- 1Institute of Botany, Academy of Sciences of Uzbekistan, Tashkent, Uzbekistan
- 2Department of Biology Teaching Methodology, Jizzakh State Pedagogical University, Jizzakh, Uzbekistan
- 3College of Resources and Environment, Yunnan Agricultural University, Kunming, China
The study focused on analyzing the chloroplast genome structure and investigating the phylogenetic relationships among six species of the Cousinia genus. Within the Asteraceae family, the complete chloroplast genome sequences of six Cousinia species, ranging from 152,553 to 152,619 bp. The chloroplast genomes exhibit a characteristic quadripartite structure. The gene order is largely conserved across the genus, with an exception in the small single copy region, where a reverse orientation is observed in comparison to Cousinia thomsonii. A total of 131 genes were annotated, including 87 protein-coding genes, 36 tRNA genes, and 8 rRNA genes, with 18 genes showing duplication. Notably, 16 genes contain introns, with ycf3 and clpP carrying two introns each. Nucleotide diversity analysis revealed 412 polymorphic sites across 152,892 nucleotides in six Cousinia species. Higher nucleotide polymorphism levels were found in trnE-UUC – rpoB, trnL-UAA – trnF-GAA – ndhJ, rbcL, and ycf1 genomic regions, indicating possible genomic loci for species differentiation. Phylogenetic analysis using complete chloroplast genomes, demonstrated the genus Cousinia’s phylogenetic placement within the Cardueae tribe, forming distinct clades that align with its traditional sectional classification. The Arctiinae subtribe, containing Cousinia, forms a monophyletic group with Arctium lappa, while Saussureinae were found to be polyphyletic. The findings suggest that while morphological traits are valuable in taxonomy, they may provide limited resolution compared to the more comprehensive phylogenetic insights obtained from chloroplast genome analysis.
Introduction
Cousinia Cass. (Asteraceae, Cardueae) is a large genus, being the 50th largest genera among flowering plants. It is represented by approximately 671 species (Frodin, 2004; Ulukuş and Tugay, 2020; Uysal et al., 2022; POWO, 2025). The species of Cousinia are typically found in arid regions, with a preference for upland vegetation. They are mainly distributed in the mountainous areas of Turkey, Iran, Afghanistan and Central Asia (Knapp, 1987). Central Asia, home to ca. 260 species, is considered the most significant region for these plants (Tscherneva, 1993).
Cousinia was first described by Cassini (1827). de Candolle, (1824) listed 34 species of Cousinia, organized into three categories according to the morphology of their capitula. In an early effort to comprehensively classify the genus Cousinia, von Bunge (1865) categorized 126 species of Cousinia into 23 sections, primarily based on growth habit and capitular features, such as the texture of receptacular bristles, shape of receptacular bracts, corolla color, color of the anther tube, and whether or not hairs were present on the anther tube. Bunge’s classification served as a foundation for later studies, including Boissier’s Flora Orientalis (1875) and its supplement (1888), which documented 141 species of Cousinia organized into 14 sections. The next significant classification was by Winkler in 1892 (Winker, 1892), who emphasized capitulum morphology for taxonomy like Bunge’s classification. In a notable divergence, Kuntze (1891) proposed merging Cousinia with the genus Arctium L. Winkler’s work (1892, 1897) expanded to include 267 species divided into three subgenera (Oligochaete, Dichacantha, and Eucousinia). Bunge’s approach laid the groundwork for a more natural classification system as later elaborated by Rechinger (1953, 1972) and Tscherneva (1962, 1988). Rechinger’s Flora Iranica (1972) included over 350 species across 58 sections, addressing the flora of the Iranian Plateau, Turkmenistan, Afghanistan, and the mountainous regions of Pakistan. Similarly, Tscherneva’s treatment (1962, 1988) for the Flora of the USSR cataloged around 260 species in 50 sections, covering regions of Central Asia and the Caucasus. According to Sennikov (2010), the sections Alpinae Bunge (Carduncellus (Juz.) Rech. f.) and Subappendiculatae Tschern. were discovered to be varied in their involucre structure, anther appendage shape, and basal leaves. Consequently, the classification now includes three sections instead of two, with a new section, Tianschanicae Sennikov, being distinguished from Alpinae. Additionally, Cousinia knorringiae has been reassigned from the Alpinae section to the Subappendiculatae section, while Cousinia omissa and Cousinia subappendiculata have been moved from Subappendiculatae to Tianschanicae.
Molecular studies started at the end of the 1990s. The phylogeny of the Cardueae tribe, studied by Häffner and Hellwig (1999) using ITS sequence data and later supported by Garcia-Jacas et al. (2002) through ITS and matK data, demonstrated a close relationship among the genera Cousinia, Arctium, Saussurea, and Jurinea. The molecular analysis of the Arctium-Cousinia complex by Susanna et al. (2003) identified two main lineages: the Arctioid clade, which includes Arctium s.str. and certain Cousinia subgenera (Hypacanthodes, Cynaroides, Hypacanthium, and Schmalhausenia), and the Cousinioid clade, consisting of Cousinia subg. Cousinia. This classification was supported by subsequent studies (Susanna et al., 2006; López-Vinyallonga et al., 2009). In 2011, López-Vinyallonga et al. transferred all Arctioid species to Arctium based on analyses of ITS, trnL-trnF, and matK sequences. Mehregan and Kadereit (2009) investigated potential hybridization among 214 species of Cousinia and related genera, concluding that there was no evidence of hybridization between Cousinia s.str. and other clades within the Arctium-Cousinia complex, nor between annual and perennial species of Cousinia s.str. Atazadeh et al. (2021) focused on species delineation within the sections Cynaroideae and Platyacanthae, but their ITS-based phylogenetic tree did not separate these sections. Kalouti et al. (2022) examined the monophyly of Cousinia sect. Stenocephalae, along with sect. Albidae and Cousinia, finding that none of these sections are monophyletic based on ITS data.
While various studies have used genetic sequence data from nuclear ITS regions and short chloroplast markers to explore relationships within the genus (Häffner and Hellwig, 1999; Garcia-Jacas et al., 2002; Susanna et al., 2003, 2006; López-Vinyallonga et al., 2009, 2011; Mehregan and Kadereit, 2009; Atazadeh et al., 2021; Kalouti et al., 2022), none have focused on the complete structure of the chloroplast (cp) genome, and only one cp genome of Cousinia is currently available in GenBank (Accession No. PP525141).
To address these gaps in understanding the evolutionary relationships within Cousinia, we sequenced the cp genomes of six Cousinia species. These include Cousinia rhodantha Kult. (Southwestern Pamir-Alay), C. pseudodshizakensis Tschern. & Vved. (Northwestern Pamir-Alay), C. proxima Juz. (Gissar Range), C. subcandicans Tschern. (Gissar Range), C. orthacantha Tschern. (Southwestern Pamir-Alay, Afghanistan), and C. rotundifolia C.Winkl. (Pamir-Alay), exhibit highly restricted distributions (Tscherneva, 1962), emphasizing their endemism and conservation importance. These species play crucial ecological roles in their native mountainous habitats, contributing to soil stabilization, supporting pollinators, and maintaining local biodiversity.
The primary objectives of our study were: 1) to analyze the cp genome structures of Cousinia species, 2) to identify the most divergent regions that can aid in species differentiation, 3) to make phylogenetic inferences to determine the position of Cousinia.
Materials and methods
Plant materials
The study species represented three distinct sections within the genus Cousinia: sect. Alpinae (syn. Cousinia section Carduncellus) (Cousinia pseudodshizakensis, C. rhodantha and C. rotundifolia), sect. Homalochaete (C. subcandicans and C. proxima) and sect. Dichotomae (C. orthacantha collected in two populations). (Figure 1). All the species were collected from Uzbekistan. The herbarium specimens have been deposited at the National Herbarium of Uzbekistan (TASH) (Supplementary Table S1).
