- 1National Engineering and Technology Center for Information Agriculture, Nanjing Agricultural University, Nanjing, China
- 2Ministry of Education Engineering Research Center of Smart Agricultural, Nanjing Agricultural University, Nanjing, China
- 3Ministry of Agriculture and Rural Affairs Key Laboratory for Crop System Analysis and Decision Making, Nanjing Agricultural University, Nanjing, China
- 4Jiangsu Key Laboratory for Information Agriculture, Nanjing Agricultural University, Nanjing, China
- 5Institute of Smart Agriculture, Nanjing Agricultural University, Nanjing, China
- 6Jiangsu Cooperation and Innovation Center for Modern Crop Production, Nanjing, China
- 7Institute of Sanya, Nanjing Agricultural University, Sanya, China
Introduction: Optimizing nitrogen (N) fertilizer management is key to reducing active N losses in farmland. While current research has proposed various methods to minimize N losses, it often overlooks the need for integrated strategies that address both N losses and crop productivity. By clarifying the impact of different N fertilizer optimization practices on crop productivity and N loss, rational N fertilizer management strategies can be adopted to achieve stable crop yields and reduction in N application rates.
Methods: Our study collected 476 peer-reviewed papers and used meta-analysis to analyze the impact of different N optimization management practices (combined application of organic-inorganic fertilizer, deep fertilization, and enhanced-efficiency fertilizer) on yield, N use efficiency, and N loss (N2O emissions, NH3 volatilization, N runoff, and N leaching).
Results: Optimized N management led to a 5%-10% increase in yield, a 17%-21% improvement in N use efficiency, and reductions in N2O (10%-37%), NH3 (7%-40%), N runoff (9%-20%), and N leaching (7%-10%) compared to standard farmer management practices.
Conclusion: Twice fertilization, the combination of organic and inorganic fertilizers, and the appropriate application of enhanced-efficiency fertilizer could improve rice yield, decrease N losses and ensure food security and improve the sustainable agricultural development.
1 Introduction
Nitrogen (N) is an essential element for crop growth and development (Kakar et al., 2020), and its fertilization improves crop yields. However, excessive N application, often as an “insurance” component to prevent yield loss, is common in china rice production (Ju et al., 2009). At present, the yield returns diminish with increased fertilizer application rates. Furthermore, China faces the world’s largest N surplus and the lowest N use efficiency (NUE) (Zhang et al., 2015), which contributes to the imminent risk of N pollution. Excessive active N not only affects crop yield and quality (Jin, 2018) but also causes a variety of environmental issues, including air pollution (Behera et al., 2013), global warming (IPCC, 2013), and water eutrophication (Di and Cameron, 2002; Ju et al., 2006). Therefore, effective N fertilizer management is crucial for sustaining rice yields while minimizing environmental risks in rice production systems.
The application rate of N fertilizer is a key factor influencing N losses from farmland. N losses typically peak within 1 to 3 days after fertilizer application (Chen et al., 2016; Qiao et al., 2021; Wang et al., 2012), and excessive N application significantly exacerbates these losses. Improving N fertilizer practices and increasing NUE are important strategies to reduce N losses. Appropriately reducing N application rates can substantially mitigate these losses (Zhang et al., 2017; Zhong et al., 2017). However, insufficient N application may also lead to a decline in crop yields. Increasing the frequency of fertilizer applications and delaying topdressing are effective ways for reducing N losses while maintaining grain yield (Bai et al., 2020; Huang et al., 2016). Moreover, the N fertilizer management strategies significantly affect N losses. Altering fertilizer placement can reduce N leaching, and replacing some inorganic fertilizer with organic fertilizer can minimize ammonia (NH3) volatilization (Xia et al., 2020; Yao et al., 2018). The application of enhanced efficiency N fertilizer (slow-release fertilizer, SRF; nitrification inhibitors, NI; urease inhibitors, UI) can effectively reduce N environmental emissions without reducing yield (Lan et al., 2021; Liu et al., 2016; Tang et al., 2018; Xue et al., 2011). These N fertilizer management practices often only focus on one type of N loss, potentially increasing other types of N losses through different pathways. For example, while nitrification inhibitors reduce N leaching, N runoff, and nitrous oxide (N2O) emissions, they may also increase NH3 volatilization (He et al., 2022; Liu et al., 2022; Wang et al., 2016). Similarly, crop straw input promoted the increase of N2O emissions and reduced N leaching (Janz et al., 2022). Therefore, it is essential to consider multiple factors when developing fertilization strategies to minimize N losses while maintaining yields. In recent years, meta-analyses have been widely used in agronomy to analyze the effect of different N fertilizer management practices on N losses and crop yield. Wei et al. (2021) performed a meta-analysis to quantitatively compare how different organic fertilizer application methods affect N leaching and N runoff. Li et al. (2021) comprehensively assessed the global effects of N fertilizer types and crop residue types on N2O emissions and N leaching. Other studies focused on the effects of N fertilization replacement on rice yield and NUE (Ding et al., 2018). However, these aforementioned studies and N fertilizer management strategies mainly focused on individual N fertilizer management measures or their effects on the loss of a single N form, lacking a comprehensive assessment of their combined impact on both environmental and crop productivity. Therefore, it is imperative to explore the effects of different N fertilizer optimization practices on yield and N losses to develop more effective and sustainable N fertilizer management strategies.
The middle and lower reaches of the Yangtze River are China’s main rice production areas, contributing 51% of the national rice output (China, N.B.O.S, 2019). However, the high intensity of N fertilizer use and improper application methods in this region have made it a hotspot for increased N and N2O emissions from farmland (Ju and Gu, 2014; Sun et al., 2018). The growing environmental pollution from excessive fertilization has led to numerous field studies on N losses from farmland (Cao et al., 2014; Lan et al., 2020; Shi et al., 2020), providing valuable data for a comprehensive assessment of N losses and their influencing factors in this region. Therefore, relevant literature should be searched and analyzed to study the effects of optimized N management on crop productivity and N losses in the lower reaches of the Yangtze River in China, including three N fertilizer optimization management measures (combined application of organic and inorganic N fertilizers; deep application of N fertilizers; enhanced-efficiency N fertilizers), in order to: 1) quantifying the effects of optimized N management on NUE and grain yield, 2) determining the influences of optimized N management practices on N losses in paddy fields, and 3) exploring optimal management strategies for different N management practices.
2 Data analysis
2.1 Data collection
The relevant literature from 2000 to 2023 was searched in the Web of Science, CNKI, and Wanfang databases for the meta-analysis undertaken in this study. To identify relevant studies and literature, the following keywords and their corresponding Chinese words were queried: “rice yield”, “rice NUE”, “N loss”, “N balance”, “ammonia volatilization”, “nitrous oxide emission”, “N runoff”, “N leaching”, etc. The collected literature data must satisfy the following requirements to be considered: 1) a rice field experiment performed in the middle and lower reaches of the Yangtze River (Figure 1); 2) either a zero N level or conventional N application level was used as a control; 3) at least one target variable was quantified (yield, NUE (partial fertilizer productivity), nitrous oxide emissions, ammonia volatilization, N runoff, N leaching); 4) each treatment was performed in at least three replicates; 5) the standard deviations was available or calculable.
A total of 476 peer-reviewed papers were extracted. From the studies, we collected the following data: (1) mean annual temperature (MAT), mean annual precipitation (MAP), and geographical coordinates of each experimental site; (2) soil pH, soil total N content (TN), soil organic carbon content (SOC); (3) yield, NUE, NH3 emissions, N2O emissions, N runoff, N leaching. Regarding data presented in a table format in the studies, the required data were directly extracted. Data presented in graphs, the GetData Graph Digitizer 2.24 software was used to digitize and extract the data from the graphs.
2.2 Meta-analysis
According to the N fertilizer management practice in the field, the data were divided into four categories (Table 1): (1) conventional N fertilizer management practice; (2) combined application of organic and inorganic N fertilizers; (3) deep application of N fertilizers; (4) enhanced-efficiency N fertilizers (slow- release fertilizer, nitrification inhibitor, urease inhibitor). We analyzed the effects of different N fertilizer optimization management practice on crop yield and N losses according to fertilizer application rate, fertilizer application frequency, fertilizer type and substitution ratio (SR) (Supplementary Table S1). The NUE in this study was calculated as the partial factor productivity (1). The impact of different N fertilizer management practices on crop productivity and N losses was quantified using the natural logarithm of response ratio (lnRR) as the effect size (Hedged et al., 1999), calculated by Formula 2:
In the formula, Xt represents the yield, NUE, or N losses of treatment, and Xc represents the yield, NUE, or N losses of control. The data divided into two groups, the first for conventional fertilizer management practice (control) and N fertilizer optimization management practice (treatment), and the second for no fertilization (control) and conventional fertilizer management (treatment).
The variance () of each study was estimated as follows:
nt and nc correspond to the sample size of the treatment group and the control group. SDt and SDc are the standard deviations of the treatment and control groups, respectively. If only the standard error was provided, it was converted by Formula 4. If not provided, 10% of the average was used as the standard error (Luo et al., 2006).
The meta-analysis was performed using a nonparametric weighting function, and the weighting factor (Wij), weighted response lnRR++, and standard error S(lnRR++) was calculated as follows:
where m is the number of groups and k was the number of comparisons The 95% confidence interval (CI) of lnRR++ is calculated according to (Curtis and Wang, 1998):
If the 95% confidence interval (CI) range included 0, there was no difference between treatment and control. When p< 0.05, the difference was considered significant.
After calculating the variance () of each group, the restricted maximum likelihood estimation (REML) method of the “metafor” package in R (version 4.2.1) was used to estimate the effect magnitude. To make the results more intuitive, lnRR was converted into a percentage form (%change=(eln(RR)-1)×100%) and plotted with ‘ggplot2 ‘ (version 4.2.3). Random forest (RF) was used to determine the importance of meteorological and soil factors on N loss. The RF procedure was implemented using the “randomForest” package in R (version 4.2.3). SPSS v.12.5 was used to assess the correlations between the LnRR and meteorological and soil factors by the Person correlation coefficient. In addition, the egger test was used to evaluate the publication bias (Supplementary Table S2).
3 Result
3.1 The overall effect of N fertilizer optimization management practices on crop productivity and N losses
N management optimization significantly increased crop productivity and reduced N loss compared to conventional fertilization. The combined application of organic and inorganic fertilizers increased yield and NUE by 10% and 17%, respectively. Moreover, it reduced N2O, NH3, N runoff, and N leaching by 10%, 7%, 20%, and 7%, respectively (Figure 2a). The application of enhanced efficiency N fertilizers increased yield and NUE by 4% and 18%, and reduced N2O, NH3, N runoff, and N leaching by 40%, 23%, 9%, and 10%, respectively (Figure 2b). N fertilizer deep application increased the yield and NUE by 9% and 21%, and reduced NH3 volatilization and N2O emissions by 40% and 30% (Figure 2c).
