D., Kulkarni, M., Buchwaldt, M., Rajagopalan, N., Bhowmik, P., Rozwadowski, K., et al. (2019). WheatCRISPR: a web-based guide RNA design tool for CRISPR/Cas9-mediated genome editing in wheat. BMC Plant Biol. 19, 474. doi: 10.1186/s12870-019-2097-z
Debernardi, J. M., Tricoli, D. M., Ercoli, M. F., Hayta, S., Ronald, P., Palatnik, J. F., et al. (2020). A GRF–GIF chimeric protein improves the regeneration efficiency of transgenic plants. Nat. Biotechnol. 38, 1274–1279. doi: 10.1038/s41587-020-0703-0
Demeke, T., Morris, F. (2002). Molecular characterization of wheat polyphenol oxidase (PPO). Theor. Appl. Genet. 104, 813–818. doi: 10.1007/s00122-001-0847-3
Doench, J. G., Fusi, N., Sullender, M., Hegde, M., Vaimberg, E. W., Donovan, K. F., et al. (2016). Optimized sgRNA design to maximize activity and minimize off-target effects of CRISPR-Cas9. Nat. Biotechnol. 34, 184–191. doi: 10.1038/nbt.3437
Fuerst, E. P., Okubara, P. A., Anderson, J. V., Morris, C. F. (2014). Polyphenol oxidase as a biochemical seed defense mechanism. Front. Plant Sci. 5, 689. doi: 10.3389/fpls.2014.00689
Gao, C. (2021). Genome engineering for crop improvement and future agriculture. Cell 184, 1621–1635. doi: 10.1016/j.cell.2021.01.005
González, M. N., Massa, G. A., Andersson, M., Turesson, H., Olsson, N., Fält, A. S., et al. (2019). Reduced enzymatic browning in potato tubers by specific editing of a polyphenol oxidase gene via ribonucleoprotein complexes delivery of the CRISPR/Cas9 system. Front. Plant Sci. 10, 1649. doi: 10.3389/fpls.2019.01649
Hayta, S., Smedley, M. A., Clarke, M., Forner, M., Harwood, W. A. (2021). An efficient Agrobacterium-mediated transformation protocol for hexaploid and tetraploid wheat. Curr. Protoc. 1, e58. doi: 10.1002/cpz1.58
He, X. Y., He, Z. H., Zhang, L. P., Sun, D. J., Morris, C. F., Fuerst, E. P., et al. (2007). Allelic variation of polyphenol oxidase (PPO) genes located on chromosomes 2A and 2D and development of functional markers for the PPO genes in common wheat. Theor. Appl. Genet. 115, 47–58. doi: 10.1007/s00122-007-0539-8
Hystad, S. M., Martin, J. M., Graybosch, R. A., Giroux, M. J. (2015). Genetic characterization and expression analysis of wheat (Triticum aestivum) line 07OR1074 exhibiting very low polyphenol oxidase (PPO) activity. Theor. Appl. Genet. 128, 1605–1615. doi: 10.1007/s00122-015-2535-8
IWGSC (2018). Shifting the limits in wheat research and breeding using a fully annotated reference genome. Science 361, eaar7191. doi: 10.1126/science.aar7191
Jayakodi, M., Golicz, A. A., Kreplak, J., Fechete, L. I., Angra, D., Bednář, P., et al. (2023). The giant diploid faba genome unlocks variation in a global protein crop. Nature 615, 652–659. doi: 10.1038/s41586-023-05791-5
Liu, H., Ding, Y., Zhou, Y., Jin, W., Xie, K., Chen, L. L. (2017a). CRISPR-P 2.0: An improved CRISPR-Cas9 tool for genome editing in plants. Mol. Plant 10, 530–532. doi: 10.1016/j.molp.2017.01.003
Liu, J., He, Z., Rasheed, A., Wen, W., Yan, J., Zhang, P., et al. (2017b). Genome-wide association mapping of black point reaction in common wheat (Triticum aestivum L.). BMC Plant Biol. 17, 220. doi: 10.1186/s12870-017-1167-3
