- 1Pomology Institute, Shanxi Agricultural University, Taiyuan, Shanxi, China
- 2The Research Center of Soil and Water Conservation and Ecological Environment, Chinese Academy of Sciences and Ministry of Education, Yangling, Shaanxi, China
- 3Institute of Soil and Water Conservation, Chinese Academy of Sciences and Ministry of Water Resources, Yangling, Shaanxi, China
- 4University of Chinese Academy of Sciences, Beijing, China
- 5College of Soil and Water Conservation Science and Engineering, Northwest A&F University, Yangling, China
- 6CSIC, Global Ecology Unit CREAF-CSIC-UAB, Bellaterra, Catalonia, Spain
- 7CREAF, Cerdanyola del Vallès, Catalonia, Spain
Mixed-species reforestation/afforestation has been a common practice for improving ecosystem nutrient sustainability. In the Loess Hilly Region, however, it remains unclear whether the mixture of dinitrogen (N2)-fixing species Robinia pseudoacacia with economic species Amygdalus davidiana and Armeniaca sibirica can improve nutrient concentrations of green and senescent leaves and their nutrient resorption. In 2022, we analyzed N and phosphorus (P) concentrations in green (GLNC, GLPC) and senescent leaves (SLNC, SLPC), resorption efficiencies (NRE and PRE), and relative resorption efficiency (RRE) in mixtures of R. pseudoacacia × A. davidiana and R. pseudoacacia × A. sibirica and monocultures of three species, considering tree structure and topsoil (0-20 cm) properties. One-way ANOVA followed by Tukey’s test showed that senescent leaves nutrient concentrations for R. pseudoacacia and Student’s t test that green and senescent leaves of A. davidiana and A. sibirica were significantly greater in mixed stands than monocultures. Based on the same statistical methods, NRE and PRE of R. pseudoacacia varied; those of A. davidiana and A. sibirica were significantly lower in mixed stands than monocultures. Additionally, RRE was < 100% for R. pseudoacacia, but > 100% for A. davidiana and A. sibirica; NRE and PRE was significantly positively associated in A. sibirica and all species. The correlation analysis demonstrated that crown area were significantly positively correlated with N and P concentrations of green and senescent leaves but negatively correlated with NRE and PRE for A. davidiana and A. sibirica, however, they were unrelated for R. pseudoacacia and all species. Further, a random forest model analysis showed that soil available P was main factor driving GLNC for individual rather than all species. Within each species, linear regression analysis revealed that NRE was significantly negatively correlated with SLNC; for A. davidiana and A. sibirica, NRE and PRE were significantly negatively correlated with SLNC and SLPC, respectively. In contrast, the PRE of all species was significantly negatively correlated with GLPC but positively correlated with SLPC. Overall, the results suggested that tree mixture increased N and P cycling more in A. davidiana and A. sibirica than in R. pseudoacacia, in the plant-soil system.
1 Introduction
Reforestation/afforestation has historically been a common practice used to control degraded land (Payn et al., 2015) and improve nutrient cycling in terrestrial ecosystems (Lamb et al., 2005). Although the global planted forest area increased by 123 million ha between 1990 and 2020 (FAO, 2020), plantations have not always promoted nutrient cycling (Delgado-Baquerizo et al., 2013; Jiang et al., 2019). This expansion often relies on monoculture plantations, which have led to declines in soil fertility, ecological services, and functional stability (Liu et al., 2018). In contrast, suitable combinations of mixed species have been demonstrated to increase ecosystem stability (Forrester et al., 2006) and enhance resilience to both biotic and abiotic stressors (Coll et al., 2018). In particular, planting dinitrogen (N2)-fixing species in mixed plantations can improve soil nitrogen availability and nutrient cycling (Forrester et al., 2006; Stewart et al., 2008; Nygren and Leblanc, 2015). However, current research has focused mainly on the mechanisms by which N2-fixing or non-N2-fixing species promote nutrient cycling in pure plantations (Cao and Chen, 2017; Deng et al., 2019; Xu et al., 2020). Therefore, how N2-fixing and non-N2-fixing species promote nutrient cycling differently in mixed versus pure plantations is unknown.
Compared with monocultures, interspecific competition for aboveground light and belowground nutrients consumes some of the energy used for nutrient uptake (Li et al., 2018; Zhu et al., 2020). This implies that mixed N2-fixing and non-N2-fixing species tend to maintain a balance of nutrient use strategies based on the environmental conditions (Jucker et al., 2015; Bu et al., 2019; Lin et al., 2022). For instance, leaf N and phosphorus (P) concentrations can be used to quantify the balance/status of bioelements between N2-fixing and non-N2-fixing species in mixed and monoculture plantations (Sardans et al., 2012; Peñuelas et al., 2013; Hayes et al., 2014; Sardans et al., 2021). Additionally, differences in the nitrogen (NRE) and phosphorus (PRE) resorption efficiencies of N2-fixing and non-N2-fixing species among mixed and pure plantations may reflect their adoption strategies to optimize nutrient use and conservation (Aerts, 1996; Reed et al., 2012) due to the influence of stand type on plant physiological activity (Chen and Chen, 2022). Therefore, nutrient interactions and balance among different species can be revealed by examining differences in leaf N and P concentrations and nutrient resorption efficiency (NuRE) of N2-fixing versus non-N2-fixing species in mixed plantations versus monocultures.