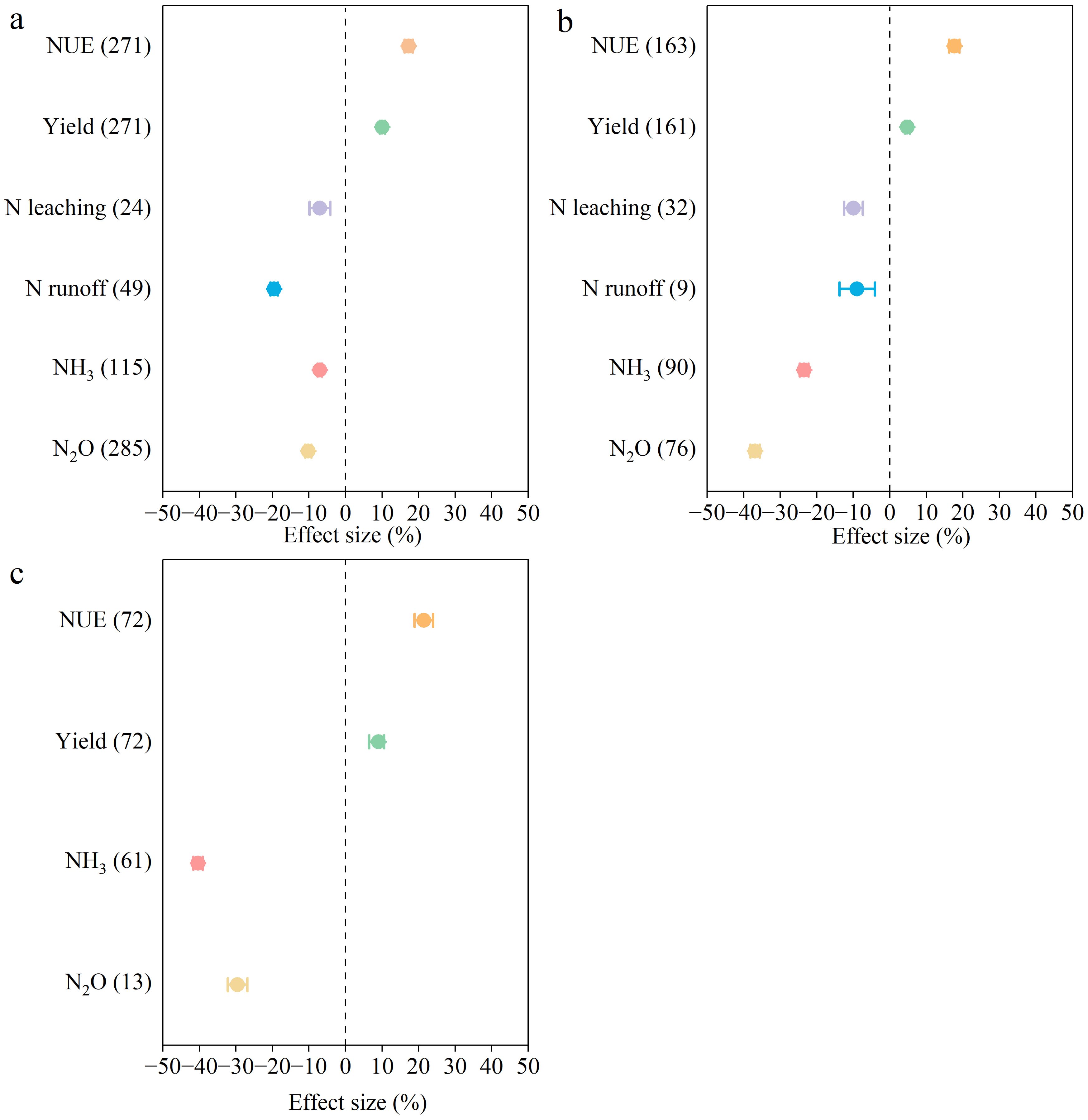
Figure 2. The overall impact of optimized N fertilizer management practice on crop yield, NUE, N2O emissions, NH3 emissions, N runoff, and N leaching (a). combined application of organic and inorganic N fertilizers (b) enhanced-efficiency N fertilizer (c) deep placement of N fertilizer). The mean effect and 95% CI are shown. When the CI does not overlap with zero, the response is considered as significant. Numbers in parentheses indicate the number of observations.
3.2 N losses and crop productivity responses to conventional N fertilizer management practices
Regarding the conventional N fertilizer management practices, with the increase of N application rate and fertilizer application frequency, the yield correspondingly gradually increased. However, when the N application rate exceeded 330 kg/ha, the yield tended to decrease (Figure 3a). To enhance yield under the conventional N fertilizer management practices, the optimal N fertilizer application rate is 270< N ≤ 330 kg/ha with 4 application times.
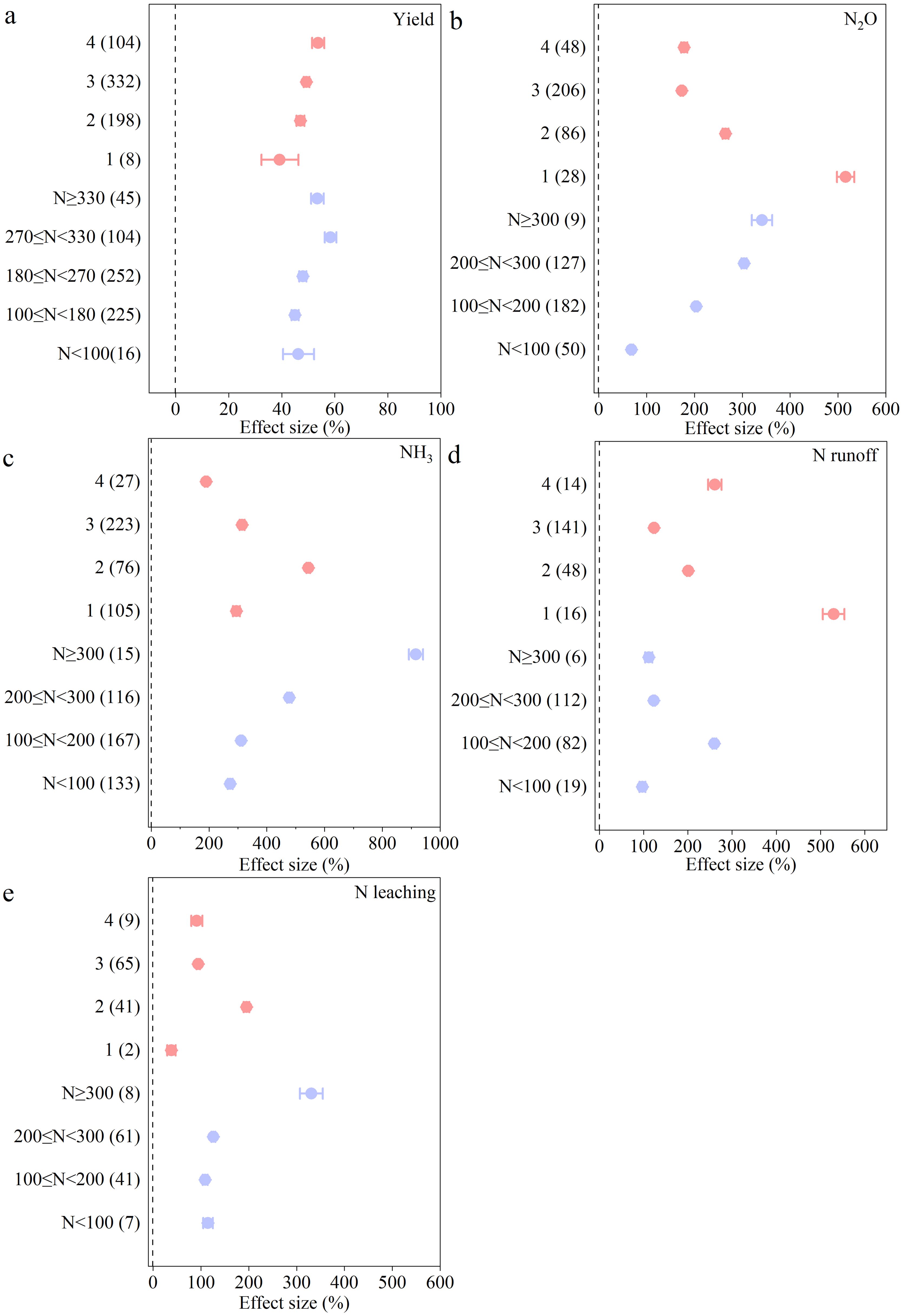
Figure 3. The effects of inorganic N fertilizer applications on crop yield, compared to no N fertilizer applied: (a) Yield, (b) N2O, (c) NH3, (d) N runoff, and (e) N leaching. The mean effect and 95% CI are shown. When the CI does not overlap with zero, the response is considered as significant. Numbers in parentheses indicate the number of observations.
The conventional N fertilizer management practices increased N2O, NH3, N runoff, N leaching by 208%, 395%, 171%, and 128% compared to the absence of N. With the increase in N application rate, N losses increased gradually. The extent of N losses was significantly decreased as N fertilizer application frequency increased (Figure 3). The N loss is the lowest when the fertilizer application rate is less than 100 kg/ha. Compared with a high N application rate (N ≥ 300 kg/ha), lower amount of N fertilizer (N< 100 kg/ha) reduced N2O emissions, NH3 volatilization, N runoff, and N leaching by 80%, 78%, 13%, and 65%, respectively. The optimal fertilizer application frequency of N2O and N runoff was 3 times, and for NH3 and N leaching was 4 times.
3.3 Yield and NUE responses to optimized N management
Compared with the conventional management practices, the combined organic-inorganic fertilizer application had a greater effect on increasing yield especially at higher fertilizer application rates (N > 300 kg/ha). The combination of organic and inorganic fertilizer application performed the best to increase yield and efficiency at the fertilization frequency of 4 times (Figure 4a). With the increase in the N fertilizer replacement value, the effect of organic fertilizers on yield and NUE was reduced. At a lower replacement ratio (SR ≤ 0.3), the combined application of organic-inorganic N fertilizers significantly increased yield and NUE by 12% and 22%. The application of organic fertilizer following the application of inorganic fertilizer also had the same effect, increasing the yield and NUE by 10% and 20%, respectively. The type of organic fertilizer also significantly affected yield and NUE. Rapeseed cake fertilizer and manure had a greater effect on yield increase, and green manure and mixed organic fertilizer had a greater effect on NUE.