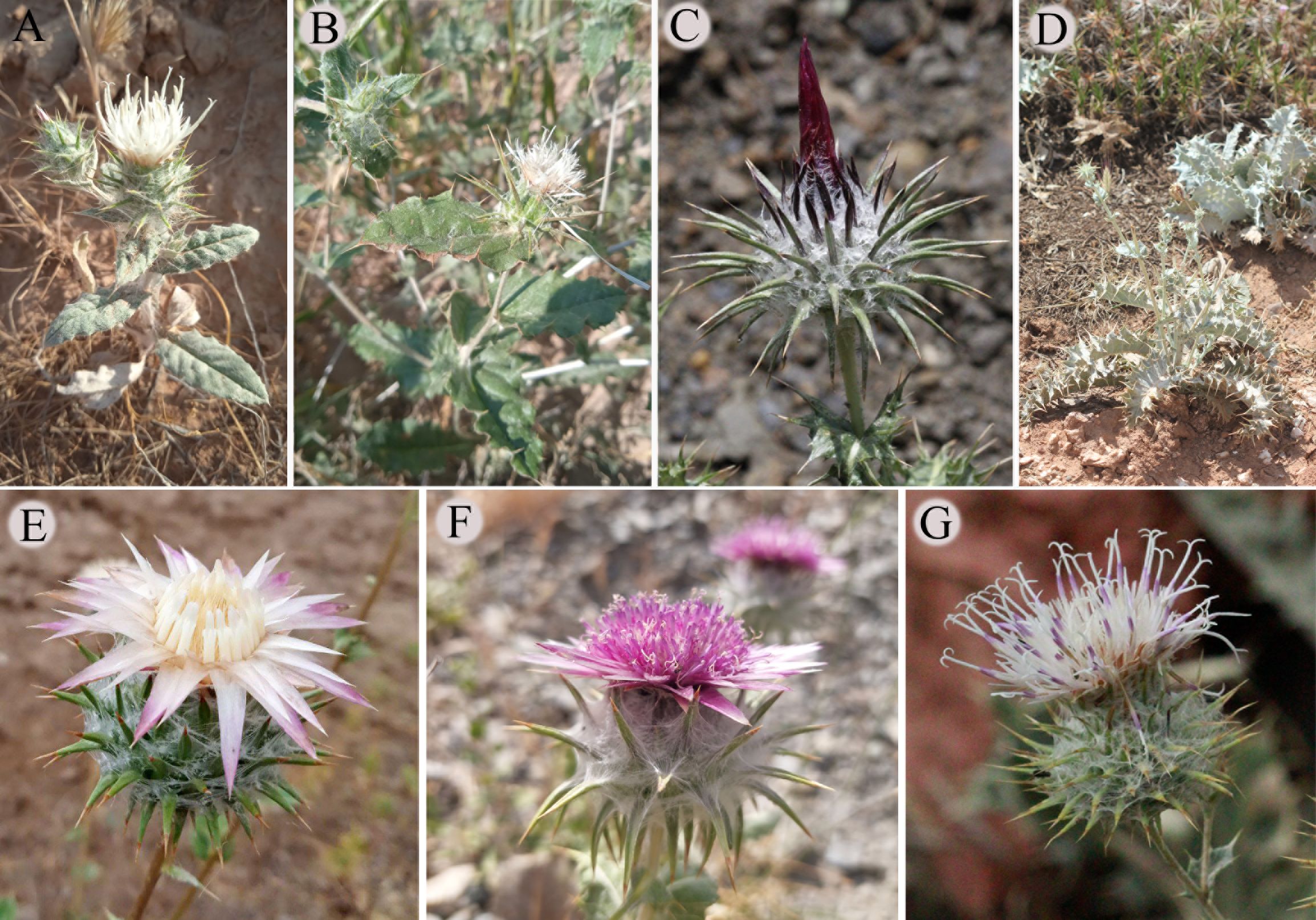
Figure 1. Collected species of Cousinia in this study. (A) Cousinia orthacantha (desert) (B) Cousinia orthacantha (agricultural area) (C) Cousinia rhodantha, (D) Cousinia subcandicans, (E) Cousinia rotundifolia, (F) Cousinia pseudodshizakensis, (G) Cousinia proxima.
Sequencing, assembly, and annotation
Genomic DNA was isolated from leaf samples using the DP305 Plant Genomic DNA kits from Tiangen, Beijing, China, following the provided protocol. For library preparation, the NEBNext® UltraTM DNA Library Prep Kit for Illumina from NEB, USA (Catalog: E7370L) was utilized, following the manufacturer’s recommendations. Index codes were incorporated into each sample. The genomic DNA underwent sonication to fragment it into pieces of approximately 350 base pairs. The resulting DNA fragments were subjected to end polishing, A-tailing, and ligated with full-length adapters suitable for Illumina sequencing. Subsequent PCR amplification was performed. The PCR products were purified using the AMPure XP system from Beverly, USA. The quality of the libraries was evaluated using the Agilent 5400 system from Agilent, USA, and their concentration was determined by QPCR (1.5 nM). The qualified libraries were combined and sequenced on Illumina platforms using the PE150 strategy at Novogene Bioinformatics Technology Co., Ltd in Beijing, China, according to the required library concentrations and data volume. In an investigation of six Cousinia species, paired-end sequencing produced between 7,890,409 reads (C. pseudodshizakensis) and 20,105,500 reads (C. subcandicans), with each read comprising 150 base pairs. Based on an estimated genome size of 150 kilobases, this corresponds to a sequencing depth ranging from approximately 7,890× to 20,105×. De novo assembly of the cp genomes for the six Cousinia species was performed using NOVOPlasty v4.3.4 (Dierckxsens et al., 2017). Clean paired-end reads in FASTQ format were used as input. During the time of our analysis, the cp genome sequence of Cousinia thomsonii (PP525141) was not available in the NCBI database. Consequently, Arctium lappa (NC042724) was chosen as a reference for assembly and annotation due to its availability and taxonomic placement within the Arctiinae subtribe. The assemblies produced circular cp genomes.
Gene annotation was carried out using Geneious v.9.0.2, with Arctium lappa set as the reference. Manual inspection was performed to verify the start and stop codons and the boundaries of introns and exons for protein-coding genes (Kearse et al., 2012).
The SRA (Sequence Read Archive) data associated with the PRJNA1124612 BioProject has been deposited in the GenBank database (Supplementary Table S2).
Genome structure analyses
The identification of cp simple sequence repeats (SSRs) was performed using the MIcroSAtellite (https://webblast.ipk-gatersleben.de/misa/) web tool (Beier et al., 2017). The search parameters were set to detect perfect mono-, di-, tri-, tetra-, penta-, and hexa nucleotide motifs with a minimum repeat count of 10, 5, 4, 3, 3, and 3, respectively. Additionally, the REPuter web tool (https://bibiserv.cebitec.uni-bielefeld.de/reputer) (Kurtz et al., 2001) was employed to identify various types of repeats, including forward, reverse, palindrome, and complement sequences, within the cp genomes. The repeat identification settings used were: a hamming distance of three, a minimum repeat size of 30 base pairs, and a maximum computed repeat size of 90 base pairs.
Genome comparison and nucleotide variation analysis
The OGDRAWv1.1 online tool (https://chlorobox.mpimp-golm.mpg.de/OGDraw.html) (Lohse et al., 2007) was employed for the graphical representation of the cp genome. The nucleotide variability (Pi) values were obtained with the DnaSP v.6.12.03 software (Rozas et al., 2017). A window length of 1500 base pairs and a step size of 700 base pairs were used for this analysis. The IR region boundaries, as well as the genomic regions, were visualized and analyzed using the IRscope online analysis tool (https://irscope.shinyapps.io/irapp/) (Amiryousefi et al., 2018).
Phylogenetic analyses
The newly sequenced cp genomes and nuclear ribosomal DNA (nrDNA) ITS sequences of six Cousinia species, along with 39 plastome sequences and 76 nrDNA ITS sequences from the Cardueae tribe obtained from NCBI (Supplementary Table S3), were utilized to construct a phylogenetic tree. Famatinanthus decussatus (Hieron.) Ariza & S.E. Freire was designated as the outgroup (Herrando-Moraira et al., 2019). The MAFFT software (Katoh and Standley, 2013) was used for the alignment of complete cp genomes. Bayesian analyses were performed using MrBayes v.3.2 (Ronquist et al., 2011). The best-fitting models of nucleotide substitutions, selected based on the Akaike information criterion (AIC) in jModelTest v.2.1.4 (Darriba et al., 2012), were employed in this analysis. Maximum likelihood (ML) analyses were carried out using RAxML v.8.0 (Stamatakis, 2014) with the best-fit GTR + G model and 1000 bootstrap replicates.
Microscopic examination of anther appendages
We investigated the morphology of anther appendages in 20 species from three sections (Alpinae, Homalochaete, Dichotomae) of the Cousinia genus using microscopy. Specimens were sourced from the National Herbarium of Uzbekistan and the Herbarium of the Institute of Botany, Plant Physiology, and Genetics at the National Academy of Sciences of Tajikistan.
Results
Chloroplast genome features
We obtained the full cp genome sequences of the six Cousinia species ranged from 152,553 to 152,619 bp (Figure 2). The cp genome presented a typical quadripartite structure including one LSC region, one SSC region, and a pair of IR regions, respectively. The gene order within the genus was generally conserved, except for the SSC region, which exhibited a reverse orientation in the six Cousinia species, in contrast to the forward orientation observed in C. thomsonii. The GC content of the seven Cousinia species was nearly identical (Table 1).