Leaf N and P concentrations have been widely reported to influence the N and P resorption efficiencies of mixed species (Chen et al., 2012; Vergutz et al., 2012; Deng et al., 2019). Previous studies have shown positive relationships between N and P resorption efficiencies and green leaf N and P concentrations (Zhang et al., 2018), but the relationships reported in other studies have been negative (Kobe et al., 2005; Vergutz et al., 2012; Liu et al., 2014) or not significant (Aerts, 1996; Sun et al., 2015). The difference is that N and P resorption efficiencies have negative effects on senescent leaf N and P concentrations because the decomposition of senescent leaves can increase the soil N and P concentrations (Wang et al., 2014; Zhou et al., 2016). These findings suggest that the difference in the N and P resorption efficiencies of mixed species can be reflected by the variation in green and senescent leaf N and P concentrations. Therefore, the relationship between the nutrient concentrations of green and senescent leaves and their resorption efficiencies can reveal the different roles of mixed N2-fixing and non-N2-fixing species in nutrient conservation.
Theoretically, leaf N and P concentrations and their resorption efficiencies in mixed and monoculture species are largely influenced by soil nutrient availability (Tully et al., 2013) through variations in stand characteristics (incl. tree structure and leaf morphological traits) and root distributions (Forrester et al., 2006; Jucker et al., 2015; Bu et al., 2019). Leaf N and P concentrations of mixed species are also indicated by leaf morphological traits in the variation of interspecific competition for light under different stand structures (Sanz-Perez et al., 2009; Duan et al., 2014; Metz et al., 2016). Furthermore, mixed N2-fixing species can increase soil N availability by rhizobia (Forrester et al., 2006; Paula et al., 2015; Waithaisong et al., 2020) but decrease soil P availability due to high P demand for N fixation (Augusto et al., 2013; Yuan et al., 2016). Thus, mixed species may modify N and P resorption efficiencies based on variations in soil N and P availability (Brant and Chen, 2015) and the potential effects of stand characteristics on leaf N and P concentrations (Vergutz et al., 2012; Liu et al., 2014; Xu et al., 2020). However, few studies have evaluated the effects of aboveground (stand) and belowground (soil nutrient availability) characteristics influenced by mixed planting strategies on leaf N and P concentrations and the resorption efficiencies of N2-fixing and non-N2-fixing species. Therefore, it is necessary to determine the feedback signals between leaf N and P concentrations and the nutrient resorption efficiencies of N2-fixing and non-N2-fixing species with soil N and P concentrations in monoculture and mixed plantations influenced by stand characteristics and soil nutrients to elucidate the mechanism by which mixed species affect nutrient cycling.
The historic degradation of natural vegetation across the Loess Plateau (covering 6.4 × 105 km2) resulted in the occurrence of drought and soil erosion. In 1999, the Chinese government initiated the “Grain for Green” project with the objective of increasing plantation cover. By 2021, this initiative resulted in a 402.11% increase in plantation coverage (Meng et al., 2023). However, planted forests mostly consist of monocultures, which have a negative impact on the ecological environment of the Loess Plateau, including the depletion of soil fertility (Chen et al., 2015). The fast-growing N2-fixing tree species Robinia pseudoacacia L. has been widely introduced as a pioneer afforestation species into the Loess Plateau because of its high drought tolerance and resistance to low soil fertility (Cierjacks et al., 2013; Tsunekawa et al., 2014). However, the growth of R. pseudoacacia is limited by soil P availability and becomes increasingly limited by soil P as the stand ages (Cao and Chen, 2017; Liu et al., 2017). In addition, the branches that have withered over the long-term (19–25 years) growth of R. pseudoacacia plantations have led to concerns about their nutrient status. Although previous studies have examined the leaf N and P concentrations and N and P resorption efficiencies of mixed species (Bu et al., 2019; Lin et al., 2022), they have not included R. pseudoacacia, limiting our understanding of the nutrient status of mixed N2-fixing species (Forrester et al., 2006; Coll et al., 2018). Coincidentally, the native species Amygdalus davidiana (Carr.) C. de Vos ex Henry var. davidiana and Armeniaca sibirica (L.) Lam. for ecological (e.g., drought-resistant, well-developed roots and strong barberries) and economic benefits (e.g., the kernel of A. davidiana is often made for decoration, while the pulp of A. sibirica is edible) often mixed planted with R. pseudoacacia on the Loess Plateau. However, the mechanism by which the mixed species R. pseudoacacia alters leaf N and P concentrations and their resorption efficiencies by influencing stand and soil characteristics remain uncertain.
In the present study, green and senescent leaves of individual species and soil samples from R. pseudoacacia monocultures and mixed plantations were collected. The objectives of this study were to evaluate the effects of N2-fixing (R. pseudoacacia) and non-N2-fixing trees (A. davidiana and A. sibirica, respectively) in mixed and monoculture plantations on leaf N and P concentrations and their resorption efficiencies. These aims were achieved by comparing the differences in leaf N and P concentrations and resorption efficiencies between monocultures and mixed species and by examining the relationships between these variables and influencing factors such as stand characteristics and soil properties. It was hypothesized that (1) multispecies mixing could increase the leaf N and P concentrations of R. pseudoacacia, A. davidiana and A. sibirica; (2) species mixing could reduce the NRE but increase the PRE of R. pseudoacacia, A. davidiana and A. sibirica and increase the soil P limitation for R. pseudoacacia; (3) correlations between the leaf N concentration and NRE and the leaf P concentration and PRE could not be significant in R. pseudoacacia from different plantations but be significantly negative in A. davidiana and A. sibirica.