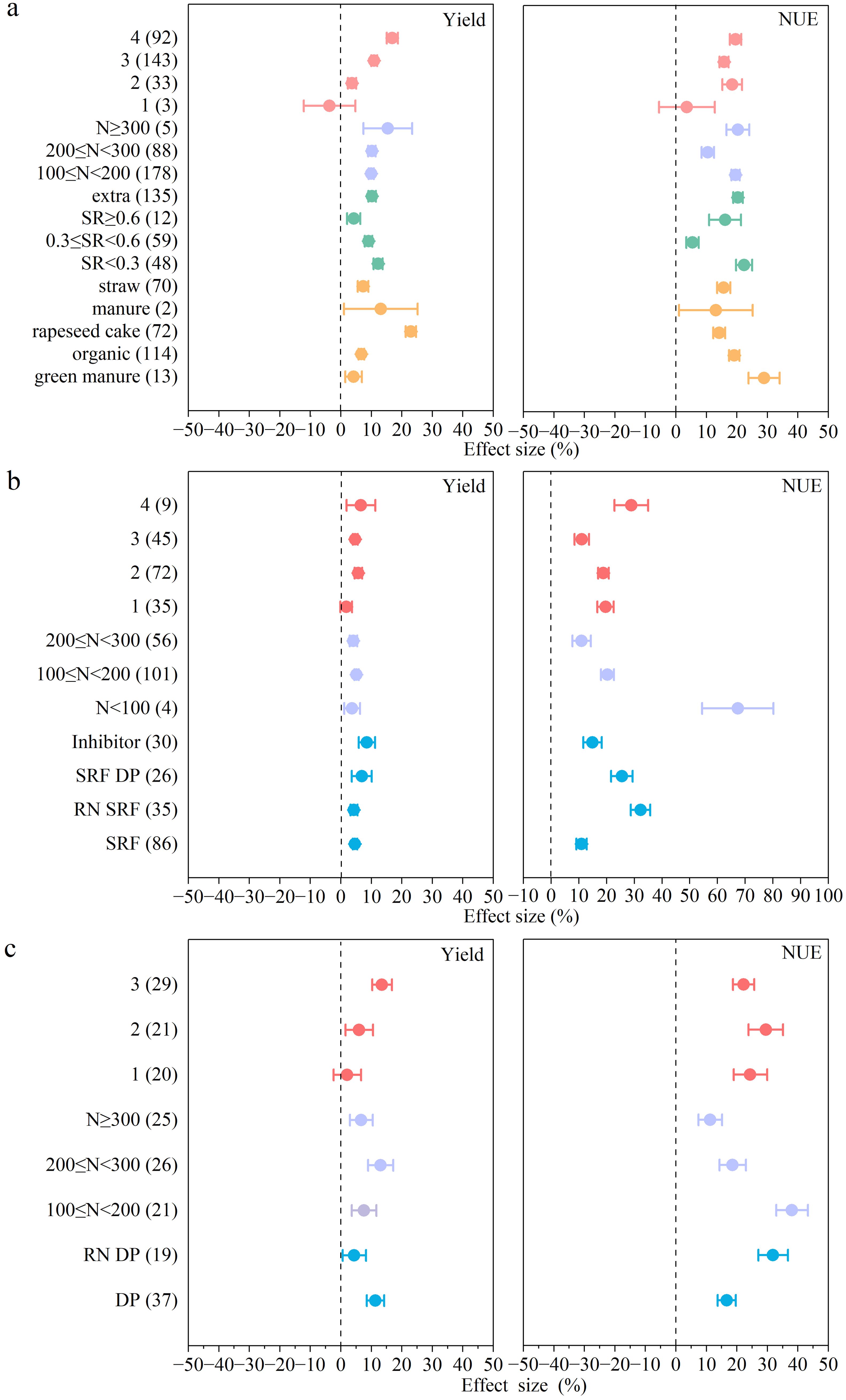
Figure 4. The effect of optimized N fertilizer management practice on crop yield, NUE ((a). combined application of organic and inorganic N fertilizers (b) enhanced-efficiency N fertilizer (c) deep placement of N fertilizer). The mean effect and 95% CI are shown. When the CI does not overlap with zero, the response is considered as significant. Numbers in parentheses indicate the number of observations.
With the increase in N application rate, the effect of SRF application on yield and NUE was reduced (Figure 4b). The enhanced-efficiency N fertilizer was more optimal for yield increase at lower N application rates (100< N ≤ 200 kg/ha) (Figure 4b). The application of enhanced-efficiency N fertilizers resulted in the most increase in yield and NUE of 7%, 29% when fertilizer was applied 4 times. At a N application rate of N< 100 kg/ha, SRF exhibited the greatest effect in improving NUE by 67%, but their effect on yield increase was not significant. Under a lower N application rate (100< N ≤ 200 kg/ha), applying enhanced-efficiency N fertilizers increased the yield and NUE by 5% and 20%. When inorganic N fertilizer was applied, the effect on yield increase by the combined application of inhibitors was stronger than that of SRF, while the opposite effect was observed on NUE. The application of slow-release fertilizers and the reduction of N application rate significantly increased the NUE by 32%, but the yield was only 4% higher compared to the conventional management practices.
The effect of deep placement of N fertilizer on NUE improvement gradually weakened with the increase in N application rate. NUE improvement was the most significant, increasing by 38% when the fertilizer application rate was (100< N ≤ 200 kg/ha). Under the higher fertilizer application rates (200< N ≤ 300 kg/ha), the deep placement of N fertilizer had the highest effect on yield, which was increased by 13% (Figure 4c). Specifically, increasing N application times had a greater effect on yield and efficiency. Compared to the conventional fertilizer management practice, 3-time-fertilization increased the yield by 13% and twice-fertilization improved the NUE by 21%. Compared with the deep placement of the regular N application rates, deep application of RNDP increased NUE by 91%. Although the yield under this treatment was decreased by 62%, it was still higher than conventional management.
3.4 N losses in response to optimized N management practices
N application rate and fertilization frequency also significantly affected the extent of N loss after combined organic and inorganic fertilizer application (Figure 5). The greatest effect on the reduction of emissions and N losses was observed under the lower N application rate (100< N ≤ 200 kg/ha). Increased N application rates resulted in an increase in nitrous oxide emissions, ammonia volatilization, and N runoff. Compared to the standard management practices followed by the farmers, the replacement of inorganic N with organic fertilizer at certain ratios could reduce N losses in paddy fields. A ratio of 0.3 ≤ SR< 0.6 most significantly reduced N2O emissions and N leaching, by 30% and 11%, respectively. At SR< 0.3, NH3 volatilization and N runoff were most significantly reduced by 24% and 22%, but a further increase in the ratio of organic fertilizer application increased NH3 emissions. Except for the increase in N losses due to NH3 volatilization under the straw return practice, the application of other types of organic fertilizers reduced N losses, among which manure had the most significant effect on N emissions reduction. The application of rapeseed cake fertilizer reduced N2O emissions, NH3 volatilization, and N leaching but did not significantly affect N runoff. The application of green manure significantly reduced ammonia volatilization and N leaching by 31% and 21%, respectively.
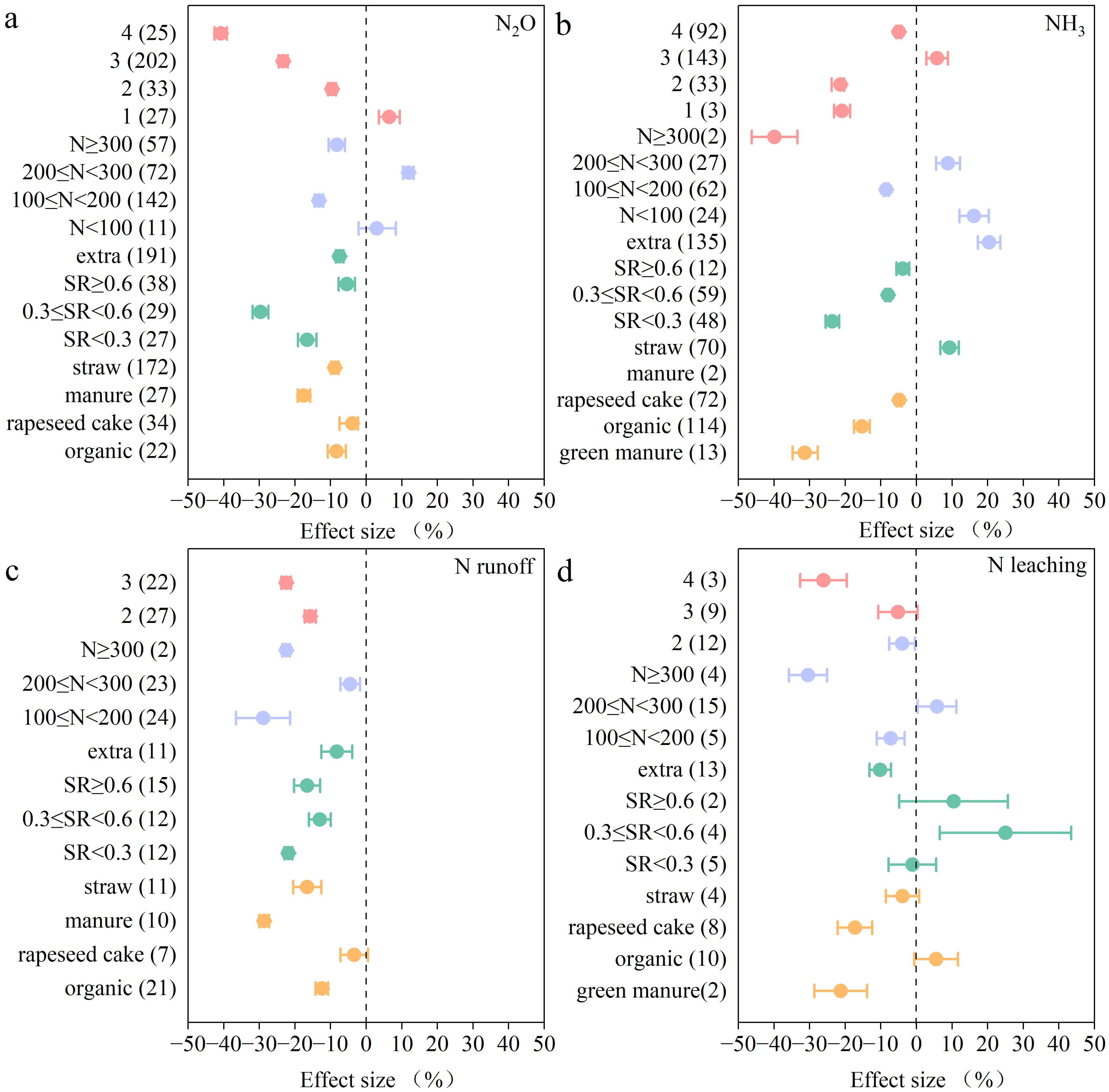
Figure 5. The effects of organic fertilizer application compared with conventional practices on: (a) N2O, (b) NH3, (c) N runoff, and (d) N leaching. The mean effect and 95% CI are shown. When the CI does not overlap with zero, the response is considered as significant. Numbers in parentheses indicate the number of observations.
Enhanced-efficiency fertilizers significantly reduced N losses. At a 100< N ≤ 200 kg/ha N application rate, total N losses were significantly reduced, specifically by 47% for N2O, 40% for NH3, 9% for N runoff, and 24% for N leaching. Fertilization application frequency significantly affected the effect of the enhanced-efficiency N fertilizer on emission reduction. When fertilization was applied twice, the best emission reduction effect was achieved when using inorganic N fertilizer as the base fertilizer and SRF as the top dressing. However, with the increase in fertilization frequency, the effect of reduced ammonia volatilization decreased, increasing NH3 volatilization by 6% with a fertilization frequency of 4 applications.When applying inorganic fertilizers, inhibitor application had a greater effect on reducing N2O emissions and N leaching compared to SRF. Still, the application of inhibitors significantly increased NH3 volatilization by 17% (Figure 6b).