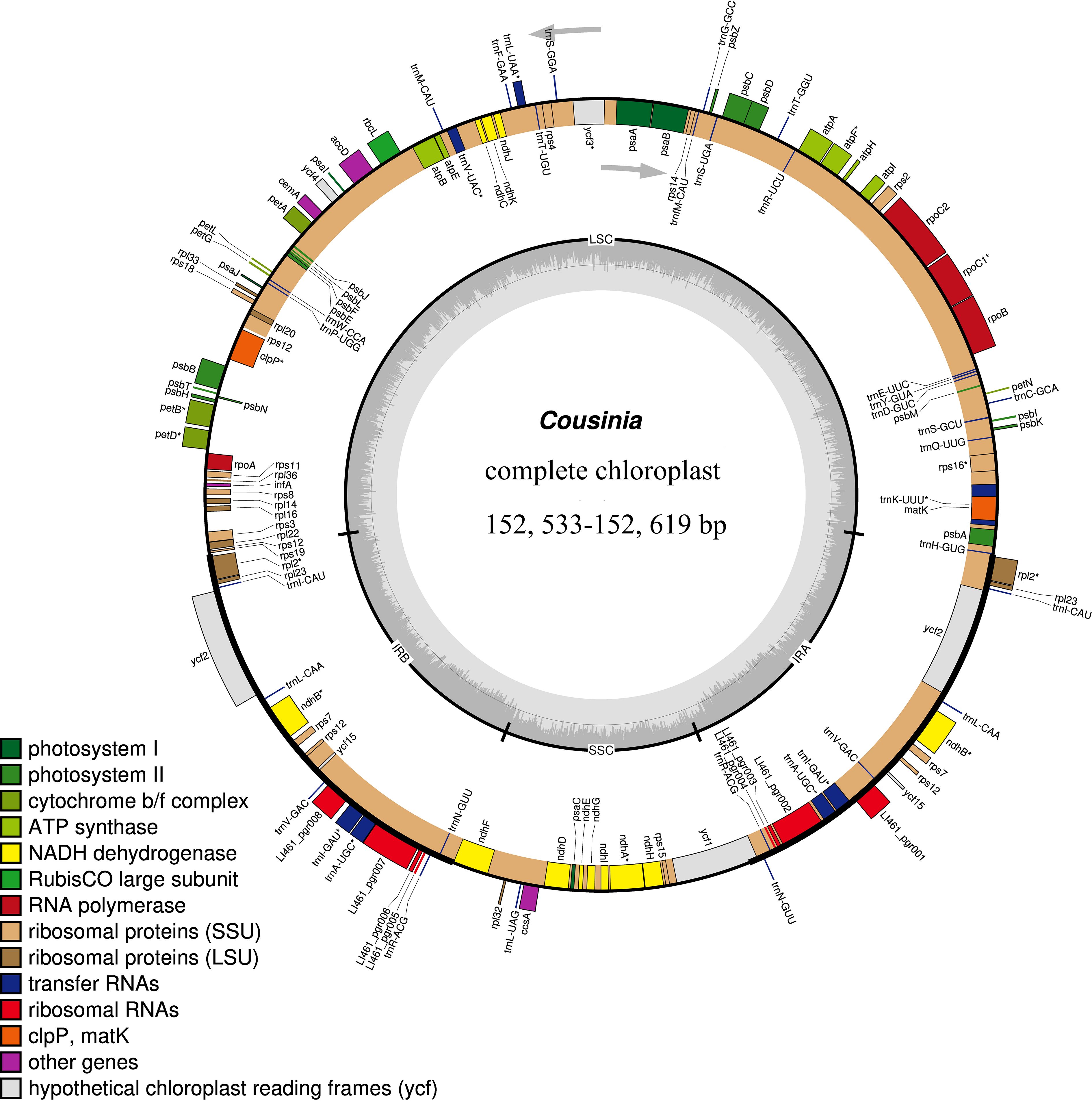
Figure 2. Gene map of the Cousinia chloroplast genome. Genes shown outside the outer circle are transcribed clockwise, and those insides are transcribed counterclockwise. Genes are color coded according to different functional groups. The darker gray in the inner circle indicates the GC content, and the lighter gray indicates the AT content. The inner circle also shows that the chloroplast genome contains two copies of inverted repeats (IRA and IRB), a large single-copy (LSC) region and a small single-copy (SSC)region.
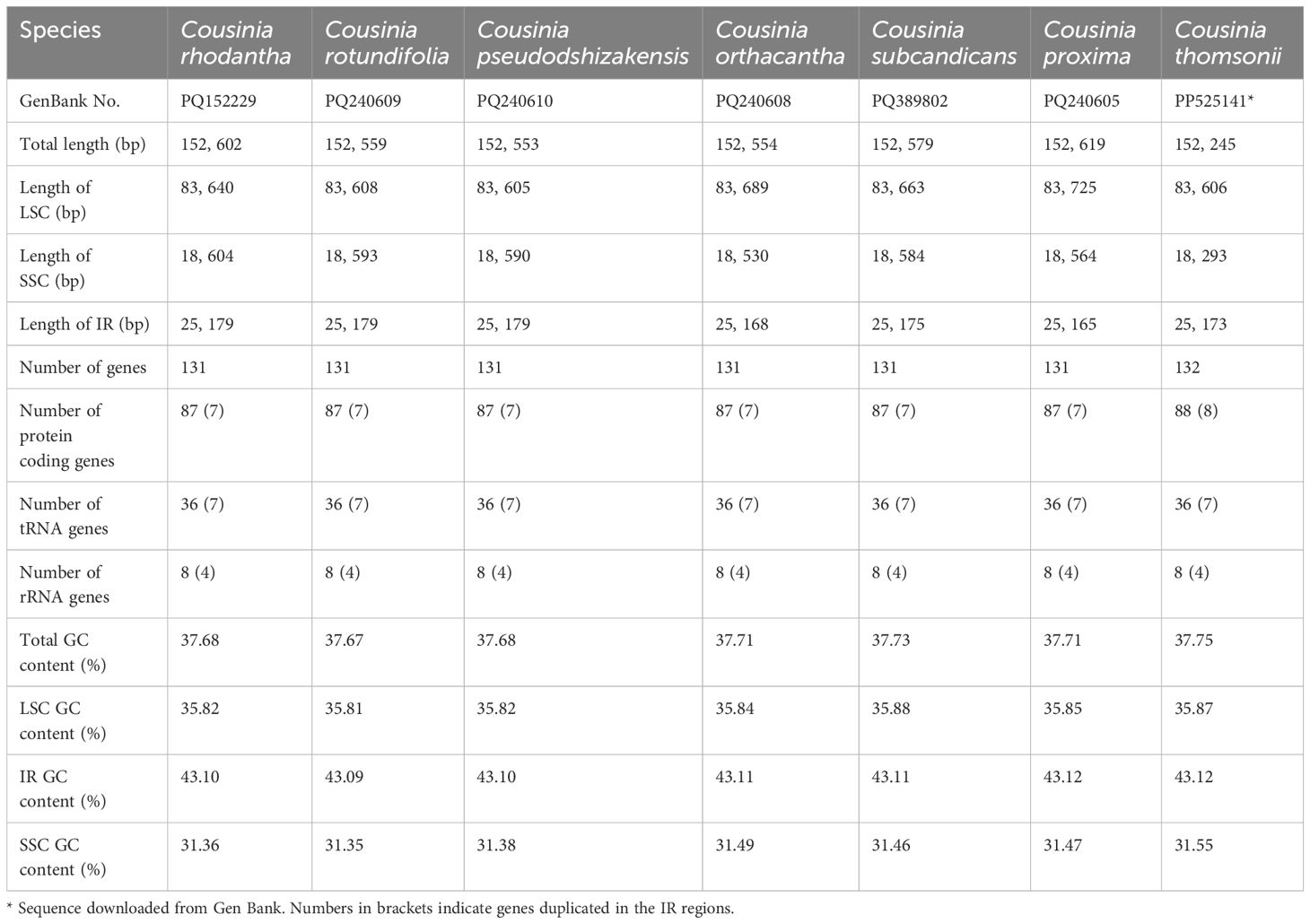
Table 1. General characteristics of the plastomes of the seven Cousinia species included in this study.
In six species 131 genes were annotated, of which 87 were protein-coding genes (PCGs), 36 were transfer RNA (tRNA) genes, and 8 were ribosomal RNA (rRNA) genes (Table 2). Notably, 18 genes within the cp genome are duplicated. This set of duplicated genes includes seven tRNAs (trnA-UGC, trnI-CAU, trnI-GAU, trnL-CAA, trnN-GUU, trnR-ACG, trnV-GAC), four rRNAs (rrn16, rrn23, rrn4.5, rrn5), and seven PCGs (ndhB, rpl2, rpl23, rps12, ycf2, ycf15, rps7). Sixteen genes possess introns, of which 11 are protein-coding genes (namely atpF, rpoC1, rpl2, ndhB, ndhA, petB, petD, rps16, rps12, ycf3, and clpP) and five are tRNA genes (specifically tRNA-UGC, trnI-GAU, trnK-UUU, trnL-UAA, and trnV-UAC). Two genes, ycf3 and clpP have two introns, while the remaining 14 genes have only one.
Long repeat sequences and SSR analyses
Among the seven plant species within the Cousinia genus, the number of long repeats ranged from 39 to 44 (Figure 3). The long repetitive sequences were primarily forward and palindromic repeats, with only a single reverse repeat identified. Of these repeats, the shortest is only 30 bp in length, while the longest is as large as 57 bp.
Five types of simple sequence repeats (SSRs) were identified: mononucleotide, dinucleotide, trinucleotide, tetranucleotide, and hexanucleotide. Among these, the A/T repeat was the most commonly observed across all species (Figure 4). The C/G repeat type was absent in C. rotundifolia, but it appeared once in each of the other species. A repeat type, AAAC/GTTT, was detected in C. orthacantha, C. subcandicans, and C. proxima, while the repeat type AATAGG/ATTCCT was found only in C. thomsonii. The majority of the identified SSRs were located in the LSC region of the genome.