2 Materials and methods
2.1 Study site
The study was conducted in Ansai County, Shaanxi Province (36°35’21”-36°36’40” N, 109°13’12”-109°16’31” E), the hinterland of the Loess Plateau, where the mean annual temperature and precipitation are 8.8°C and 510 mm, respectively. The rainy season is concentrated between June and September (72.9%). The soils are categorized as Calcic Cambisols (FAO and UNESCO, 1974). Over the past two centuries, the original vegetation has been completely devastated. Secondary forest regeneration began naturally in the 1970s, and plantation efforts have increased significantly since 1999, following the project to return farmland to plantations. Due to soil erosion over a thousand years, the background data on soil properties are quite similar on the Loess Plateau. The initial afforestation densities varied across the the Loess Plateau depending on tree species and site conditions, and later, with the growth of vegetation, the stand density changed. Thus, after 24 years of growth (up to 2022), changes in interspecific relationships and individual differences of plantation vegetation due to stand density are a crucial part of the natural process of succession.
2.2 Experimental design
The investigation included five type of plantations on the Loess Hilly Region, and the stand density for each plot was determined through a counting process. There were categorized into two stand types: monoculture plantations of R. pseudoacacia (RP, 1083 ± 42 trees ha-1), A. davidiana (AD, 733 ± 33 trees ha-1), and A. sibirica (AS, 700 ± 58 trees ha-1) and mixed plantations of R. pseudoacacia (1192 ± 22 trees ha-1) with A. davidiana (325 ± 14 trees ha-1) (RPAD) and R. pseudoacacia (1025 ± 80 trees ha-1) with A. sibirica (308 ± 55 trees ha-1) (RPAS). Specifically, two tree species combinations were designed: RPAD, RP, and AD; and RPAS, RP, and AS. The objectives of this study were to determine which tree species presented greater leaf N and P concentrations and lower nutrient resorption efficiencies in mixed stands than in their corresponding monoculture stands and to identify the factors that influence these phenomena. The plantations were established approximately 16–25 years ago and are situated on loess soil with a southwest orientation. Three 20 m × 20 m plots from each plantation, 15 plots in total, separated by less than 3 km, and a 5-m buffer zone around each plot to avoid edge effects were selected for analysis (see Supplementary Table S1).
2.3 Vegetation survey and sampling
To ensure that the sample is representative, we select standard wood at the four corners and center of the quadrat. In each plantation stand, green and senescent leaves from pure plantations were collected from five representative trees of each species without withered branches, and samples from mixed plantations were taken from adjacent two different species. The diameter at breast height (DBH, cm) was measured using a tree diameter tape. The average tree height (AH, m) was determined using a height gauge. Meanwhile, the crown area (CA, m2) was determined using a tapeline. When the biomass of plants was at its peak (in mid-August 2022), intact and complete green leaves were collected from the lower, middle, and upper canopies using high branch shears. From November to December 2022, fallen senescent leaves were collected from nylon nets (1 m × 1 m, 0.1 mm) suspended below the canopy. Based on the characteristics of defoliation season of different tree species, the collection time of senescent leaves of R. pseudoacacia and A. davidiana was set in late December 2022, while that of A. sibirica was set in mid-September 2022. Intact 5-10 green leaf samples without pests and diseases were selected and stored in ice bags to measure morphological features such as specific leaf area (SLA), leaf dry matter content (LDMC), and leaf tissue density (LD). Other green and senescent leaves were oven-dried to a constant mass at 105°C for 10 min and at 70°C for 24 h to determine the green leaf nitrogen (GLNC) and phosphorus (GLPC) concentrations and the senescent leaf nitrogen (SLNC) and phosphorus (SLPC) concentrations. The stand characteristics of the monocultures and mixed stands are shown in Supplementary Table S2.
Soil samples were collected from 0–20 cm in an S shape with five loci per plot using a 4 cm auger under the representative trees of each plantation, in mid-August 2022. Before the soil passed through the 2 mm mesh screen, we manually removed the roots, stones, and debris. The soil was divided into two parts: one part was air-dried, ground, and passed through a 0.15 mm sieve to determine the soil chemical properties, e.g., soil organic carbon (SOC), total N (TN), total P (TP), available N (AN), and available P (AP) concentrations; the other part was shade-dried and passed through a 1 mm porous sieve to determine the pH and particle composition (i.e., sand content, SA; silt content, SI; clay content, CL). At a depth of 0–20 cm, undisturbed soil was collected from each plot using a ring knife (100 cm3) to determine the bulk density (BD).
2.4 Sample measurement
The N concentrations of green (GLNC, g kg-1) and senescent (SLNC, g kg-1) leaves were determined by the Kjeldahl method (Kjeltec 2300 analyzer unit, Foss Tecator, Hoganas, Sweden) after digestion in H2SO4–H2O2 solution. The P concentrations of green (GLPC, g kg-1) and senescent (SLPC, g kg-1) leaves were determined by the molybdenum yellow method (U-2800 spectrophotometer, Shanghai, China). Specific leaf area (SLA, cm2 g-1), leaf dry matter content (LDMC, g g-1) and leaf tissue density (LD, g cm-3) were calculated using the following equation:
where leaf fresh weight (FW) and dry weight (DW) were determined using an electronic balance (precision 0.001 g), leaf thickness (LT, mm) was determined using a digital vernier caliper, and leaf area (LA, cm2) was determined using an Epson Perfection V850 Pro scanner (Epson (China) Co., Ltd. Beijing, China) and ImageJ software.