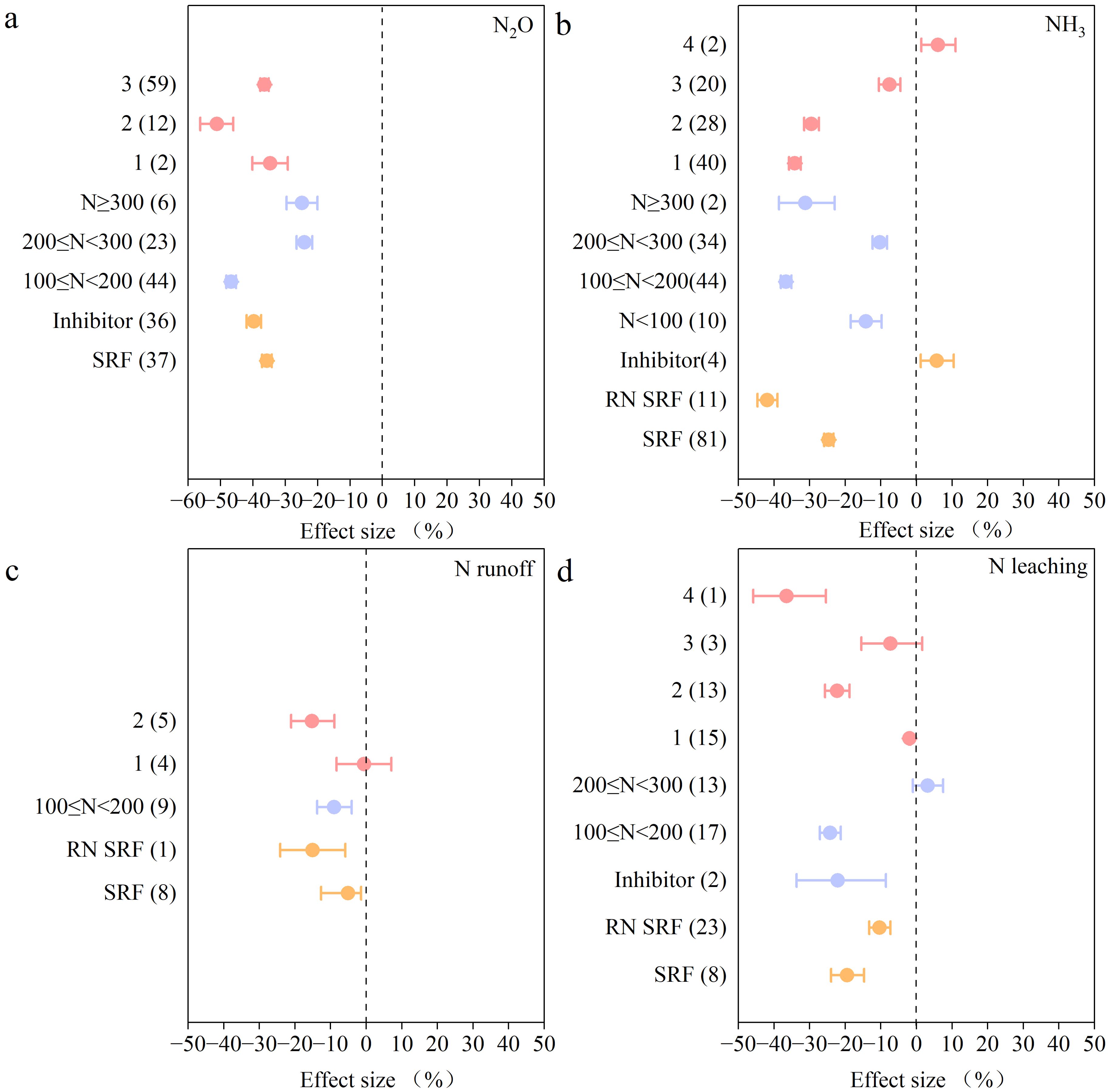
Figure 6. The effects of applying enhanced-efficiency N fertilizer compared with conventional practices on: (a) N2O, (b) NH3, (c) N runoff, and (d) N leaching. The mean effect and 95% CI are shown. When the CI does not overlap with zero, the response is considered as significant. Numbers in parentheses indicate the number of observations.
Deep placement of N fertilizer significantly reduced Nous gas emissions in paddy fields. The emission of N2O was significantly reduced at a 100< N ≤ 200 kg/ha application rate with twice fertilization applications. A 200< N ≤ 300 kg/ha application rate and a frequency of three fertilization applications significantly affected NH3 volatilization reduction (Figure 7b). We also found that RNDP had a greater effect on reduced N losses than the standard N application. Compared with deep application of the standard amount of N (DP), RNDP further reduced N2O emissions by 39%, reduced NH3 volatilization by 31%, and increased NUE by 91%. Due to data limitations, the effects of deep application of N fertilizers on N runoff and N leaching are not discussed here.
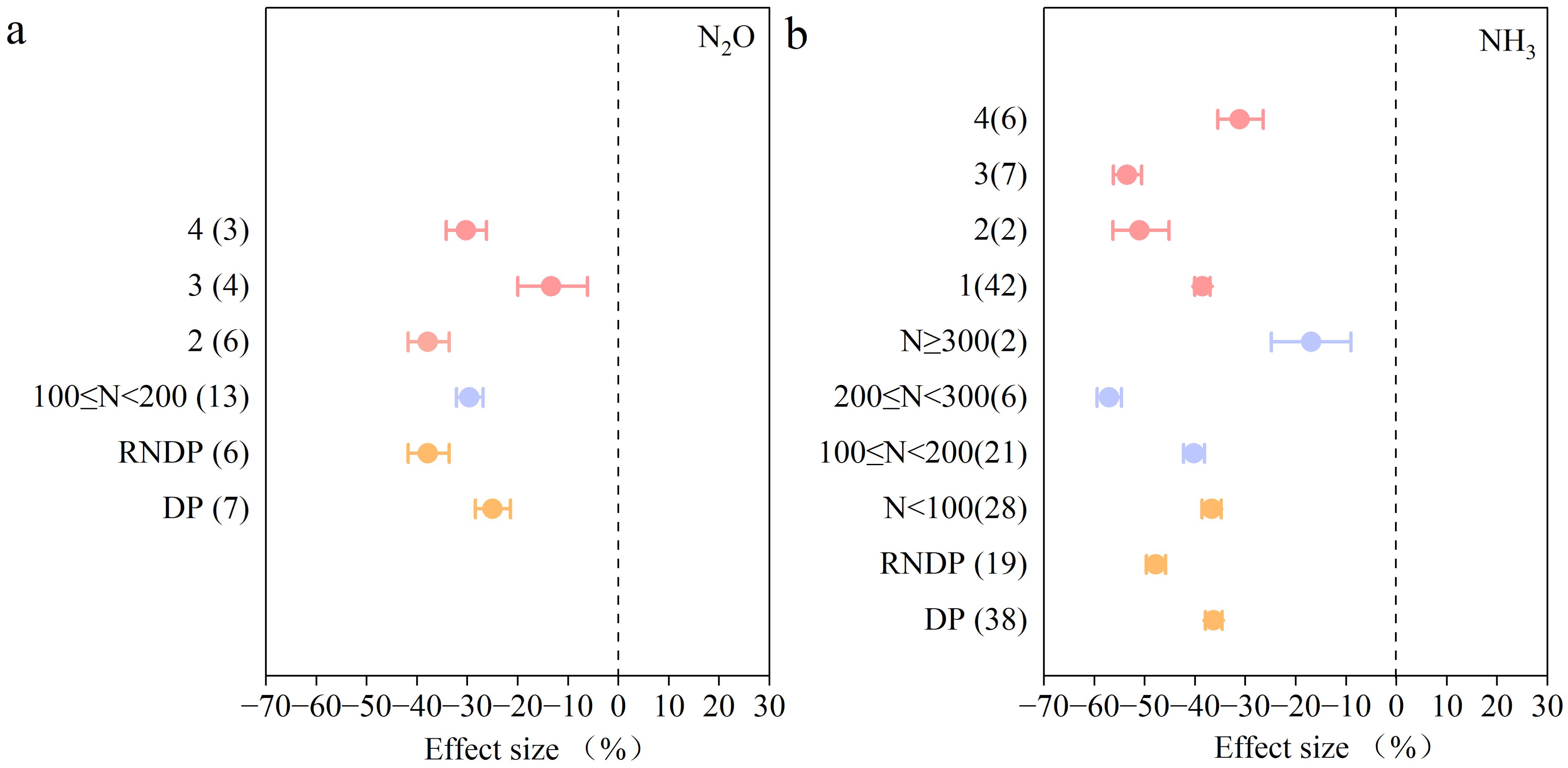
Figure 7. The impact of deep soil application of N fertilizers compared with conventional practices on: (a) N2O, and (b) NH3. The mean effect and 95% CI are shown. When the CI does not overlap with zero, the response is considered as significant. Numbers in parentheses indicate the number of observations.
3.5 The impact of different climate and soil factors on N losses under optimized N fertilizer management practices
N application rate had the greatest impact on N2O emissions, followed by SOC, MAP, and TN. For NH3, N application rate also had the greatest impact, followed by SOC, pH, and TN. Among all types of N losses, N fertilizer application rate was an important factor that affected total N losses, but it was not always the dominant factor. Regarding hydrological N losses, the effects of MAP, MAT, and pH are greater than the amount of N applied (Table 2).
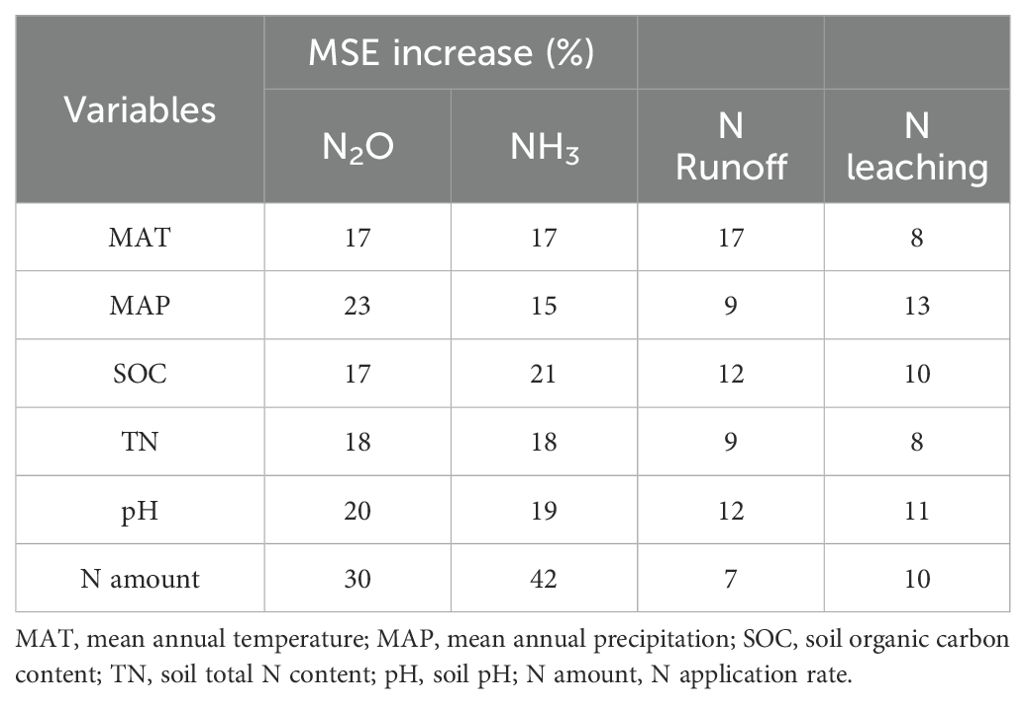
Table 2. Relative importance of independent variables influencing N2O emissions, NH3 volatilization, N runoff and N leaching under optimal N management practices as determined using random forest (RF) models.