IR expansion and contraction
A comparison of Inverted Repeat (IR) region boundaries of Cousinia species with Arctium lappa revealed that they share a similar genomic arrangement. However, some differences were detected (Figure 5). In all Cousinia species, the rps19 gene was found to be situated at the JLB (Large Single Copy and Inverted Repeat junction b), where it extended 60 base pairs into the IRb (Inverted Repeat b region). In contrast, in the case of Arctium lappa, the rps19 gene was located in the Large Single Copy (LSC) region, 70 base pairs from the JLB junction. In Arctium lappa, the ycf1 gene had a duplicate copy, which was positioned at the JSB (Small Single Copy and Inverted Repeat b junction) and JSA (Small Single Copy and Inverted Repeat a junction). However, the ycf1 gene was located only in JSA in all species of the Cousinia genus.
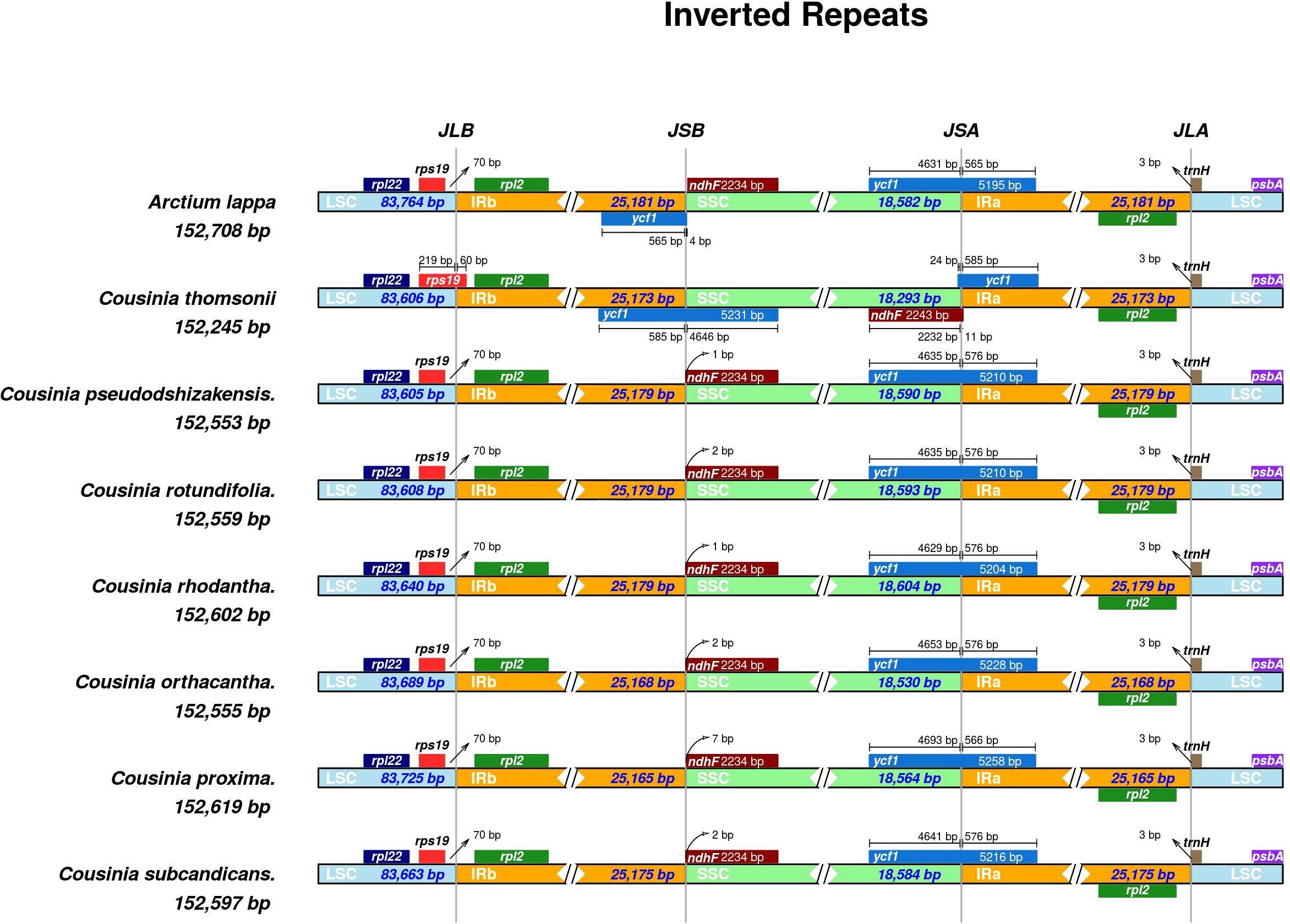
Figure 5. Comparisons of the borders of large single copy (LSC), small single copy (SSC), and inverted repeats IR regions (IR) among seven Cousinia species and Arctium lappa.
Nucleotide diversity
The examination of nucleotide diversity in six Cousinia species showed that their cp genomes are highly conserved. From 152,892 nucleotide sites only 412 were polymorphic of which 259 were parsimony informative. Comparison of the cp genomes of two C. orthacantha specimens collected from populations inhabiting different ecological habitats revealed a single nucleotide difference (in the intron region of the rps16 gene). The Pi values for six plastomes ranged from 0 to 0.00807 (Figure 6). Four regions trnE-UUC – rpoB, trnL-UAA – trnF-GAA – ndhJ, rbcL and ycf1 exhibited Pi values greater than 0.005. The first three regions are situated in the LSC region of the plastome, while ycf1, located in the SSC region and approximately 6000 bp in length, had a Pi value of 0.00807. In contrast, all regions within the IR region had Pi values below 0.002.
Phylogenetic analysis
The complete plastome-based trees obtained using the Bayesian Inference (BI) and Maximum Likelihood (ML) methods were congruent. Our tribal level tree (Figure 7) included six subtribes (Arctiinae, Saussureinae, Centaureinae, Carduinae, Onopordinae, and Carlininae) of Cardueae tribe. Except for Saussureinae, all subtribes formed monophyletic clusters. The Carlininae subtribe occupied a basal position. In the Arctiinae subtribe, the Cousinia species formed a monophyletic group with Arctium lappa from the Arctium genus (0.99/56).
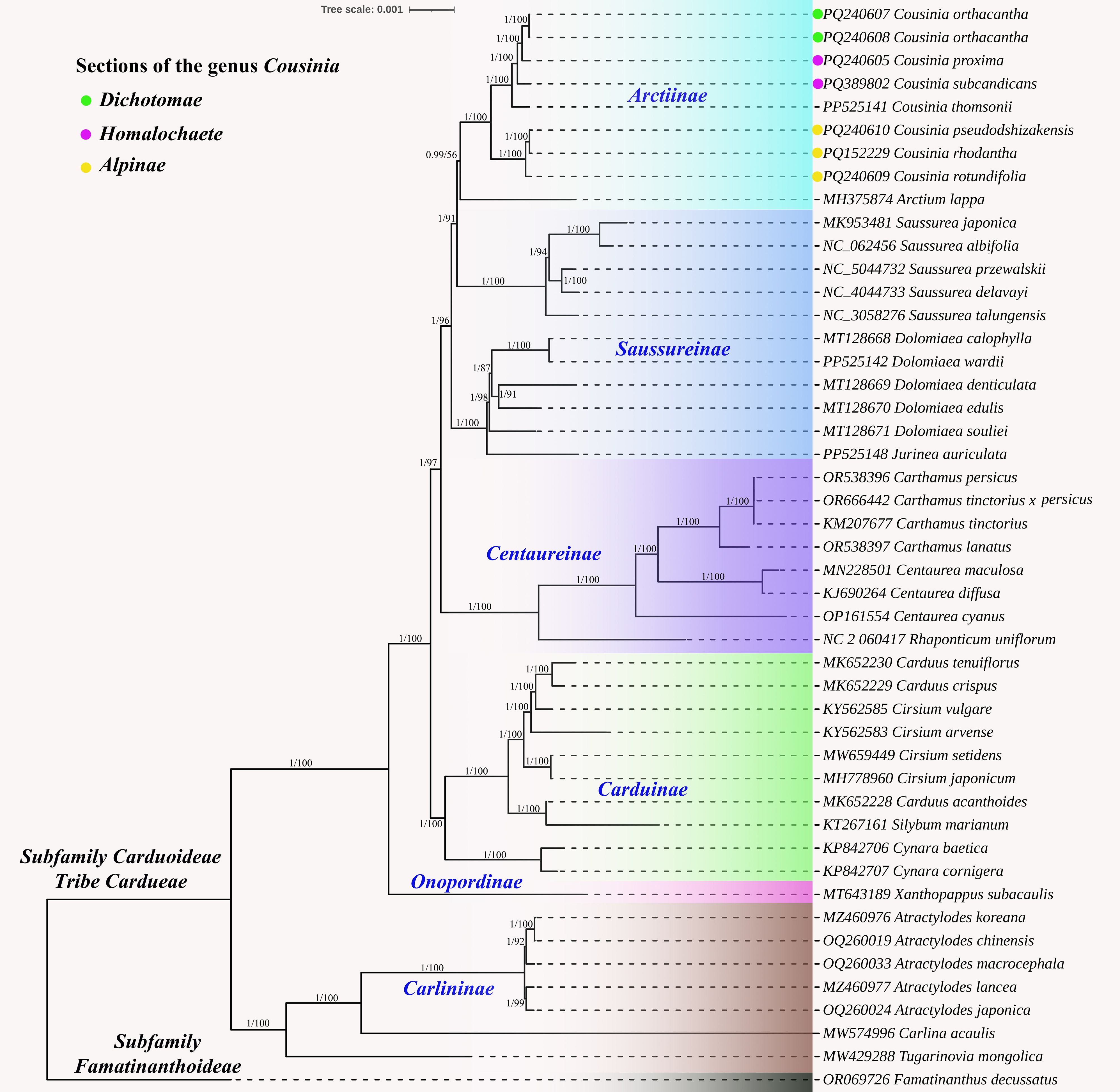
Figure 7. Phylogenetic tree constructed using the Bayesian inference (BI) and maximum likelihood (ML) methods based on the whole chloroplast genomes from 46 different species. The numbers near the branches represent the Bl posterior probabilities and MI bootstrap values.