Liu, C., Zhou, Z., Wu, Z., Qin, M., Shi, X., Wang, Y., et al. (2020). In-depth genetic analysis reveals conditioning of polyphenol oxidase activity in wheat grains by cis regulation of TaPPO2A-1 expression level. Genomics 112, 4690–4700. doi: 10.1016/j.ygeno.2020.08.019
Martin, A., Orgogozo, V. (2013). The loci of repeated evolution: A catalog of genetic hotspots of phenotypic variation. Evolution 67, 1235–1250. doi: 10.1111/evo.12081
Murata, M., Nishimura, M., Murai, N., Haruta, M., Homma, S., Itoh, Y. (2001). A transgenic apple callus showing reduced polyphenol oxidase activity and lower browning potential. Biosci. Biotechnol. Biochem. 65, 383–388. doi: 10.1271/bbb.65.383
Pearce, S., Vazquez-Gross, H., Herin, S. Y., Hane, D., Wang, Y., Gu, Y. Q., et al. (2015). WheatExp: an RNA-seq expression database for polyploid wheat. BMC Plant Biol. 15, 299. doi: 10.1186/s12870-015-0692-1
Raffan, S., Oddy, J., Mead, A., Barker, G., Curtis, T., Usher, S., et al. (2023). Field assessment of genome-edited, low asparagine wheat: Europe’s first CRISPR wheat field trial. Plant Biotechnol. J. 21, 1097–1099 doi: 10.1111/pbi.14026
Rodríguez-Leal, D., Lemmon, Z. H., Man, J., Bartlett, M. E., Lippman, Z. B. (2017). Engineering quantitative trait variation for crop improvement by genome editing. Cell 171, 470–480.e478. doi: 10.1016/j.cell.2017.08.030
Sato, K., Abe, F., Mascher, M., Haberer, G., Gundlach, H., Spannagl, M., et al. (2021). Chromosome-scale genome assembly of the transformation-amenable common wheat cultivar ‘Fielder’. DNA Res. 28, 1–7. doi: 10.1093/dnares/dsab008
Song, X., Meng, X., Guo, H., Cheng, Q., Jing, Y., Chen, M., et al. (2022). Targeting a gene regulatory element enhances rice grain yield by decoupling panicle number and size. Nat. Biotechnol. 40, 1403–1411. doi: 10.1038/s41587-022-01281-7
Stern, D. L., Orgogozo, V. (2008). The loci of evolution: how predictable is genetic evolution? Evolution 62, 2155–2177. doi: 10.1111/j.1558-5646.2008.00450.x
Sullivan, M. L. (2014). Beyond brown: polyphenol oxidases as enzymes of plant specialized metabolism. Front. Plant Sci. 5, 783. doi: 10.3389/fpls.2014.00783
Taketa, S., Matsuki, K., Amano, S., Saisho, D., Himi, E., Shitsukawa, N., et al. (2010). Duplicate polyphenol oxidase genes on barley chromosome 2H and their functional differentiation in the phenol reaction of spikes and grains. J. Exp. Bot. 61, 3983–3993. doi: 10.1093/jxb/erq211
Taranto, F. (2015). Mapping and allelic variations of Ppo-B1 and Ppo-B2 gene-related polyphenol oxidase activity in durum wheat. Mol. Breed 35. doi: 10.1007/s11032-015-0272-y
Taranto, F., Pasqualone, A., Mangini, G., Tripodi, P., Miazzi, M. M., Pavan, S., et al. (2017). Polyphenol oxidases in crops: biochemical, physiological and genetic aspects. Int. J. Mol. Sci. 18, 377. doi: 10.3390/ijms18020377
Tran, L. T., Taylor, J. S., Constabel, C. P. (2012). The polyphenol oxidase gene family in land plants: Lineage-specific duplication and expansion. BMC Genom 13, 395. doi: 10.1186/1471-2164-13-395
van Gelder, C. W. G., Flurkey, W. H., Wichers, H. J. (1997). Sequence and structural features of plant and fungal tyrosinases. Phytochemistry 45, 1309–1323. doi: 10.1016/S0031-9422(97)00186-6
Walkowiak, S., Gao, L., Monat, C., Haberer, G., Kassa, M. T., Brinton, J., et al. (2020). Multiple wheat genomes reveal global variation in modern breeding. Nature 588, 277–283. doi: 10.1038/s41586-020-2961-x