4 Discussion
4.1 Effects of combined application of organic and inorganic fertilizers on crop productivity and N loss
The delayed nutrient release and low N content of organic fertilizers can lead to reduced crop yield if they fail to meet crop N demands on time (Röös et al., 2018; Seufert et al., 2012). However, combining organic and inorganic fertilizers can significantly enhance crop productivity (Pan et al., 2009). Our results showed that this combination increased yield by 10%, NUE by 17%, and reduced N2O, NH3, N runoff, and N leaching losses by 10%, 7%, 20%, and 7%, respectively. Organic fertilizers stimulate microbial growth, increasing biomass and microbial residue accumulation, which enhances mineral N content and improves soil N supply, thus boosting N absorption by crops and reducing N losses (Mohanty et al., 2013; Xia et al., 2021; Zhou et al., 2023). They also improve soil water-holding capacity (Vengadaramana and Jashothan, 2012) and promote NH4+ adsorption, reducing ammonium N concentrations (Stewart and Carter, 1996; Zhou et al., 2013). Moreover, organic matter decomposition in soil consumes oxygen, inhibiting mineralization and nitrification. In oxygen-deprived conditions, nitrous oxide is produced during denitrification, reducing N losses (Beauchamp et al., 1989). Humus formed from organic fertilizers improves soil structure and prevents nutrient leaching. Organic fertilizers can also lower soil pH, contributing to plant growth (Keeney, 1982; Singh et al., 2020). The effects of different organic fertilizers on yield and NUE vary. Rapeseed cake (23%) > manure (13%) > straw (7%) = organic fertilizer (7%) > green manure (4%) for yield enhancement. For NUE, the ranking is: green manure (29%) > organic fertilizer (19%) > straw (16%) > rapeseed cake (14%) > manure (13%). These differences are due to varying application methods. Rapeseed cake and straw are often applied with inorganic N fertilizers, leading to substantial yield increases but decreased NUE. In contrast, rapeseed cake, green manure, and organic fertilizers typically replace some inorganic N, reducing N input and improving NUE. The impact of organic fertilizers on N loss is debated. Some studies show reduced N2O emissions with organic fertilizers (Ma et al., 2019), while others suggest straw return increases N2O emissions (Jacinthe and Lal, 2003; Wu et al., 2018). Meta-analyses indicate no significant effect on N runoff or leaching from organic fertilizers in paddy fields (Wang et al., 2019). Our study found organic fertilizers significantly reduced N losses, which could be due to fertilizer type differences. Manure, with a lower C/N ratio and faster nutrient release, may increase N losses (Chantigny et al., 2001). In contrast, straw has a higher C/N ratio and fewer N compounds, increasing the N pool and SOC, which reduces N losses. Green manure improves N availability and soil health, promoting better root growth and nutrient absorption, thus increasing yield (Kumar et al., 2014; Mohanty et al., 2013; Srinivasarao et al., 2014). Our study also suggests that manure is more effective than other organic fertilizers in reducing emissions and improving yield (Figures 3, 5). Organic fertilizers promote microbial N fixation, reducing N volatilization and leaching losses by preventing excess inorganic N from being unused by crops. They enhance soil N content, improving NUE and yield (Cao et al., 2018). Manure, in particular, enhances soil quality, provides supplementary nutrients (P, Ca, Fe), and boosts soil enzyme activity. Recent studies show manure is more effective than other fertilizers in improving N supply and soil structure (He et al., 2015; Hou et al., 2023; Ye et al., 2019), making it highly beneficial for increasing yield and reducing N losses. However, our results indicate that straw return to the field increases NH3 volatilization, which aligns with previous studies (Sun et al., 2018; Xu et al., 2017). During straw decomposition, alkaline cations are released, raising ammonium N concentration and pH, which promotes ammonia volatilization (Wang et al., 2012).
Combining organic and inorganic fertilizers reduces mineral N application and enhances soil nutrient content, improving water and soil conservation. This approach promotes higher N uptake, reducing N losses. The effects depend on the organic N substitution ratio, with a higher substitution ratio leading to slower nutrient release from organic matter and reduced N availability. Excessive substitution can lower NUE and yield, particularly during early growth (Ding et al., 2018; Liu et al., 2021). Our findings suggest that the yield-increasing effect of organic-inorganic fertilizer combinations decreases with higher substitution ratios, indicating that excessive substitution may negatively impact crop yields. Therefore, maintaining a suitable substitution ratio (recommended ≤30%) is crucial for optimizing organic-inorganic fertilizer combinations (Meng et al., 2009; Liu et al., 2021). Studies show that this ratio improves soil fertility, increases yields, and reduces N losses, particularly in rice production systems (Zhang et al., 2020; Ren et al., 2022).
4.2 Effects of application of enhanced-efficiency N fertilizers on crop productivity and N losses
Slow and controlled-release fertilizers improve NUE and reduce losses by aligning nutrient release with crop needs and absorption capacity (Geng et al., 2015). Compared to conventional fertilizers, SRF effectively limit N losses (Li et al., 2018; Wang et al., 2007; Yang et al., 2015). Our meta-analysis indicates that, with an N application rate of 100 ≤ N< 200 kg/ha, enhanced-efficiency N fertilizers reduce N2O emissions and N leaching by 47% and 24%, respectively. Compared to standard farmer practices, this approach increased yield by 5%, NUE by 20%, reduced NH3 volatilization by 24%, and decreased N runoff by 9% (Figures 3, 6). Controlled-release fertilizers gradually release nutrients, with lower early-stage release and higher release later, avoiding nutrient leaching during the rainy season and promoting N absorption during active and reproductive growth phases. This reduces N concentration in soil leachate and mitigates N leaching (Du et al., 2007; Zheng et al., 2004; Trinh et al., 2015). In contrast to available chemical fertilizers that promote exchangeable Ca2+ and Mg2+ leaching, damaging soil structure and fertility (Zhang et al., 2001), controlled-release fertilizers help maintain soil structure and reduce N losses. Our study found that using SRF instead of conventional practices increased yield by 5% and NUE by 11%. When RN SRF was used, yield increased by 4%, and NUE rose by 32%. However, the exact proportion of SRF in total N application was not specified in this study and warrants further investigation.
Nitrification inhibitors are widely used to delay urea hydrolysis. They inhibit nitrification, reduce microbial biomass, improve plant N uptake, and reduce N2O emissions (Meng et al., 2020). Urease inhibitors extend urea N retention by regulating soil urease activity and slowing hydrolysis (Tian et al., 2020). In our study, the use of nitrification inhibitors improved yield by 5%, NUE by 14%, and reduced N2O emissions by 40%. However, the increased retention time of NH4+ in the soil raises NH3 volatilization risks (Kim et al., 2012), with our results confirming a 17% increase in NH3 emissions during rice cultivation (Figure 6d). Meta-analyses by Fan et al. (2022) showed that urease and nitrification inhibitors reduce NH3 loss and N2O emissions while boosting crop yields. However, the effects of these inhibitors vary based on temperature, soil texture, and crop species. While they reduce gaseous N loss, they extend N retention in soil, which may increase the risk of N leaching and runoff under flooded conditions, requiring further research to understand these dynamics.
4.3 Effects of deep N fertilizer placement on crop productivity and N losses
At N application rates of 100 ≤ N< 200 kg/ha, NUE increased by 38%, and yield increased by 8%. Our results, unlike previous studies (Zhong et al., 2021), show that deeper fertilization also significantly inhibits NH3 volatilization at high N application rates. Deep placement brings nutrients closer to the plant roots, maintaining a high NH4+-N concentration in the root zone. As roots grow through this nutrient-rich zone, their capacity to absorb nutrients increases, enhancing N uptake and overall growth (Angela, 2004). A continuous high N supply at the early growth stage boosts tiller number, grains per spike, and aboveground biomass (Liu et al., 2016). Thus, deep fertilization can improve NUE and increase yield.
Deep placement of N fertilizers is effective in reducing ammonia volatilization (Yao et al., 2018; Zhong et al., 2021), with our meta-analysis supporting this finding. Deep placement reduced NH3 emissions by 40% and N2O emissions by 30%. This practice stimulates root development, reduces N fixation and adsorption, and enhances nutrient availability (Su et al., 2015; Shen et al., 2013). Deep placement also increases NH4+ adsorption by soil colloids, inhibits urease activity, and reduces NH4+ concentration and surface water pH (Liu et al., 2015), further reducing NH3 volatilization. In flooded paddy fields, strong soil reduction conditions promote denitrification, reducing N2O production (Liu et al., 2010; Hou et al., 2012; Liu et al., 2012; Yang et al., 2012). Additionally, deep N fertilizer placement reduces interaction with nitrification or denitrification microorganisms, effectively reducing N2O emissions (Liu T. et al., 2020). However, deep fertilization may lead to N accumulation in the root zone, exceeding plant uptake capacity and increasing the risk of N leaching losses (Zhao et al., 2012). Therefore, careful consideration of fertilizer placement depth is necessary. Although many studies do not specify the depth of N fertilizer placement, future research should focus on determining the optimal depth to draw stronger conclusions about its impact on ammonia volatilization. Moreover, while SRF, DP, and RNDP reduced N losses and improved NUE, they did not enhance crop yield (Figures 3, 7). Deep N fertilizer placement can increase yield, NUE, and reduce gaseous N losses, but further research is needed to explore its effect on N discharge into water bodies. Limited studies have been conducted, making it difficult to draw definitive conclusions through meta-analysis. Additionally, deep placement extends the retention time of N in the soil, which may reduce N losses for the current season but requires further study of its long-term effects on subsequent crops and soil. Zhang et al. (2022) demonstrated that combining nitrification inhibitors with deep N fertilizer placement reduces both ammonia and nitrous oxide emissions. Future research should focus on combined optimization practices to maximize both economic and environmental benefits.
4.4 Effects of soil and meteorological factors on N losses
N losses under organic-inorganic fertilization were significantly influenced by meteorological factors (P< 0.05), with annual temperature positively correlated with N2O and NH3 emissions (Supplementary Tables S3, S4). Warmer climates enhance microbial nitrification-denitrification processes, increasing N2O emissions (Barnard et al., 2005). Higher temperatures also promote urea hydrolysis and NH4+ conversion to NH3, leading to greater NH3 volatilization (Koivunen and Horwath, 2004). However, N2O emissions do not increase linearly with temperature (Smith, 1997). Under warm and humid conditions, organic carbon from manure enhances denitrification, reducing N2O emissions and increasing N2 production (Das and Adhya, 2014). Annual rainfall was positively correlated with N runoff but negatively with NH3 volatilization and N leaching (Supplementary Tables S3, S4). Heavy rainfall after fertilization promotes N diffusion and reduces NH3 volatilization by mitigating the pH increase in surface soil (Liu X. et al., 2020). N fertilizer application rates, soil depth, irrigation, and ridge height also influence N losses through water leaching in paddy fields. As soil depth increases, the effect of N fertilizer rate on leaching diminishes, with higher total N concentrations found in the infiltration water 40 cm from the surface (Zhao et al., 2012). The lack of a standard for soil depth in leaching studies may explain the discrepancies in our findings.