The soil organic C (SOC, g kg-1) concentration was determined by the calorific boiling and combustion with the H2SO4-K2Cr2O7 method (Nelson and Sommers, 1982). The soil total N (TN, g kg-1) concentration was determined by the Kjeldahl method (Bremner and Mulvaney, 1982), whereas the available N (AN, mg kg-1) concentration was measured by alkaline hydrolysis diffusion (Bao, 2000). The soil total P (TP, g kg-1) and available P (AP, mg kg-1) concentrations were determined by the molybdenum blue method after extraction with H2SO4–HClO4 and NaHCO3, respectively (Murphy and Riley, 1962). The soil pH was measured using an automatic acid–base titrator (PB-10 standard pH meter; Sartorius, Göttingen, Germany) with a water/soil ratio of 2.5:1. The cutting ring method was used to determine the soil BD (g cm-3) (Bao, 2000). The soil particle composition (SA/SI/CL, %) was determined using a Mastersizer 2000 Laser Particle Size Analyzer (Malvern Panalytical, Malvern, UK). The soil physicochemical properties are listed in Supplementary Table S3.
2.5 Data analysis
2.5.1 Nutrient resorption efficiency
The nutrient concentration percentage changes in green leaves were calculated using the following equation:
where ΔNuCgreenmix−mono (%) is the percentage changes in green leaf nitrogen (N) or phosphorus (P) concentrations between mixed and monoculture stands (see Supplementary Table S4), with NuCgreenmix and NuCgreenmix being the N or P concentrations in mixed and monoculture stands, respectively.
The nutrient concentration percentage changes in senescent leaves were calculated using the following equation:
where ΔNuCsenescentmix-mono (%) is the percentage changes in senescent leaf N or P concentrations between mixed and monocultures stands (see Supplementary Table S4), with NuCsenescentmix and NuCsenescentmix being the N or P concentrations in mixed and monoculture stands, respectively.
2.5.2 Nutrient resorption efficiency, relative nutrient resorption efficiency, and associated percentage changes
Nutrient resorption efficiency (NuRE, %) in monoculture stands was calculated using the following equation:
where NuREmono is the N or P resorption efficiency in monoculture stands (Figure 1a), with NuCsenescentmono and NuCgreenmono being the N or P concentrations in respective senescent and green leaves, and MLCF is the angiosperm-specific mass loss correction factor (0.784; Vergutz et al., 2012).
Nutrient resorption efficiency in mixed stands was calculated using the following equation:
where NuREmix is the N or P resorption efficiency in mixed stands (Figure 1b), with NuCsenescentmix and NuCgreenmix being the N or P concentrations in senescent and green leaves, respectively.
Relative nutrient resorption efficiency (RRE, %) in monoculture/mixed stands was calculated using the following equation:
where RREmono/mix is the relative nutrient resorption efficiency in monoculture/mixed stands (Figure 2a), with NREmono/mix and PREmono/mix being the respective N and P resorption efficiency in momoculture/mixed stands. When RREmono/mix > 100%, N limits plant growth; when RREmono/mix < 100%, P limits plant growth; and when RREmono/mix = 100%, N and P have the same limitations on plant growth (Reed et al., 2012).
The percentage changes in nutrient resorption efficiency were calculated using the following equation:
where ΔNuREmix-mono (%) is the percentage changes in N or P resorption efficiency between mixed and monoculture stands (see Supplementary Table S4), with NuREmix and NuREmono being the leaf N or P resorption efficiency in mixed stands and monocultures, respectively.
2.6 Statistical analysis
Data were tested for normality and homogeneity using the Shapiro–Wilk and Levene tests. When necessary, the data were log transformed. One way analysis of variance (ANOVA) followed by Tukey’s test was performed using SPSS 23.0 (SPSS Inc., Chicago, Illinois, USA) to determine the differences in green and senescent leaves concentrations, nutrient resorption, and relative nutrient resorption of R. pseudoacacia among the different stands and the differences among R. pseudoacacia, A. davidiana, and A. sibirica monocultures. Student’s t test was performed using SPSS software to examine differences between monoculture and mixed stands in green and senescent leaves concentrations, nutrient resorption, and its ratio of A. davidiana/A. sibirica, and examine differences between R. pseudoacacia and A. davidiana with A. sibirica in the same mixed stand. For further analysis, two-way ANOVA was performed using SPSS software to determine the effects of stand type, tree species, and their interaction on leaf nutrient concentrations and resorption efficiency. Linear regression analysis was performed using Origin Pro 2024b (OriginLab, Northampton, Massachusetts, USA) to determine the relationships between leaf nutrient concentrations, resorption efficiencies, and soil nutrient concentrations and between leaf nutrient concentrations and resorption efficiencies of specific and all species in monocultures and mixed stands. Pearson correlation analysis was performed using Origin Lab 2024b with “Correlation Plot” applications to confirm the correlations among the leaf nutrient concentrations, resorption efficiencies, and influencing factors (stand characteristics and soil properties) of the individuals and all species in the different stands. After standardizing the data with a Z-Score, random forest model analysis was performed using the “randomForest” package in R 4.2.2 (R Core Team, 2022) to determine the influencing factors that contribute to the leaf nutrient concentrations and resorption efficiencies of individuals and all species in pure and mixed stands. The significance level of the statistical analysis was set at P < 0.05.