Waltz, E. (2016). Gene-edited CRISPR mushroom escapes US regulation. Nature 532, 293–293. doi: 10.1038/nature.2016.19754
Wang, W., Pan, Q., He, F., Akhunova, A., Chao, S., Trick, H., et al. (2018). Transgenerational CRISPR-Cas9 activity facilitates multiplex gene editing in allopolyploid wheat. CRISPR J. 1, 65–74. doi: 10.1089/crispr.2017.0010
Wang, K., Shi, L., Liang, X., Zhao, P., Wang, W., Liu, J., et al. (2022). The gene TaWOX5 overcomes genotype dependency in wheat genetic transformation. Nat. Plants 8, 110–117. doi: 10.1038/s41477-021-01085-8
Yu, Y., Tang, T., Qian, Q., Wang, Y., Yan, M., Zeng, D., et al. (2008). Independent losses of function in a polyphenol oxidase in rice: differentiation in grain discoloration between subspecies and the role of positive selection under domestication. Plant Cell 20, 2946–2959. doi: 10.1105/tpc.108.060426
Yu, Z., Yunusbaev, U., Fritz, A., Tilley, M., Akhunova, A., Trick, H., et al. (2023). CRISPR-based editing of the ω- and γ-gliadin gene clusters reduces wheat immunoreactivity without affecting grain protein quality. BioRxiv. doi: 10.1101/2023.01.30.526376
Zadoks, J. C., Chang, T. T., Konzak, C. F. (1974). A decimal code for the growth stages of cereals. Weed Res. 14, 415–421. doi: 10.1111/j.1365-3180.1974.tb01084.x
Zhai, S., He, Z., Wen, W., Liu, J., Jin, H., Yan, J., et al. (2020). Genetic architecture of polyphenol oxidase activity in wheat flour by genome-wide association study. Crop Sci. 60, 1281–1293. doi: 10.1002/csc2.20038
Zhang, J., Sun, X. (2021). Recent advances in polyphenol oxidase-mediated plant stress responses. Phytochemistry 181, 112588. doi: 10.1016/j.phytochem.2020.112588
Zhang, S., Zhang, R., Gao, J., Song, G., Li, J., Li, W., et al. (2021). CRISPR/Cas9-mediated genome editing for wheat grain quality improvement. Plant Biotechnol. J. 19, 1684–1686. doi: 10.1111/pbi.13647
Keywords: wheat, polyphenol oxidase, CRISPR/Cas9, multi-target editing, reverse genetics
Citation: Wold-McGimsey F, Krosch C, Alarcón-Reverte R, Ravet K, Katz A, Stromberger J, Mason RE and Pearce S (2023) Multi-target genome editing reduces polyphenol oxidase activity in wheat (Triticum aestivum L.) grains. Front. Plant Sci. 14:1247680. doi: 10.3389/fpls.2023.1247680
Received: 26 June 2023; Accepted: 28 August 2023;
Published: 15 September 2023.
Edited by:
Junhua Peng, Spring Valley Agriscience Co., Ltd, ChinaReviewed by:
Zujun Yang, University of Electronic Science and Technology of China, ChinaXingguo Ye, Chinese Academy of Agricultural Sciences, China
Copyright © 2023 Wold-McGimsey, Krosch, Alarcón-Reverte, Ravet, Katz, Stromberger, Mason and Pearce. This is an open-access article distributed under the terms of the Creative Commons Attribution License (CC BY). The use, distribution or reproduction in other forums is permitted, provided the original author(s) and the copyright owner(s) are credited and that the original publication in this journal is cited, in accordance with accepted academic practice. No use, distribution or reproduction is permitted which does not comply with these terms.
*Correspondence: Stephen Pearce, c3RlcGhlbi5wZWFyY2VAcm90aGFtc3RlZC5hYy51aw==