Soil pH is a key factor in N losses, but no significant correlation was found in our analysis (Supplementary Tables S3-S5). Meta-analyses indicate that fertilizing in acidic (pH< 6) or alkaline (pH > 7) soils significantly increases N losses (Supplementary Tables S6, S7). Acidic soils tend to have more abundant N2O-producing fungal communities, resulting in higher N2O emissions (Chen et al., 2015). Our study confirmed that N2O emissions were highest in soils with pH< 6 (Supplementary Table S6). High pH also promotes ammonia volatilization by increasing the concentration of OH- in soil, which enhances the NH4+ and OH- reaction, leading to more NH3 volatilization. Surface water temperature increases more significantly than soil pH, influencing NH3 volatilization (Zhu, 1994). Increasing SOC reduces N runoff and leaching caused by fertilizer application, with SOC negatively correlated with N losses (P< 0.05) across all N fertilization optimization practices (Supplementary Tables S3-S5). Higher SOC improves soil water retention, boosting crop yield and N uptake, thereby reducing N losses (Wei et al., 2021). Higher cation exchange capacity linked to increased SOC also reduces NH4+ loss and improves NO3- retention (Blanco-Canqui and Lal, 2009; Bouwman et al., 2002). Our study found that three N fertilization optimization practices positively impacted yield and NUE in various soil and meteorological environments while reducing N losses (Supplementary Tables S6-S12). These practices enhance efficiency and reduce emissions, though emission reduction strategies should be adjusted based on specific crop production goals.
4.5 Limitations and perspectives
Although various N fertilizer management strategies and N loss pathways were considered in this study, further analysis, such as organic fertilizer application methods, types of inorganic N fertilizers, and rice crop rotation systems, remains limited due to data constraints. Due to the limitation of data, there are some uncertainties in this study. Additionally, our research primarily focuses on the middle and lower reaches of the Yangtze River. In the future, a long-term field measurement of multiple N losses, especially N water losses, should be conducted in the same agroecological region for different crop rotation systems, so as to form a N loss database suitable for regional soil-climate-crop system in China, which can provide basis for N precision management.
5 Conclusion
Through meta-analysis, the effects of optimized N management practices on N losses and crop productivity were quantified. Compared with the conventional field management practices, the optimized N fertilization management practices significantly reduced N losses and increased crop yields. The impact of optimal N fertilizer management on yield and N emissions was observably affected by N application rate and fertilization application frequency. Twice fertilization was recommended among the three N fertilizer optimization management measures. The N fertilizer management strategy combining organic and inorganic fertilizers can reduce N loss while maintain yield (SR< 0.3, N fertilizer application rate of 100 ≤ N< 200 kg/ha). The best strategy for enhanced-efficiency fertilizers is to apply SRF with a concentration of 100 ≤ N< 200 kg/ha.
Data availability statement
The original contributions presented in the study are included in the article/Supplementary Material. Further inquiries can be directed to the corresponding author.
Author contributions
YL: Investigation, Methodology, Validation, Writing – original draft, Writing – review & editing. ZF: Supervision, Validation, Writing – review & editing. WW: Supervision, Validation, Writing – review & editing. JZ: Supervision, Validation, Writing – review & editing. QC: Data curation, Funding acquisition, Resources, Supervision, Validation, Writing – review & editing. YT: Data curation, Funding acquisition, Resources, Supervision, Validation, Writing – review & editing. YZ: Data curation, Funding acquisition, Resources, Supervision, Writing – review & editing. WC: Data curation, Funding acquisition, Resources, Supervision, Validation, Writing – review & editing. XL: Conceptualization, Data curation, Formal Analysis, Funding acquisition, Investigation, Methodology, Project administration, Resources, Software, Supervision, Validation, Visualization, Writing – review & editing.
Funding
The author(s) declare that financial support was received for the research and/or publication of this article. This work was supported by the National Key Research and Development Program of China (No.2023YFD1701002, 2023YFD1701005, 2022YFD2301402), the Agricultural Science and Technology Innovation Program of Shanghai (I2023005), the Key Agricultural Technology Collaborative Promotion of Jiangsu (2024ZYXT013).
Conflict of interest
The authors declare that the research was conducted in the absence of any commercial or financial relationships that could be construed as a potential conflict of interest.
Generative AI statement
The author(s) declare that no Generative AI was used in the creation of this manuscript.
Publisher’s note
All claims expressed in this article are solely those of the authors and do not necessarily represent those of their affiliated organizations, or those of the publisher, the editors and the reviewers. Any product that may be evaluated in this article, or claim that may be made by its manufacturer, is not guaranteed or endorsed by the publisher.
Supplementary material
The Supplementary Material for this article can be found online at: https://www.frontiersin.org/articles/10.3389/fpls.2025.1485144/full#supplementary-material
References
Angela, H. (2004). The plastic plant: root responses to heterogeneous supplies of nutrients. New Phytol. 1, 9–24. doi: 10.1111/j.1469-8137.2004.01015.x
Bai, Z., Huang, J., Zhu, L., Cao, X., Zhu, C., Zhong, C., et al. (2020). Effects of N application strategies on N leaching loss in paddy soil and N use characteristics in different super hybrid rice cultivars. Paddy Water Environ. 18, 27–41. doi: 10.1007/s10333-019-00762-x
Barnard, R., Leadley, P. W., Hungate, B. A. (2005). Global change, nitrification, and denitrification: A review. Global Biogeochem Cy 19, GB1009. doi: 10.1029/2004GB002282
Beauchamp, E. G., Trevorst, J. T., Paul., J. W. (1989). Carbon sources for bacterial denitrification. Soil Sci. 10, 113–142. doi: 10.1007/978-1-4613-8847-0_3
Behera, S. N., Sharma, M., Aneja, V. P., Balasubramanian, R. (2013). Ammonia in the atmosphere: a review on emission sources, atmospheric chemistry and deposition on terrestrial bodies. Environ. Sci. pollut. Res. Int. 20, 8092–8131. doi: 10.1007/s11356-013-2051-9
Blanco-Canqui, H., Lal, R. (2009). Crop residue removal impacts on soil productivity and environmental quality. CRC Crit. Rev. Plant Sci. 28, 139–163. doi: 10.1080/07352680902776507
Bouwman, A. F., Boumans, L. J. M., Batjes, N. H. (2002). Estimation of global NH3 volatilization loss from synthetic fertilizers and animal manure applied to arable lands and grasslands. Global Biogeochem Cy. 2, 1024. doi: 10.1029/2000GB001389
Cao, Y., Sun, H., Zhang, J., Chen, G., Zhu, H., Zhou, S., et al. (2018). Effects of wheat straw addition on dynamics and fate of N applied to paddy soils. Soil Tillage Res. 178, 92–98. doi: 10.1016/j.still.2017.12.023
Cao, Y., Tian, Y., Yin, B., Zhu, Z. (2014). Improving agronomic practices to reduce nitrate leaching from the rice-wheat rotation system. Agric. Ecosyst. Environ. 195, 61–67. doi: 10.1016/j.agee.2014.05.020
Chantigny, M. H., Rochette, P., Angers, D. A. (2001). Short-term C and N dynamics in a soil amended with pig slurry and barley straw: a field experiment. Can. J. Soil Sci. 81, 131–137. doi: 10.4141/S00-046
Chen, Z., Chen, F., Zhang, H., Liu, S. (2016). Effects of N application rates on net annual global warming potential and greenhouse gas intensity in double-rice cropping systems of the southern China. Environ. Sci. pollut. 23, 24781–24795. doi: 10.1007/s11356-016-7455-x
Chen, H., Mothapo, N. V., Shi, W. (2015). Soil moisture and pH control relative contributions of fungi and bacteria to N2O production. Microb. Ecol. 69, 180–191. doi: 10.1007/s00248-014-0488-0
China, N.B.O.S (2019). China Statistical Yearbook-2019, No.38 (Beijing: China Statistical Publishing House). Available at: https://www.stats.gov.cn/sj/ndsj/2019/indexeh.htm (Accessed December 01, 2019).
Curtis, P. S., Wang, X. (1998). A meta-analysis of elevated CO2 effects on woody plant mass, form, and physiology. Oecologia 113, 229–313. doi: 10.1007//s004420050381
Das, S., Adhya, T. K. (2014). Effect of combine application of organic manure and inorganic fertilizer on methane and nitrous oxide emissions from a tropical flooded soil planted to rice. Geoderma. 213, 185–192. doi: 10.1016/j.geoderma.2013.08.011
Di, H. J., Cameron, K. C. (2002). Nitrate leaching in temperate agroecosystems: sources, factors and mitigating strategies. Nutr. Cycl Agroecosyst. 64, 237–256. doi: 10.1023/A:1021471531188
Ding, W., Xu, X., He, P., Ullah, S., Zhang, J., Cui, Z., et al. (2018). Improving yield and N use efficiency through alternative fertilization options for rice in China: A meta-analysis. Field Crops Res. 227, 11–18. doi: 10.1016/j.fcr.2018.08.001
Du, J. J., Wu, Y. L., Tian, J. L., Wang, Q. Y., Cui, D. Y. (2007). Effect of several controlled/slow-release fertilizers on decreasing ammonia volatilization and N leaching. J. Soil Water Conserv. 21, 49–52. doi: 10.13870/j.cnki.stbcxb.2007.02.013
Fan, D., He, W., Smith, W. N., Drury, C. F., Jiang, R., Grant, B. B., et al. (2022). Global evaluation of inhibitor impacts on ammonia and nitrous oxide emissions from agricultural soils: A meta-analysis. Glob Chang Biol. 28, 5121–5141. doi: 10.1111/gcb.16294
Geng, J., Sun, Y., Zhang, M., Li, C., Yang, Y., Liu, Z., et al. (2015). Long-term effects of controlled release urea application on crop yields and soil fertility under rice-oilseed rape rotation system. Field Crops Res. 184, 65–73. doi: 10.1016/j.fcr.2015.09.003
He, T., Yuan, J., Xiang, J., Lin, Y., Luo, J., Lindsey, S., et al. (2022). Combined biochar and double inhibitor application offsets NH3 and N2O emissions and mitigates N leaching in paddy fields. Environ. pollut. 292, 118344. doi: 10.1016/j.envpol.2021.118344
He, Y. T., Zhang, W. J., Xu, M. G., Tong, X. G., Sun, F. X., Wang, J. Z.., et al. (2015). Long-term combined chemical and manure fertilizations increase soil organic carbon and total N in aggregate fractions at three typical cropland soils in China. Sci. Total Environ. 532, 635–644. doi: 10.1016/j.scitotenv.2015.06.011
Hedged, L. V., Curevitch, J., Curtis, P. S. (1999). The meta-analysis of response ratios in experiment ecology. Ecology 80, 1150–1156. doi: 10.1890/0012-9658(1999)080[1150:TMAORR]2.0.CO;2
Hou, Q., Ni, Y., Huang, S., Zuo, T., Wang, J., Ni, W. (2023). Effects of substituting chemical fertilizers with manure on rice yield and soil labile N in paddy fields of China: A meta-analysis. Pedosphere. 33, 172–184. doi: 10.1016/j.pedsph.2022.09.003
Hou, H. J., Peng, S. Z., Xu, J. Z., Yang, S. H., Mao, Z. (2012). Seasonal variations of CH4, and N2O emissions in response to water management of paddy fields located in southeast China. Chemosphere 89, 884–892. doi: 10.1016/j.chemosphere.2012.04.066
Huang, S., Lv, W., Bloszies, S., Shi, Q., Pan, X., Zeng, Y. (2016). Effects of fertilizer management practices on yield-scaled ammonia emissions from croplands in China: A meta-analysis. Field Crops Res. 192, 118–125. doi: 10.1016/j.fcr.2016.04.023
IPCC (2013). Climate change 2013: the physical science basis: Working Group I contribution to the Fifth assessment report of the Intergovernmental Panel on Climate Change (Cambridge: Cambridge University). doi: 10.1017/CBO9781107415324
Jacinthe, P. A., Lal, R. (2003). N fertilization of wheat residue affecting nitrous oxide and methane emission from a central Ohio Luvisol. Biol. Fertil Soils. 37, 338–347. doi: 10.1007/s00374-003-0607-4
Janz, B., Havermann, F., Lashermes, G., Zuazo, P., Engelsberger, F., Torabi, S. M., et al. (2022). Effects of crop residue incorporation and properties on combined soil gaseous N2O, NO, and NH3 emissions-A laboratory-based measurement approach. Sci. Total Environ. 807, 151051. doi: 10.1016/j.scitotenv.2021.151051
Jin, X. (2018). Study on the regulation of soil acidification as affected by water N and carbon in farmland. Available online at: https://kns.cnki.net/kcms2/article/abstract?v=OHSidZhWKUnM08SVDLU9k1DpWT_6P7_PxqeRpWRT0w8HkrSsO579Nt3g00NeaDIU9HBxh6VY8tu7jPCianO4e1iVczaiT7nO96oL_HUxvHGjXJPMWIqwcykgR-jgnL6GL-zDUm7Uj8I=&uniplatform=NZKPT&language=CHS (Accessed December 02, 2018).