The Cousinia species were divided into two distinct subclades. The first clade included C. pseudodshizakensis, C. rhodantha, and C. rotundifolia, which are part of the Alpinae section. The second clade consisted of C. orthacantha, C. proxima, C. subcandicans, and C. thomsonii. C. orthacantha belongs to the Dichotomae section, while C. proxima and C. subcandicans are part of the Homalochaete section.
Similarly, the nrDNA ITS based phylogeny recovered using BI and ML methods demonstrated congruence. However, while all subtribes except Carlininae formed monophyletic groups, Carlina libanotica Boiss., a member of the Carlininae subtribe, was unexpectedly positioned within the Carduinae subtribe (Figure 8). Within the Arctiinae subtribe, Cousinia species formed a well-supported monophyletic clade (0.98/76), clustering with Arctium species. The Cousinia clade was further subdivided into multiple subclades, however, some Cousinia species were not well resolved and did not correspond to their traditionally assigned taxonomic sections. Specifically, species from the Stenocephalae, Albidae, and Cousinia sections exhibited ambiguous phylogenetic placements, forming paraphyletic or polyphyletic groupings.
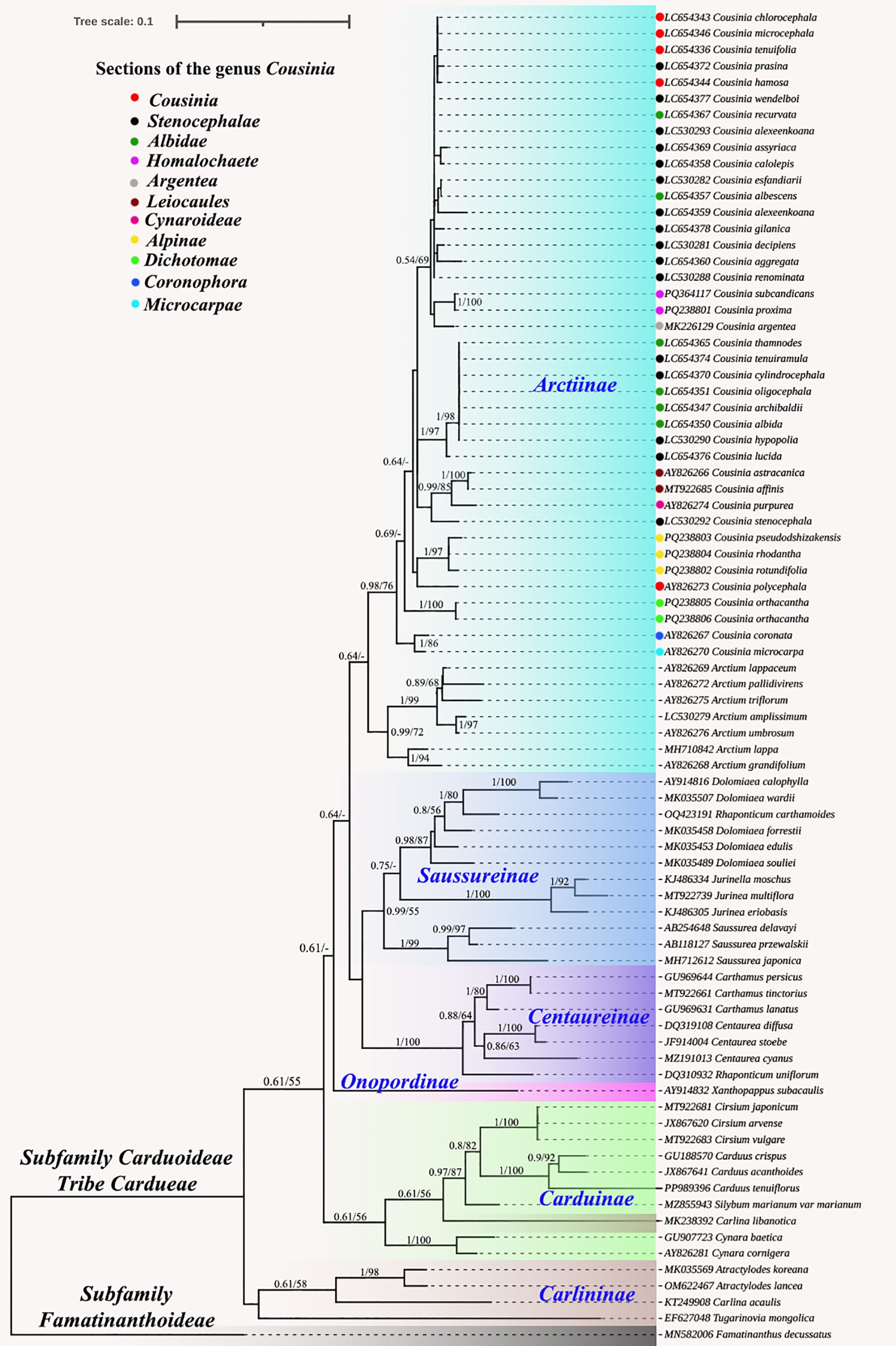
Figure 8. Phylogenetic tree constructed using the Bayesian inference (BI) and maximum likelihood (ML) methods based on the ITS from 80 different species. The numbers near the branches represent the Bl posterior probabilities and MI bootstrap values (below 0.52/52 not displayed).
Morphology of the anther appendages
The shape of the anther appendages of 7 species from Homalochaete, 9 species from Alpinae, and 4 species from section Dichotomae were examined under a microscope. The findings indicated that in the Homalochaete section, the appendages all investigated species are characterized by a narrow, elongated, acute, and toothed shape. The Alpinae section features appendages that are short, acute, or obtuse, and have an arched outline. Except for C. princeps, the anther appendages are generally narrowly long and acute. In the Dichotomae section, the appendages are short, acute, and very short with a toothed appendage (Figure 9).
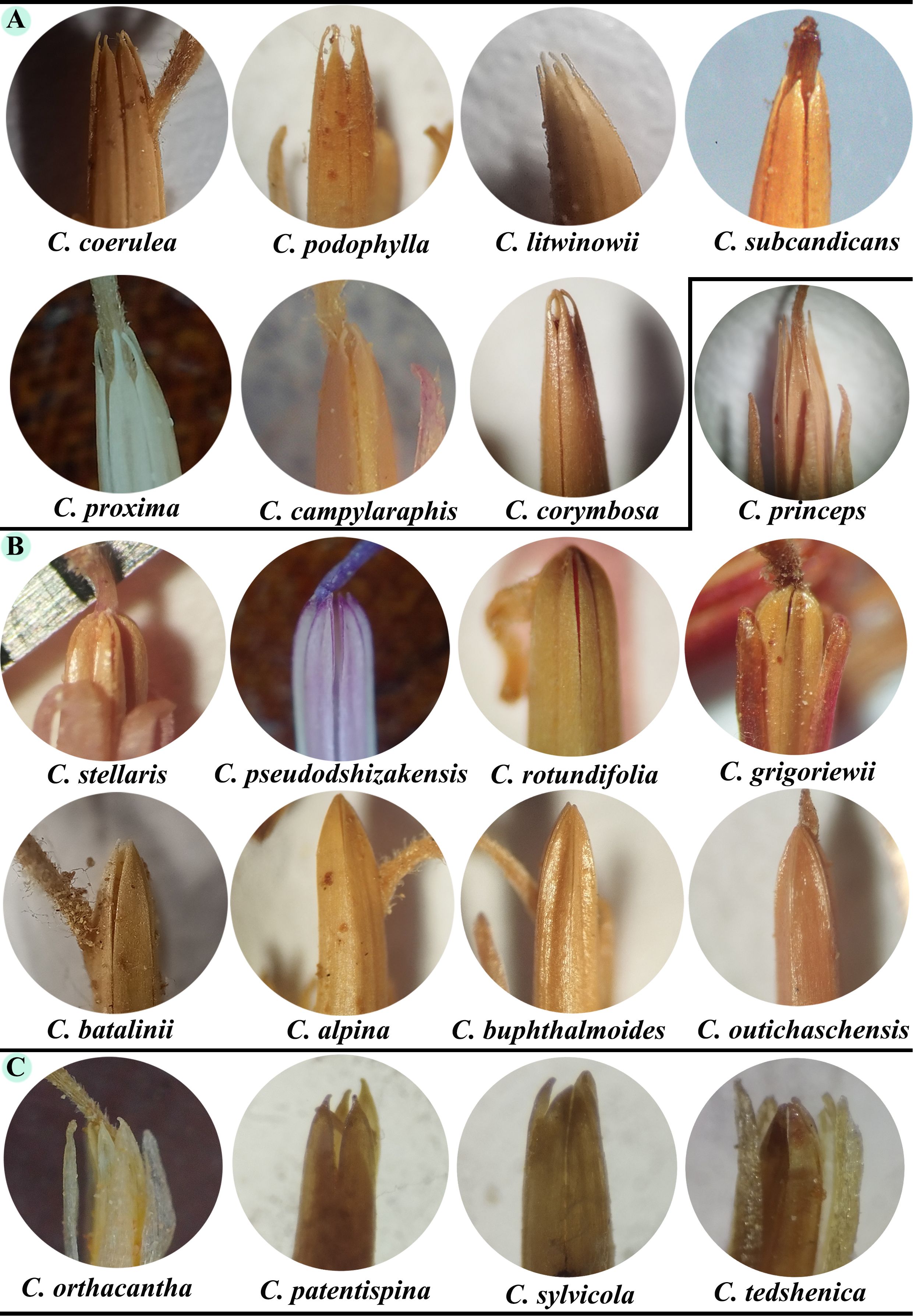
Figure 9. The form of the anther appendages in species from the (A) Homalochaete, (B) Alpinae, and (C) Dichotomae sections.