3 Results
3.1 Leaf nutrient concentrations
In our study, two-way ANOVA revealed the significant effects of stand type, tree species, and their interaction on leaf nutrient concentrations (P < 0.05, Figure 1). Specifically, GLNC of R. pseudoacacia in the RPAS stand was 6.41% lower (P < 0.05; Figure 1a; Supplementary Table S4) and the GLPC of R. pseudoacacia in the RPAD stand was 15.70% higher than those in the RP stand (P < 0.05; Figure 1b; Supplementary Table S4). Additionally, the GLNC and GLPC of A. davidiana in the RPAD stand were 44.48% and 35.23% higher (P < 0.05; Figures 1a, b; Supplementary Table S4), respectively, and those of A. sibirica in the RPAS stand were 28.83% and 14.29% higher than those of their respective monocultures (P < 0.05; Figures 1a, b; Supplementary Table S4).
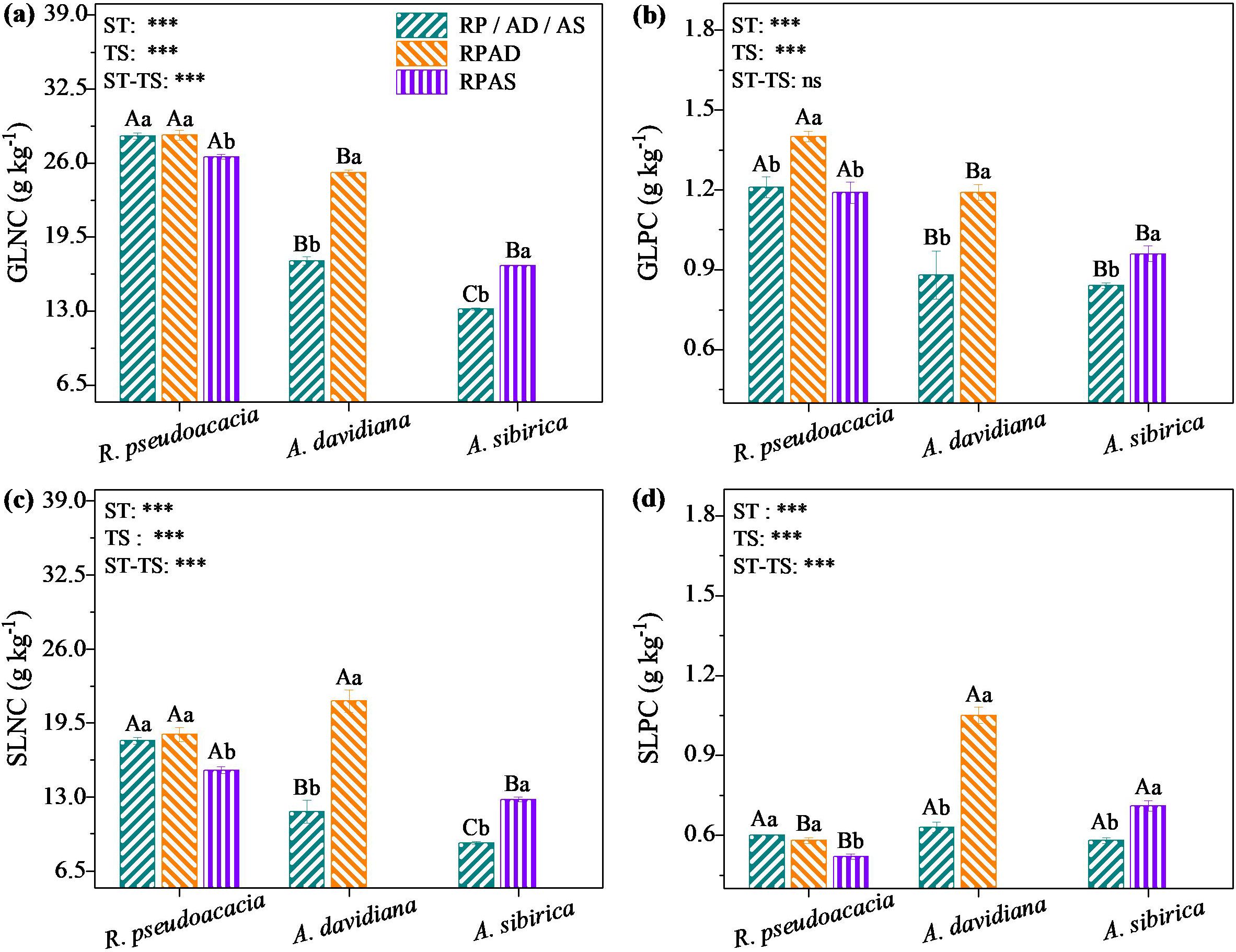
Figure 1. Changes in green leaf nitrogen (GLNC, a) and phosphorus (GLPC, b) concentrations, and senescent leaf nitrogen (SLNC, c) and phosphorus (SLPC, d) concentrations of different tree species in monocultures and mixed stands. The results of the two-way ANOVA for stand type (ST) and tree species (TS) and their interaction (ST-ST) on green and senescent leaf nitrogen and phosphorus concentrations are shown in the figures. Different uppercase letters indicate significant differences among different tree species for the same stand type, whereas different lowercase letters above the bars indicate significant differences among the same tree species for different stand types (***, P < 0.001; ns, P > 0.05).