Ju, X., Gu, B. (2014). Status-quo, problem and trend of N fertilization in China. J. Plant Nutr. Fert 20, 783–795. doi: 10.11674/zwyf.2014.0401
Ju, X. T., Kou, C. L., Zhang, F. S., Christie, P. (2006). N balance and groundwater nitrate contamination: Comparison among three intensive cropping systems on the North China Plain. Environ. pollut. 143, 117–125. doi: 10.1016/j.envpol.2005.11.005
Ju, X. T., Xing, G. X., Chen, X. P., Zhang, F. S. (2009). Reducing environmental risk by improving N management in intensive Chinese agricultural systems. Proc. Natl. Acad. Sci. 106, 3041–3043. doi: 10.1073/pnas.0813417106
Kakar, K., Xuan, T. D., Noori, Z., Aryan, S., Gulab, G. (2020). Effects of organic and inorganic fertilizer application on growth, yield, and grain quality of rice. Agriculture. 10, 544. doi: 10.3390/agriculture10110544
Keeney, D. R. (1982). Nitrogen management for maximum efficiency and minimum pollution. Nitrogen in agricultural soils, 605–649. doi: 10.2134/agronmonogr22.c16
Kim, D., Saggar, S., Roudier, P. (2012). The effect of nitrification inhibitors on soil ammonia emissions in N managed soils: a meta-analysis. Nutr. Cycl Agroecosyst. 93, 51–64. doi: 10.1007/s10705-012-9498-9
Koivunen, M. E., Horwath, W. R. (2004). Effect of management history and temperature on the mineralization of methylene urea in soil. Nutr. Cycl Agroecosyst. 68, 25–35. doi: 10.1023/B:FRES.0000012232.56756.f0
Kumar, S., Patra, A., Singh, D., Purakayastha, T. (2014). Long-term chemical fertilization along with farmyard manure enhances resistance and resilience of soil microbial activity against heat stress. J. Agron. Crop Sci. 200, 156–162. doi: 10.1111/jac.12050
Lan, T., Li, M., Han, Y., Deng, O., Tang, X., Luo, L., et al. (2020). How are annual CH4, N2O, and NO emissions from rice-wheat system affected by N fertilizer rate and type? Appl. Soil Ecol. 150, 103469. doi: 10.1016/j.apsoil.2019.103469
Lan, T., Zhang, H., Han, Y., Deng, O., Tang, X., Luo, L., et al. (2021). Regulating CH4, N2O, and NO emissions from an alkaline paddy field under rice-wheat rotation with controlled release N fertilizer. Environ. Sci. pollut. Res. Int. 28, 18246–18259. doi: 10.1007/s11356-020-11846-1
Li, P., Lu, J., Wang, Y., Wang, S., Hussain, S., Ren, T., et al. (2018). N losses, use efficiency, and productivity of early rice under controlled-release urea. Agric. Ecosyst. Environ. 251, 78–87. doi: 10.1016/j.agee.2017.09.020
Li, Z., Reichel, R., Xu, Z., Vereecken, H., Brüggemann, N. (2021). Return of crop residues to arable land stimulates N2O emission but mitigates NO3- leaching: a meta-analysis. Agron. Sustain Dev. 41, 66. doi: 10.1007/s13593-021-00715-x
Liu, X., Chen, L., Hua, Z., Mei, S., Wang, P., Wang, S. (2020). Comparing ammonia volatilization between conventional and slow-release N fertilizers in paddy fields in the Taihu Lake region. Environ. Sci. pollut. Res. Int. 27, 8386–8394. doi: 10.1007/s11356-019-07536-2
Liu, T. Q., Fan, D. J., Zhang, X. X., Chen, J., Li, C. F., Cao, C. G. (2015). Deep placement of N fertilizers reduces ammonia volatilization and increases N utilization efficiency in no-tillage paddy fields in central China. Field Crops Res. 184, 80–90. doi: 10.1016/j.fcr.2015.09.011
Liu, J., Hou, H., Sheng, R., Chen, Z., Zhu, Y., Qin, H., et al. (2012). Denitrifying communities differentially respond to flooding drying cycles in paddy soils. Appl. Soil Ecol. 62, 155–162. doi: 10.1016/j.apsoil.2012.06.010
Liu, T. Q., Li, S. H., Guo, L. G., Cao, C. G., Li, C. F., Zhai, Z. B., et al. (2020). Advantages of N fertilizer deep placement in greenhouse gas emissions and net ecosystem economic benefits from no-tillage paddy fields. J. Clean Prod. 263, 121322. doi: 10.1016/j.jclepro.2020.121322
Liu, B., Mørkved, P. T., Frostegård, Å., Bakken, L. R. (2010). Denitrification gene pools, transcription and kinetics of NO, N2O and N2 production as affected by soil pH. FEMS Microbiol. Ecol. 72, 407–417. doi: 10.1111/j.1574-6941.2010.00856.x
Liu, C., Ren, D., Liu, H., Zhang, Y., Wang, L., Li, Z., et al. (2022). Optimizing N management diminished reactive N loss and acquired optimal net ecosystem economic benefit in a wheat-maize rotation system. J. Clean Prod. 331, 129964. doi: 10.1016/j.jclepro.2021.129964
Liu, B., Wang, X., Ma, L., Chadwick, D., Chen, X. (2021). Combined applications of organic and synthetic N fertilizers for improving crop yield and reducing reactive N losses from China’s vegetable systems: A meta-analysis. Environ. pollut. 269, 116143. doi: 10.1016/j.envpol.2020.116143
Liu, X., Wang, H., Zhou, J., Hu, F., Zhu, D., Chen, Z., et al. (2016). Effect of N fertilization pattern on rice yield, N use efficiency and fertilizer-N fate in the yangtze river basin, China. PloS One 11, e166002. doi: 10.1371/journal.pone.0166002
Luo, Y., Hui, D., Zhang, D. (2006). Elevated CO2 stimulates net accumulations of carbon and N in land ecosystems: a meta-analysis. Ecology. 1, 53–63. doi: 10.1890/04-1724
Ma, Y., Liu, D. L., Schwenke, G., Yang, B. (2019). The global warming potential of straw-return can be reduced by application of straw-decomposing microbial inoculants and biochar in rice-wheat production systems. Environ. pollut. 252, 835–845. doi: 10.1016/j.envpol.2019.06.006
Meng, X., Li, Y., Yao, H., Wang, J., Dai, F., Wu, Y. (2020). Nitrification and urease inhibitors improve rice N uptake and prevent denitrification in alkaline paddy soil. Agric. Ecosyst. Environ. Appl. Soil Ecol. 154, 103665. doi: 10.1016/j.apsoil.2020.103665
Meng, L., Zhang, X. L., Jiang, X. F., Wang, Q. J., Huang, Q. W., Xu, C. Y., et al (2009). Effects of partial mineral nitrogen substitution by organic fertilizer nitrogen on the yields of rice grains and their proper substitution rate. Sci. Agric. Sin. 42, 532–542. doi: 10.3864/j.issn.0578-1752.2009.02.019
Mohanty, S., Anjani, A., Kumar, A., Tripathi, R., Shahid, M., Bhattacharyya, P., et al. (2013). Carbon and N mineralization kinetics in soil of rice-rice system under long term application of chemical fertilizers and farmyard manure. Eur. J. Soil Biol. 58, 113–121. doi: 10.1016/j.ejsobi.2013.07.004
Pan, G., Zhou, P., Li, Z., Smith, P., Li, L., Qiu, D., et al. (2009). Combined inorganic/organic fertilization enhances N efficiency and increases rice productivity through organic carbon accumulation in a rice paddy from the Tai Lake region, China. Agri Ecosyst. Environ. 131, 274–280. doi: 10.1016/j.agee.2009.01.020
Qiao, J., Zhao, D., Zhou, W., Yan, T. M., Yang, L. Z. (2021). Sustained rice yields and decreased N runoff in a rice-wheat cropping system by replacing wheat with Chinese milk vetch and sharply reducing fertilizer use. Environ. Pollut. 288, 117722. doi: 10.1016/j.envpol.2021.117722
Ren, F., Sun, N., Misselbrook, T., Wu, L., Xu, M., Zhang, F., et al. (2022). Responses of crop productivity and reactive N losses to the application of animal manure to China’s main crops: A meta-analysis. Sci. Total Environ. 850, 158064. doi: 10.1016/j.scitotenv.2022.158064
Röös, E., Mie, A., Wivstad, M., Salomon, E., Johansson, B., Gunnarsson, S., et al. (2018). Risks and opportunities of increasing yields in organic farming. A review. Agron. Sustain. Dev. 38, 14. doi: 10.1007/s13593-018-0489-3
Seufert, V., Ramankutty, N., Foley, J. (2012). Comparing the yields of organic and conventional agriculture. Nature 485, 229–232. doi: 10.1038/nature11069
Shen, J. B., Li, C. J., Mi, G. H., Li, L., Yuan, L. X., Jiang, R. F., et al. (2013). Maximizing root/rhizosphere efficiency to improve crop productivity and nutrient use efficiency in intensive agriculture of China. J. Exp. Bot. 64 (5), 1181–1192. doi: 10.1093/jxb/ers342
Shi, X., Hu, K., Batchelor, W. D., Liang, H., Wu, Y., Wang, Q., et al. (2020). Exploring optimal N management strategies to mitigate N losses from paddy soil in the middle reaches of the Yangtze River. Agric. Water Manage. 228, 105877. doi: 10.1016/j.agwat.2019.105877
Singh, T. B., Ali, A., Prasad, M., Yadav, A., Shrivastav, P., Goyal, D., et al. (2020). Role of organic fertilizers in improving soil fertility. Springer Int. Publishing Cham pp, 61–77. doi: 10.1007/978-3-030-41552-5_3
Smith, K. (1997). The potential for feedback effects induced by global warming on emissions of nitrous oxide by soils. Glob Chang Biol. 3, 327–338. doi: 10.1046/j.1365-2486.1997.00100.x
Srinivasarao, C., Venkateswarlu, B., Lal, R., Singh, A., Kundu, S., Vittal, K., et al. (2014). Long-term mauring and fertilizer effect of soil organic carbon stocks under pearl millet-cluster bean-caster rotation in western India. Land Degrad Dev. 25, 173–183. doi: 10.1002/ldr.1158
Stewart, B. A., Carter, M. R. (1996). Structure and organic matter storage in agricultural soils (Boca Raton, FL: Lewis Publishers). doi: 10.1201/9781003075561
Su, W., Liu, B., Liu, X., Li, X. K., Ren, T., Cong, R., et al. (2015). Effect of depth of fertilizer banded-placement on growth, nutrient uptake and yield of oilseed rape (Brassica napus L). Eur. J. Agro. 62, 38–45. doi: 10.1016/J.EJA.2014.09.002
Sun, L., Wu, Z., Ma, Y., Liu, Y., Xiong, Z. (2018). Ammonia volatilization and atmospheric N deposition following straw and urea application from a rice-wheat rotation in southeastern China. Atmos Environ. 181, 97–105. doi: 10.1016/j.atmosenv.2018.02.050
Tang, Y., Li, X., Shen, W., Duan, Z. (2018). Effect of the slow-release N fertilizer oxamide on ammonia volatilization and N use efficiency in paddy soil. Agronomy. 8, 53. doi: 10.3390/agronomy8040053
Tian, F. X., Ji, X. H., Guan, D., Xie, Y. H., He, S. H., Liu, Z. B. (2020). Advances of research on N inhibitors. Hybrid Rice. 35, 7–13. doi: 10.16267/j.cnki.1005-3956.20191210.290
Trinh, T., Kushaari, K., Shuib, A., Ismail, L., Azeem, B. (2015). Modelling the release of N from controlled release fertiliser: constant and decay release. Biosyst. Eng. 130, 34–42. doi: 10.1016/j.biosystemseng.2014.12.004
Vengadaramana, A., Jashothan, J. P. T. (2012). Effect of organic fertilizers on the water holding capacity of soil in different terrains of Jaffna peninsula in Sri Lanka. J. Nat. Prod. Plant Resour 4, 500–503. Available at: https://www.researchgate.net/publication/259900746 (Accessed January 01, 2012).