Discussion
In this research, we performed sequencing of six species of Cousinia and carried out a comparative analysis of their cp genomes. This is the first report of complete cp. genomes from the genus Cousinia. They were found to be similar to those of other genera in Asteraceae with typical double-stranded circular tetramer structures and sizes ranging from 152,555 bp to 155,619 bp (Xing et al., 2019; Wu et al., 2019; Yun and Kim, 2022; He et al., 2023). The cp. genomes of six Cousinia species encoded 131 genes like most other species of Asteraceae, pseudogenes ycf1 and rps19 were also detected (Liu et al., 2024). A total of 16 intron-containing genes were identified in six plants of the genus Cousinia. These included 14 genes with one intron, and two genes with two introns. These intron-containing genes can play important roles in regulating gene expression (Wu et al., 2023). The total GC contents of the complete cp. genomes among the seven species of the Cousinia varied 37.67 to 37.75 (Table 1). Among the LSC, SSC, and IR regions, the IR regions had the highest GC contents (43.09 to 43.12%), followed by the LSC (35.81 to 35.88) and SSC regions (31.35 to 31.55%). The IR regions had the highest GC contents among the four regions, which is likely due to the presence of two copies of rRNAs (rrna4.5, rrna5, rrna23, and rrna16) within this region (Shahzadi et al., 2020; Liu et al., 2024).
In this study, the repetitive sequences observed in the cp genomes of the seven Cousinia plant species displayed no significant heterogeneity in terms of their number, type, or length. Among the repetitive sequence types, reverse repeats were limited to only one in all species, while palindromic (P) repeats ranged from 18 to 21, and forward (F) repeats ranged from 20 to 23. Notably, complementary (C) repeats were absent in all seven Cousinia species. These long repetitive sequences play crucial roles in gene recombination and sequence structure variation (He et al., 2023). Furthermore, they may serve as valuable markers for differentiating Cousinia species, offering potential applications in species identification and phylogenetic studies (Gao et al., 2018).
SSRs, also called microsatellites, are widely utilized as valuable molecular markers in species identification and phylogenetic studies due to their high substitution rates (Upadhyay et al., 2010). In this study, a total of 273 SSRs were identified in the complete cp. genomes of seven Cousinia species, 158 were found to be mononucleotide repeats, constituting the majority (57.8%) of all SSRs (Figure 4). Previous studies have suggested that this may be related to natural selection and genetic mutation (He et al., 2023; Zhang et al., 2023).
Vascular plant cp genomes usually exhibit the contraction or expansion of boundaries within different genera or even within the same genus, which is the main factor leading to variations in the length and number of genes in various species (Gu et al., 2024). The LSC/IRb boundary was generally located between the rps19 and rpl2 genes in the plastid genomes of six Cousinia species and Arctium lappa (NC042724). However, in Cousinia thomsonii (PP525141), the boundary was located within the rps19 gene. Upon further analysis of rps19 using Geneious v9.0.2, an illegal start codon was detected, suggesting potential annotation or sequencing issues that require further investigation. Moreover, the orientation of the SSC region in C. thomsonii was found to be forward, in contrast to the reverse orientation observed in other Cousinia species and A. lappa. This unique structural variation highlights the genomic divergence within the group. In C. thomsonii and A. lappa, the expansion of the IR region resulted in partially duplicated ycf1 genes being located in the IRb region, thereby generating a pseudogene ycf1 at the IRb/SSC boundary. While most land plants typically have pseudogenes in their cp genomes at the SSC/IRa boundary (Cai et al., 2023), the six Cousinia species analyzed did not exhibit annotated pseudogenes at this boundary. This absence is likely due to nucleotide insertions or deletions (indels) that disrupted the formation of a stop codon, preventing the annotation of pseudogenes in these regions.
Cp genomes provide essential resources for phylogenetic and taxonomic studies due to their conserved structure and variability in specific regions. In this study, we identified regions with nucleotide diversity (Pi) values exceeding 0.005, including trnE-UUC – rpoB, trnL-UAA – trnF-GAA – ndhJ, rbcL, and ycf1. These highly variable regions represent potential molecular markers for resolving phylogenetic relationships within the genus Cousinia. Previous studies have also demonstrated the effectiveness of cp markers such as trnL-trnF, trnL-trnT-rps4, and rpl32-trnL in differentiating Cousinia species (Susanna et al., 2006; López-Vinyallonga et al., 2009, 2011). The results highlight the utility of cp genome variability for species-level discrimination and evolutionary studies in Cousinia.
The phylogenetic analysis of the complete plastomes demonstrated congruent topologies, underscoring the reliability of plastome data for resolving phylogenetic relationships within the Cardueae tribe. One of the notable findings is the monophyletic clustering of most subtribes, with the exception of Saussureinae, indicating that this subtribe might be polyphyletic. This result aligns with previous studies suggesting complexities in Saussureinae’s taxonomy and phylogenetic placement (Herrando-Moraira et al., 2020). The basal position of the Carlininae subtribe is consistent with earlier phylogenetic reconstructions, supporting its potential as an ancestral lineage within Cardueae (Herrando-Moraira et al., 2019). Within the Arctiinae subtribe, the monophyletic grouping of Cousinia species with Arctium lappa supports previous findings by López-Vinyallonga et al. (2009, 2011), who identified a close phylogenetic relationship between these genera.
In this study, we investigated the molecular and morphological distinctions within Cousinia, particularly focusing on anther appendage shape as a taxonomic trait, alongside molecular phylogenetic data. Our results revealed two major molecular clades among the Cousinia species, which show some alignment with traditional sectional classifications, though with notable exceptions and inconsistencies. The first molecular clade, which includes C. pseudodshizakensis, C. rhodantha, and C. rotundifolia, corresponds to the Alpinae section. This molecular grouping is consistent with morphological observations, where species in the Alpinae section exhibit short, acute or obtuse anther appendages with an arched outline. However, the morphological data also suggest that C. princeps from the Alpinae section deviates in its anther appendage shape, which aligns more closely with species in the Tianschanicae and Subappendiculatae sections. This discrepancy indicates that while anther appendage morphology provides useful diagnostic features, it may not be entirely sufficient to classify species at a sectional level, especially when taxonomic boundaries overlap. The second molecular clade consists of species from the Dichotomae and Homalochaete sections, including C. orthacantha, C. proxima, C. subcandicans. Morphologically, these species share similar anther appendage traits narrow, elongated, acute, and toothed appendages in the Homalochaete section and short, acute, toothed appendages in the Dichotomae section. The congruence between molecular data and the anther appendage morphology for these species supports the close evolutionary relationship between the Dichotomae and Homalochaete sections. This finding is consistent with previous work by Rechinger (1972) and Sennikov (2010), who highlighted the taxonomic significance of anther appendage morphology in Cousinia. These traits serve as valuable markers in identifying evolutionary relationships within sections. To resolve the current uncertainties in the intersectional classification of Cousinia, particularly concerning the Alpinae, Tianschanicae, and Subappendiculatae sections, further molecular and morphological analyses are essential.