Furthermore, the SLNC and SLPC of R. pseudoacacia in the RPAS stand were 14.44% and 13.33% lower than those in the RP stand, respectively (P < 0.05; Figures 1c, d; Supplementary Table S4). Moreover, the SLNC and SLPC of A. davidiana in the RPAD stand were 83.08% and 66.67% greater (P < 0.05; Figures 1c, d; Supplementary Table S4), respectively, and those of A. sibirica in the RPAS stand were 42.75% and 22.41% greater than those in the corresponding monoculture stands (P < 0.05; Figures 1c, d; Supplementary Table S4).
3.2 Leaf nutrient resorption efficiency and its relationship
Two-way ANOVA revealed the significant effects of stand type, tree species, and their interaction on NRE (Equation 6) and PRE (Equation 7) (P < 0.05, Figure 2). Compared with that in the RP stand, the PRE of R. pseudoacacia in RPAD stand was 10.73% greater (P < 0.05; Figure 2b; Supplementary Table S4). The NRE and PRE of A. davidiana in the RPAD stand were 29.93% and 29.35% lower than those in the AD stand, respectively, and those of A. sibirica in the RPAS stand were 12.21% and 9.31% lower than those in the AS stand, respectively (P < 0.05; Figures 2a, b; Supplementary Table S4).
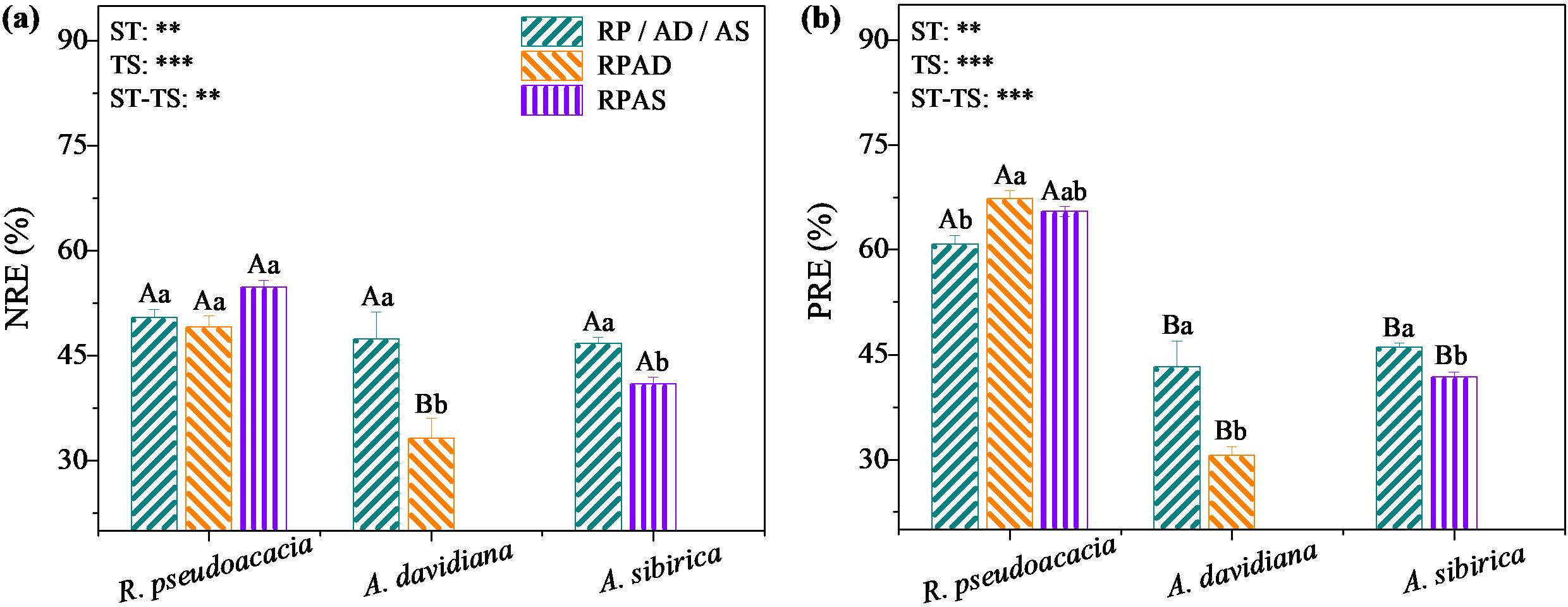
Figure 2. Changes in nitrogen (NRE, a) and phosphorus (PRE, b) resorption efficiencies of different tree species in monoculture and mixed stands. Results of the two-way ANOVA for stand type (ST) and tree species (TS) and their interaction (ST-ST) on green and senescent leaf nitrogen and phosphorus concentrations are shown in the figures. Different uppercase letters indicate significant differences among different tree species for the same plantation type, whereas different lowercase letters above the bars indicate significant differences among the same tree species for different plantation types (***, P < 0.001; **, P < 0.01).
Two-way ANOVA revealed the significant effects of stand type, tree species, and their interaction on relative nutrient efficiency (RRE, Equation 8) (P < 0.05, Figure 3a). Furthermore, the RRE of R. pseudoacacia was less than 100% and 12.05% lower in the RPAD stand than in the RP stand (P < 0.05), but those of A. davidiana and A. sibirica were greater than 100% and did not differ significantly between the pure and mixed plantations (P > 0.05) (Figure 3a; Supplementary Table S4). Moreover, linear regression analysis revealed that the NREs of A. sibirica and all species in the different stands were significantly positively correlated with PRE (P < 0.05, Figure 3b).