Wang, B., Li, Y. E., Wan, Y., Qin, X., Gao, Q., Liu, S., et al. (2016). Modifying N fertilizer practices can reduce greenhouse gas emissions from a Chinese double rice cropping system. Agric. Ecosyst. Environ. 215, 100–109. doi: 10.1016/j.agee.2015.09.008
Wang, J., Wang, D., Zhang, G., Wang, C. (2012). Effect of wheat straw application on ammonia volatilization from urea applied to a paddy field. Nutr. Cycl Agroecosyst. 94, 73–84. doi: 10.1007/s10705-012-9527-8
Wang, L., Zhao, X., Gao, J., Butterly, C. R., Chen, Q., Liu, M. (2019). Effects of fertilizer types on N and phosphorous loss from rice-wheat rotation system in the Taihu Lake region of China. Agric. Ecosyst. Environ. 285, 106605. doi: 10.1016/j.agee.2019.106605
Wang, X., Zhu, J., Gao, R., Yasukazu, H., Feng, K. (2007). N cycling and losses under rice-wheat rotations with coated urea and urea in the Taihu Lake region. Pedosphere. 17, 62–69. doi: 10.1016/S1002-0160(07)60008-3
Wei, Z., Hoffland, E., Zhuang, M., Hellegers, P., Cui, Z. (2021). Organic inputs to reduce N export via leaching and runoff: A global meta-analysis. Environ. pollut. 291, 118176. doi: 10.1016/j.envpol.2021.118176
Wu, X., Wang, W., Xie, X., Hou, H., Yin, C. (2018). Response of N2O emission to straw retention under two contrasting paddy water conditions: a field experiment over rice-rice-fallow rotation. Paddy Water Environ. 16, 199–205. doi: 10.1007/s10333-017-0621-z
Xia, Y., Chen, X., Zheng, S., Gunina, A., Ning, Z., Hu, Y., et al. (2021). Manure application accumulates more N in paddy soils than rice straw but less from fungal necromass. Agric. Ecosyst. Environ. 319, 107575. doi: 10.1016/j.agee.2021.107575
Xia, L., Li, X., Ma, Q., Lam, S. K., Wolf, B., Kiese, R., et al. (2020). Simultaneous quantification of N2, NH3 and N2O emissions from a flooded paddy field under different N fertilization regimes. Glob Chang Biol. 26, 2292–2303. doi: 10.1111/gcb.14958
Xu, S., Hou, P., Xue, L., Wang, S., Yang, L. (2017). Treated domestic sewage irrigation significantly decreased the CH4, N2O and NH3 emissions from paddy fields with straw incorporation. Atmos Environ (1994). . 169, 1–10. doi: 10.1016/j.atmosenv.2017.09.009
Xue, L., Yu, Y., Yang, L. (2011). N balance and environmental impact of paddy field under different N management methods in Taihu Lake region. J. Environ. Sci. (China). 32, 1133–1138. doi: 10.13227/j.hjkx.2011.04.010
Yang, S., Peng, S., Xu, J., He, Y., Wang, Y. (2015). Effects of water saving irrigation and controlled release N fertilizer managements on N losses from paddy fields. Paddy Water Environ. 13, 71–80. doi: 10.1007/s10333-013-0408-9
Yang, S. H., Peng, S. Z., Xu, J. Z., Luo, Y. F., Li, D. X. (2012). Methane and nitrous oxide emissions from paddy field as affected by water-saving irrigation. Phy Chem. Earth. 53–54, 30–37. doi: 10.1016/j.pce.2011.08.020
Yao, Y., Zhang, M., Tian, Y., Zhao, M., Zhang, B., Zhao, M., et al. (2018). Urea deep placement for minimizing NH3 loss in an intensive rice cropping system. Field Crops Res. 218, 254–266. doi: 10.1016/j.fcr.2017.03.013
Ye, G., Lin, Y., Kuzyakov, Y., Liu, D., Luo, J., Lindsey, S., et al. (2019). Manure over crop residues increases soil organic matter but decreases microbial necromass relative contribution in upland Ultisols: Results of a 27-year field experiment. Soil Biol. Biochem. 134, 15–24. doi: 10.1016/j.soilbio.2019.03.018
Zhang, X., Davidson, E. A., Mauzerall, D. L., Searchinger, T. D., Dumas, P., Shen, Y. (2015). Managing N for sustainable development. Nature. 528, 51–59. doi: 10.1038/nature15743
Zhang, X., Fang, Q., Zhang, T., Ma, W., Velthof, G. L., Hou, Y., et al. (2020). Benefits and trade-offs of replacing synthetic fertilizers by animal manures in crop production in China: A meta-analysis. Glob Chang Biol. 26, 888–900. doi: 10.1111/gcb.14826
Zhang, C., Song, X., Zhang, Y., Wang, D., Rees, R. M., Ju, X. (2022). Using nitrification inhibitors and deep placement to tackle the trade-offs between NH3 and N2O emissions in global croplands. Glob Chang Biol. 28, 4409–4422. doi: 10.1111/gcb.16198
Zhang, M., Tian, Y., Zhao, M., Yin, B., Zhu, Z. (2017). The assessment of nitrate leaching in a rice-wheat rotation system using an improved agronomic practice aimed to increase rice crop yields. Agric. Ecosyst. Environ. 241, 100–109. doi: 10.1016/j.agee.2017.03.002
Zhang, Q. L., Zhang, M., Tian, W. B. (2001). Leaching characteristics of controlled release and common N fertilizers and their effects on soil and ground water quality. Soil Environ. Sci. 02), 98–103. doi: 10.16258/j.cnki.1674-5906.2001.02.004
Zhao, X., Zhou, Y., Min, J., Wang, S., Shi, W., Xing, G. (2012). N runoff dominates water N pollution from rice-wheat rotation in the Taihu Lake Region of China. Agric. Ecosyst. Environ. 156, 1–11. doi: 10.1016/j.agee.2012.04.024
Zheng, S. X., Liu, D. L., Nie., J., Dai, P. A., Xiao, J. (2004). Fate and recovery efficiency of controlled release N fertilizer in flooding paddy soil. J. Plant Nutr. Fert. 10, 137–142. doi: 10.11674/zwyf.2004.0205
Zhong, Y., Wang, X., Yang, J., Zhao, X. (2017). Tracing the fate of N with 15N isotope considering suitable fertilizer rate related to yield and environment impacts in paddy field. Paddy Water Environ. 15, 943–949. doi: 10.1007/s10333-017-0606-y
Zhong, X., Zhou, X., Fei, J., Huang, Y., Wang, G., Kang, X., et al. (2021). Reducing ammonia volatilization and increasing N use efficiency in machine-transplanted rice with side-deep fertilization in a double-cropping rice system in Southern China. Agric. Ecosyst. Environ. 306, 107183. doi: 10.1016/j.agee.2020.107183
Zhou, Z., Gan, Z., Shangguan, Z., Zhang, F. (2013). Effects of long-term repeated mineral and organic fertilizer applications on soil organic carbon and total N in a semi-arid cropland. Eur. J. Agron. 45, 20–26. doi: 10.1016/j.eja.2012.11.002
Zhou, R., Liu, Y., Dungait, J. A. J., Kumar, A., Wang, J., Tiemann, L. K., et al. (2023). Microbial necromass in cropland soils: A global meta-analysis of management effects. Glob Chang Biol. 29, 1998–2014. doi: 10.1111/gcb.16613
Zhu, Z. (1994). N transformation and rational application N fertilizer in paddy soil. Chemistry 9, 15–17. Available online at: https://kns.cnki.net/kcms2/article/abstract?v=Kk8bzUe9ukq3yJK59VajthP9IxLCWKi95YPnq5_6nB7vGppEbrPfpFulVpi1o4Y93SvTrzELs8NzxmzA0R8hSSHFb4MplXTkLkp0jJy6bk_IecKlD6IIGs9Js8KMCK5NUkMaVs2ZQEN0SvuEhrB4wegUs8FejzT7_jr9CV7NHyv61YRTDGhoT3cvAwHS8a6r&uniplatform=NZKPT&language=CHS (Accessed September 01, 1994).
Keywords: rice, N fertilizer management optimization, yield, active N emissions, meta-analysis
Citation: Liu Y, Fu Z, Wang W, Zhang J, Cao Q, Tian Y, Zhu Y, Cao W and Liu X (2025) Effects of N management optimization practices on rice productivity and N loss: a meta-analysis. Front. Plant Sci. 16:1485144. doi: 10.3389/fpls.2025.1485144
Received: 27 September 2024; Accepted: 25 February 2025;
Published: 22 April 2025.
Edited by:
Richard William Bell, Murdoch University, AustraliaReviewed by:
Peng Jiang, Sichuan Academy of Agricultural Sciences, ChinaHanxi Wang, Harbin Normal University, China
Copyright © 2025 Liu, Fu, Wang, Zhang, Cao, Tian, Zhu, Cao and Liu. This is an open-access article distributed under the terms of the Creative Commons Attribution License (CC BY). The use, distribution or reproduction in other forums is permitted, provided the original author(s) and the copyright owner(s) are credited and that the original publication in this journal is cited, in accordance with accepted academic practice. No use, distribution or reproduction is permitted which does not comply with these terms.
*Correspondence: Xiaojun Liu, bGl1eGpAbmphdS5lZHUuY24=