The comparative analysis of nrDNA ITS sequences, cp data, and morphological traits provided critical insights into the phylogenetic relationships within Cousinia. While plastome phylogenies demonstrated stable and congruent topologies, supporting well-defined monophyletic clades, ITS based phylogenies were largely consistent with plastome data, with only Carlina libanotica unexpectedly positioned within the Carduinae subtribe rather than Carlininae. This anomaly highlights the limitations of ITS as a sole marker for species delimitation. In particular, taxa belonging to the groups Stenocephalae, Albidae, and Cousinia had unclear phylogenetic positions, establishing paraphyletic or polyphyletic groupings. These results are consistent with earlier studies by Kalouti et al. (2022), which also reported evolutionary complexity in these areas.
Morphological data, particularly anther appendage shape, showed partial alignment with molecular clades, reinforcing its taxonomic relevance. For instance, the Alpinae section displayed relatively strong molecular-morphological congruence. Similarly, species from the Dichotomae and Homalochaete sections exhibited consistent molecular clustering, with anther appendage morphology further supporting their evolutionary affinity. These findings highlight the necessity of an integrative taxonomic approach, where plastome data offer robust phylogenetic resolution, ITS markers capture finer-scale divergence but require cautious interpretation, and morphological traits provide critical but sometimes homoplastic diagnostic features. A combined strategy incorporating plastome, nuclear, and morphological data is essential for refining the taxonomy and phylogenetic framework of Cousinia.
Conclusion
This study compares the entire cp genome sequences within Cousinia genus for the first time. There were 131 genes found in the cp genomes of six different Cousinia species, which varied in size from 152,553 to 152,619 bp. Higher nucleotide polymorphism levels were found in trnE-UUC – rpoB, trnL-UAA – trnF-GAA – ndhJ, rbcL, and ycf1 genomic regions, indicating possible markers for species differentiation. Within Cousinia, two different clades were identified by phylogenetic analysis using whole cp genomes. In addition, anther appendage morphology investigation revealed C. princeps from Alpinae section would need to be reclassified. Additionally, the phylogenetic analysis of the Cardueae tribe and its subtribes, along with the subtribal relationships within the genus, suggests that cp genome data holds significant potential for revising evolutionary and taxonomic frameworks within both Cousinia and broader Asteraceae studies. These results are preliminary because of the small number of species that were examined. Enhancing the intersectional classification within Cousinia requires additional study using a larger sample size.
Data availability statement
The datasets presented in this study can be found in online repositories. The names of the repository/repositories and accession number(s) can be found in the article/Supplementary Material.
Author contributions
BK: Writing – review & editing, Writing – original draft. ST: Writing – original draft, Writing – review & editing. DA: Writing – original draft. ZY: Methodology, Writing – original draft, Writing – review & editing. LL: Funding acquisition, Writing – review & editing.
Funding
The author(s) declare that financial support was received for the research and/or publication of this article. This research was funded by the Joint Special Fund for Agricultural Basic Research in Yunnan Province (202401BD070001-052), the Open Research Program of State Key Laboratory for Conservation and Utilization of Bioresource in Yunnan Province (gzkf2022003), and Grant No. PRIM 01-73 “The modernization of the Institute of Botany of the Academy of Sciences of the Republic of Uzbekistan”, funded under the MUNIS Project, supported by the World Bank and the Government of the Republic of Uzbekistan. The statements do not necessarily reflect the official position of the World Bank and the Government of the Republic of Uzbekistan.
Acknowledgments
The authors would like to express their sincere gratitude to Dr. Sergei Volis and the reviewers for their insightful comments and constructive feedback, which greatly contributed to the improvement of this manuscript.
Conflict of interest
The authors declare that the research was conducted in the absence of any commercial or financial relationships that could be construed as a potential conflict of interest.
Generative AI statement
The author(s) declare that Generative AI was used in the creation of this manuscript.
Publisher’s note
All claims expressed in this article are solely those of the authors and do not necessarily represent those of their affiliated organizations, or those of the publisher, the editors and the reviewers. Any product that may be evaluated in this article, or claim that may be made by its manufacturer, is not guaranteed or endorsed by the publisher.
Supplementary material
The Supplementary Material for this article can be found online at: https://www.frontiersin.org/articles/10.3389/fpls.2025.1522950/full#supplementary-material
Supplementary Table 1 | The information about sampled specimens.
Supplementary Table 2 | Gen Bank accession numbers of sampled specimens.
Supplementary Table 3 | Information of the cp and nrDNA ITS sequences used in this study.
References
Amiryousefi, A., Hyvönen, J., Poczai, P. (2018). IRscope: an online program to visualize the junction sites of chloroplast genomes. Bioinformatics 34, 3030–3031. doi: 10.1093/bioinformatics/bty220
Atazadeh, N., Sheidai, M., Attar, F., Koohdar, F. (2021). Molecular phylogeny and morphometric analyses in the genus Cousinia Cass. (Family Asteraceae), sections Cynaroideae Bunge and Platyacanthae Rech. f. Caryologia 74, 39–50. doi: 10.36253/caryologia-1114
Beier, S., Thiel, T., Münch, T., Scholz, U., Mascher, M. (2017). MISA-web: a web server for microsatellite prediction. Bioinformatics 33, 2583–2585. doi: 10.1093/bioinformatics/btx198
Cai, H., Xu, R., Tian, P., Zhang, M., Zhu, L., Yin, T., et al. (2023). Complete chloroplast genomes and the phylogenetic analysis of three native species of paeoniaceae from the Sino-Himalayan flora subkingdom. Int. J. Mol. Sci. 25, 257. doi: 10.3390/ijms25010257
Cassini, A. (1827). Carlinées-Prototypes. Dictionnaire des sciences naturelles 47. Le mormant Paris, pp. 498–505.
Darriba, D., Taboada, G. L., Doallo, R., Posada, D. (2012). jModelTest 2: more models, new heuristics and high-performance computing. Nat. Methods 9, 772. doi: 10.1038/nmeth.2106
de Candolle, A. P. (1824). Prodromus sytematis naturalis regni vegetabilis. De Candolle, A.P. de. 1838. Prodromus systematis naturalis regni vegetabilis, vol. 6 (Paris: Treuttel & Würtz).
Dierckxsens, N., Mardulyn, P., Smits, G. (2017). NOVOPlasty: de novo assembly of organelle genomes from whole genome data. Nucleic Acids Res. 45, e18–e18. doi: 10.1093/nar/gkw955
Frodin, D. G. (2004). History and concepts of big plant genera. Taxon 53, 753–776. doi: 10.2307/4135449
Gao, X., Zhang, X., Meng, H., Li, J., Zhang, D., Liu, C. (2018). Comparative chloroplast genomes of Paris Sect. Marmorata: insights into repeat regions and evolutionary implications. BMC Genomics 19, 133–144. doi: 10.1186/s12864-018-5281-x
Garcia-Jacas, N., Garnatje, T., Susanna, A., Vilatersana, R. (2002). Tribal and subtribal delimitation and phylogeny of the Cardueae (Asteraceae): a combined nuclear and chloroplast DNA analysis. Mol. Phylogenet. Evol. 22, 51–64. doi: 10.1006/mpev.2001.1038
Gu, J., Li, M., He, S., Li, Z., Wen, F., Tan, K., et al. (2024). Comparative chloroplast genomes analysis of nine Primulina (Gesneriaceae) rare species, from karst region of southwest China. Sci. Rep. 14, 1–16. doi: 10.1038/s41598-024-81524-6
Häffner, E., Hellwig, F. H. (1999). Phylogeny of the tribe Cardueae (Compositae) with emphasis on the subtribe Carduinae: an analysis based on ITS sequence data. Willdenowia 29, 27–39. doi: 10.3372/wi.29.2902
He, H., Wang, T., Tang, C., Cao, Z., Pu, X., Li, Y., et al. (2023). Complete Chloroplast Genomes of Saussurea katochaete, Saussurea superba, and Saussurea stella: Genome Structures and Comparative and Phylogenetic Analyses. Genes 14, 2002. doi: 10.3390/genes14112002
Herrando-Moraira, S., Calleja, J. A., Galbany-Casals, M., Garcia-Jacas, N., Liu, J. Q., López-Alvarado, J., et al. (2019). Nuclear and plastid DNA phylogeny of tribe Cardueae (Compositae) with Hyb-Seq data: A new subtribal classification and a temporal diversification framework. Mol. Phylogenet. Evol. 137, 313–332. doi: 10.1016/j.ympev.2019.05.001
Herrando-Moraira, S., Cardueae Radiations Group (in alphabetical order), Calleja, J. A., Chen, Y. S., Fujikawa, K., Galbany-Casals, M., et al. (2020). Generic boundaries in subtribe Saussureinae (Compositae: Cardueae): insights from Hyb-Seq data. Taxon 69, 694–714. doi: 10.1002/tax.12314
Kalouti, S., Osaloo, S. K., Attar, F., Susanna, A., Garcia-Jacas, N. (2022). Molecular phylogeny of Cousinia sections Albidae, Stenocephalae and Cousinia (Asteraceae): systematic implications. Phytotaxa. 536, 109–125. doi: 10.11646/phytotaxa.536.2.1
Katoh, K., Standley, D. M. (2013). MAFFT multiple sequence alignment software version 7: improvements in performance and usability. Mol. Biol. Evol. 30, 772–780. doi: 10.1093/molbev/mst010
Kearse, M., Moir, R., Wilson, A., Stones-Havas, S., Cheung, M., Sturrock, S., et al. (2012). Geneious Basic: an integrated and extendable desktop software platform for the organization and analysis of sequence data. Bioinformatics 28, 1647–1649. doi: 10.1093/bioinformatics/bts199
Knapp, H. D. (1987). On the distribution of the genus Cousinia (Compositae). Plant Syst. Evol. 155, 15–25. doi: 10.1007/BF00936283
Kuntze, O. (1891). Revisio Generum Plantarum Vascularium, Vol. 1, Part 1 (A. Felix, Leipzig, Dulau & Co. London U. Hoepli. Milano GE Stechert. New York C. Klincksiek. Paris).