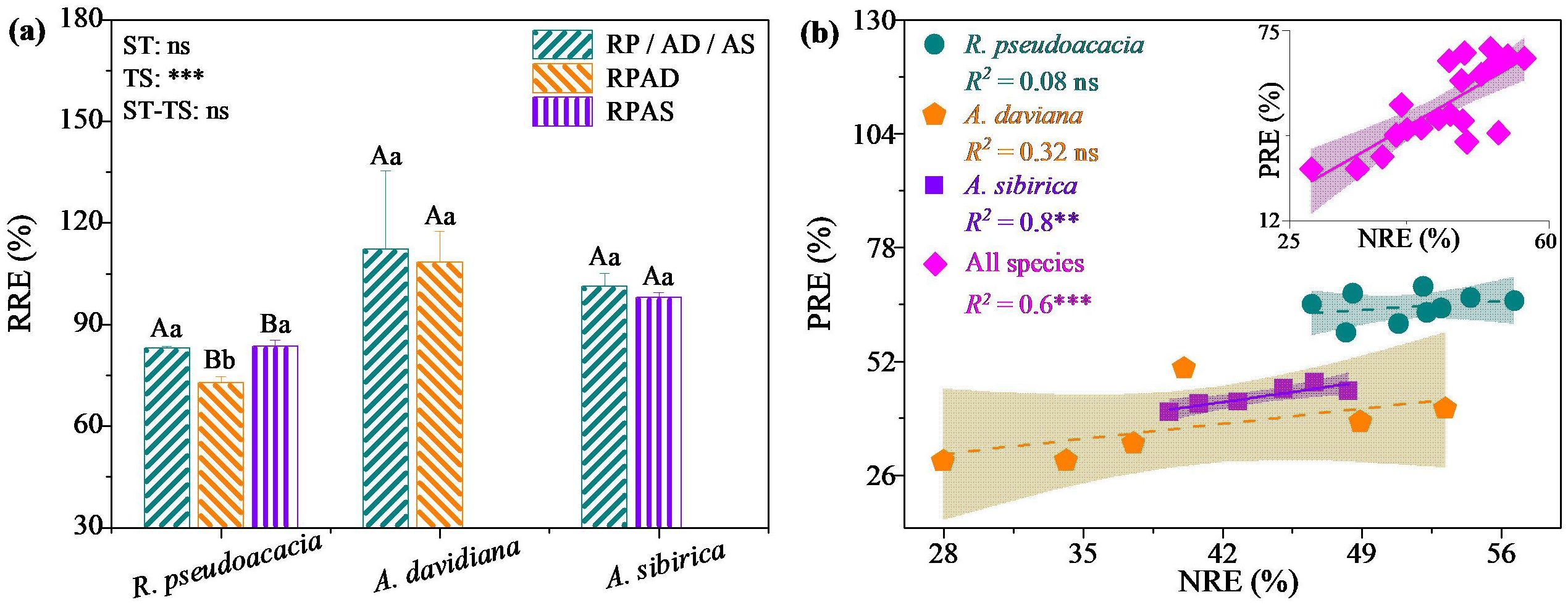
Figure 3. The relative resorption efficiency (RRE) of different tree species in monocultures and mixed stands (a) and the linear regression of associations between nitrogen (NRE) and phosphorous (PRE) resorption efficiencies of individuals and all species in monocultures and mixed stands (b). The results of the two-way ANOVA for stand type (ST) and tree species (TS) and their interaction (ST-ST) on green and senescent leaf nitrogen and phosphorus concentrations are shown in the figures. Different uppercase letters indicate significant differences among different tree species for the same stand type, while different lowercase letters above the bars indicate significant differences among the same tree species for different stand types (***, P < 0.001; **, P < 0.01; ns, P > 0.05).
3.3 Relationships between leaf nutrient concentrations, nutrient resorption efficiencies, and influencing factors
Pearson’s correlation coefficient was used to determine the relationships between the leaf nutrient concentration, resorption efficiency, and stand characteristics and soil physicochemical properties of individuals and all species (Figure 4). For individual species, the SLPC of R. pseudoacacia was significantly negatively correlated with the soil total phosphorus concentration; PRE was significantly positively correlated with the leaf dry matter content, leaf tissue density, and soil total phosphorous concentration but significantly negatively correlated with the specific leaf area (P < 0.05, Figure 4a). The GLNC, GLPC, SLNC, and SLPC of A. davidiana and A. sibirica were significantly positively correlated with crown area (P < 0.05, Figures 4b, c), but the opposite was true for NRE and PRE (P < 0.05, Figures 4b, c). In all species, GLNC, SLNC, and SLPC were significantly positively correlated with average tree height, soil total phosphorus concentration, and soil available nitrogen and phosphorus concentrations. The SLPC was significantly negatively correlated with diameter at breast height. The NRE and PRE were significantly positively correlated with average tree height and diameter at breast height (P < 0.05, Figure 4d).