Kurtz, S., Choudhuri, J. V., Ohlebusch, E., Schleiermacher, C., Stoye, J., Giegerich, R. (2001). REPuter: the manifold applications of repeat analysis on a genomic scale. Nucleic Acids Res. 29, 4633–4642. doi: 10.1093/nar/29.22.4633
Liu, X., Luo, J., Chen, H., Li, T., Qu, T., Tang, M., et al. (2024). Comparative analysis of complete chloroplast genomes of Synotis species (Asteraceae, Senecioneae) for identification and phylogenetic analysis. BMC Genomics 25, 769. doi: 10.1186/s12864-024-10663-x
Lohse, M., Drechsel, O., Bock, R. (2007). OrganellarGenomeDRAW (OGDRAW): a tool for the easy generation of high-quality custom graphical maps of plastid and mitochondrial genomes. Curr. Genet. 52, 267–274. doi: 10.1007/s00294-007-0161-y
López-Vinyallonga, S., Mehregan, I., Garcia-Jacas, N., Tscherneva, O., Susanna, A., Kadereit, J. W. (2009). Phylogeny and evolution of the Arctium-Cousinia complex (Compositae, Cardueae-Carduinae). Taxon 58, 153–171. doi: 10.1002/tax.581016
López-Vinyallonga, S., Romaschenko, K., Susanna, A., Garcia-Jacas, N. (2011). Systematics of the arctioid group: disentangling Arctium and Cousinia (Cardueae, Carduinae). Taxon 60, 539–554. doi: 10.1002/tax.602020
Mehregan, I., Kadereit, J. W. “The role of hybridization in the evolution of Cousinia s. str. (Asteraceae, Cardueae).” Willdenowia 39, no. 1 (2009), 35–47. doi: 10.3372/wi.39.39102
POWO (2025). Plants of the World Online. Facilitated by the Royal Botanic Gardens, Kew. Available online at: https://powo.science.kew.org/ (Accessed December 6, 2024).
Rechinger, K. H. (1953). Cousinia-Studien. Oesterreichische Botanische Z. 100, 437–477. doi: 10.1007/BF01815051
Rechinger, K. H. (1972). “Compositae-Cynareae I: Cousinia,” in Flora Iranica, vol. vol. 90 . Ed. Rechinger, K. H. (Akademische Druckund Verlagsanstalt, Graz).
Ronquist, F., Huelsenbeck, J., Teslenko, M. (2011). a Drft MrBayes version 3.2 manual: tutorials and model summaries. Available online at: http://brahms.biology.rochester.edu/software.html (Accessed September 1, 2024).
Rozas, J., Ferrer-Mata, A., Sánchez-DelBarrio, J. C., Guirao-Rico, S., Librado, P., Ramos-Onsins, S. E., et al. (2017). DnaSP 6: DNA sequence polymorphism analysis of large data sets. Mol. Biol. Evol. 34, 3299–3302. doi: 10.1093/molbev/msx248
Sennikov, A. N. (2010). A revision of Cousinia sections Alpinae (syn. Carduncellus), Subappendiculatae and Tianschanicae (Asteraceae) in the Kirghizian Tian-Shan and the neighbouring territories. Phytotaxa 5, 1–30. doi: 10.11646/phytotaxa.5.1.1
Shahzadi, I., Mehmood, F., Ali, Z., Ahmed, I., Mirza, B. (2020). Chloroplast genome sequences of Artemisia maritima and Artemisia absinthium: Comparative analyses, mutational hotspots in genus Artemisia and phylogeny in family Asteraceae. Genomics 112, 1454–1463. doi: 10.1016/j.ygeno.2019.08.016
Stamatakis, A. (2014). RAxML version 8: a tool for phylogenetic analysis and post-analysis of large phylogenies. Bioinformatics 30, 1312–1313. doi: 10.1093/bioinformatics/btu033
Susanna, A., Garcia-Jacas, N., Hidalgo, O., Vilatersana, R., Garnatje, T. (2006). The cardueae (compositae) revisited: insights from its, trnL-trnF, and matK nuclear and chloroplast dna analysis1, 2. Ann. Missouri Botanical Garden 93, 150–171. doi: 10.3417/0026-6493(2006)93[150:TCCRIF]2.0.CO;2
Susanna, A., Garcia-Jacas, N., Vilatersana, R., Garnatje, T. (2003). Generic boundaries and evolution of characters in the Arctium group: a nuclear and chloroplast DNA analysis. Collectanea Botanica 26, 101–118. doi: 10.3989/collectbot.2003.v26.17
Tscherneva, O. V. (1962). “Cousinia cass,” in Flora of the USSR, vol. vol. 27 . Ed. Shishkin, B. K. (Akademiya Nauk, Leningrad), 108–357.
Tscherneva, O. V. (1993). “Cousinia cass,” in Conspectus florae Asiae Mediae, vol. vol. 10 . Ed. Kamelin, R. V. (Editio Academiae Scientiarum Republicae Uzbekistan, Tashkent), 269–352.
Ulukuş, D., Tugay, O. (2020). Morphology, anatomy and palynology of two endemic Cousinia Cass. species (Sect. Cousinia, Asteraceae) and their taxonomic implications. Pakistan J. Bot. 52, 297–304.
Upadhyay, A., Kadam, U. S., Chacko, P., Karibasappa, G. S. (2010). Microsatellite and RAPD analysis of grape (Vitis spp.) accessions and identification of duplicates/misnomers in germplasm collection. Indian J. Horticult. 67, 8–15.
Uysal, T., Tugay, O., Ertuğrul, K., Bozkurt, M., Ulukuş, D., Demirelma, H. (2022). The chromosomal evolution and analyses of Turkish Cousinia species. Turkish J. Bot. 46, 244–259. doi: 10.55730/1300-008X.2685
von Bunge, A. (1865). Ubersichtliche Zusammenstellung der Arten der Gattung Cousinia Cass (Vol. 9, No. 2) (Saint Pétersbourg: Eggers et Comp).
Winkler, C. (1897). Mantissa synopsis specierum generis Cousiniae Cass. Trudy Imp. S. Peterburgsk. Bot. Sada 14, 185–243.
Wu, L., Fan, P., Zhou, J., Li, Y., Xu, Z., Lin, Y., et al. (2023). Gene losses and homology of the chloroplast genomes of Taxillus and Phacellaria species. Genes 14, 943. doi: 10.3390/genes14040943
Wu, Z. H., Liao, R., Dong, X., Qin, R., Liu, H. (2019). Complete chloroplast genome sequence of Carthamus tinctorius L. from PacBio Sequel Platform. Mitochondrial DNA Part B 4, 2635–2636. doi: 10.1080/23802359.2019.1643799
Xing, Y. P., Xu, L., Chen, S. Y., Liang, Y. M., Wang, J. H., Liu, C. S., et al. (2019). Comparative analysis of complete chloroplast genomes sequences of Arctium lappa and A. tomentosum. Biol. Plantarum 63, 565–574. doi: 10.32615/bp.2019.101
Yun, S., Kim, S. C. (2022). Comparative plastomes and phylogenetic analysis of seven Korean endemic Saussurea (Asteraceae). BMC Plant Biol. 22, 550. doi: 10.1186/s12870-022-03946-6
Keywords: Cardueae tribe, plastid genome, comparative analysis, phylogeny, anther appendages
Citation: Karimov B, Tojibaev SK, Azimova D, Yusupov Z and Liu L (2025) Comparative analysis of complete chloroplast genomes of Cousinia (Asteraceae) species. Front. Plant Sci. 16:1522950. doi: 10.3389/fpls.2025.1522950
Received: 05 November 2024; Accepted: 03 April 2025;
Published: 29 April 2025.
Edited by:
Enrico Vito Perrino, University of Foggia, ItalyReviewed by:
Zhumei Ren, Shanxi University, ChinaYan Cheng, Fujian Agriculture and Forestry University, China
Yvonne Liew, University of Malaya, Malaysia
Copyright © 2025 Karimov, Tojibaev, Azimova, Yusupov and Liu. This is an open-access article distributed under the terms of the Creative Commons Attribution License (CC BY). The use, distribution or reproduction in other forums is permitted, provided the original author(s) and the copyright owner(s) are credited and that the original publication in this journal is cited, in accordance with accepted academic practice. No use, distribution or reproduction is permitted which does not comply with these terms.
*Correspondence: Ziyoviddin Yusupov, Ym90YW55LmNvbmZlcmVuY2UudXpAbWFpbC5ydQ==; Lufeng Liu, bGxmODgzOUBzaW5hLmNvbQ==