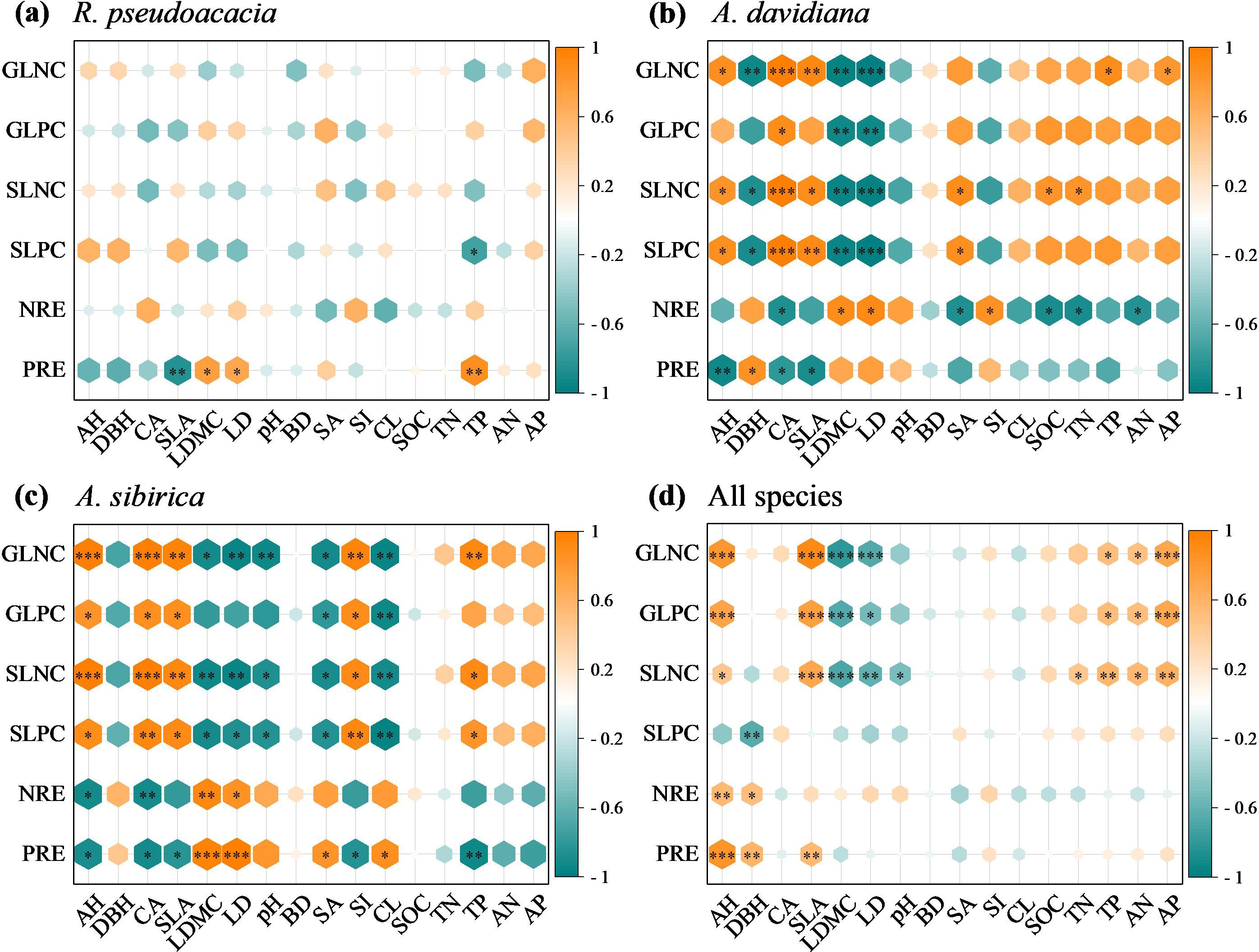
Figure 4. Pearson coefficients of the correlations between leaf nutrient concentrations, resorption efficiencies and stand characteristics and the soil properties of R. pseudoacacia (a), A. davidiana (b), A. sibirica (c), and all species (d) in monoculture and mixed stands. GLNC, green leaf nitrogen concentration; GLPC, green leaf phosphorus concentration; SLNC, senescent leaf nitrogen concentration; SLPC, senescent leaf phosphorus concentration; NRE, nitrogen resorption efficiency; PRE, phosphorus resorption efficiency; AH, average tree height; DBH, diameter at breast height; CA, crown area; SLA, specific leaf area; LDMC, leaf dry matter content; LD, leaf tissue density; pH, soil pH; BD, soil bulk density; SA, soil sand content; SI, soil silt content; CL, soil clay content; SOC, soil organic carbon concentration; TN, soil total nitrogen concentration; TP, soil total phosphorus concentration; AN, soil available nitrogen concentration; AP, soil available phosphorus concentration. ***, P < 0.001; **, P < 0.01; *, P < 0.05.
3.4 Factors contributing to leaf nutrient concentrations and nutrient resorption efficiencies
A random forest model was used to determine the relative importance of influencing factors to leaf nutrient concentrations and their resorption efficiencies for individuals and all species (Figures 5, 6). Among the individual species, soil available P concentration had large effects on the GLNC of R. pseudoacacia (32.84%), A. davidiana (12.07%), and A. sibirica (11.43%) (Figures 5a–c). The clay content had large effects on the GLPC of R. pseudoacacia (24.67%), and pH had effects on A. davidiana (11.54%), and A. sibirica (10.25%). The soil available P concentration had strong effects on the SLNC of R. pseudoacacia (15.44%), and pH had effects on A. davidiana (10.87%), and A. sibirica (14.01%). The soil available P concentration had large effects on the SLPC of R. pseudoacacia (23.22%), A. davidiana (10.87%), and A. sibirica (9.53%) (Figures 5a–c). For all species, specific leaf area had strong effects on the GLNC (62.42%), GLPC (47.6%), and SLNC (47.6%), and diameter at breast height on the SLPC (58.93%) (Figure 5d).
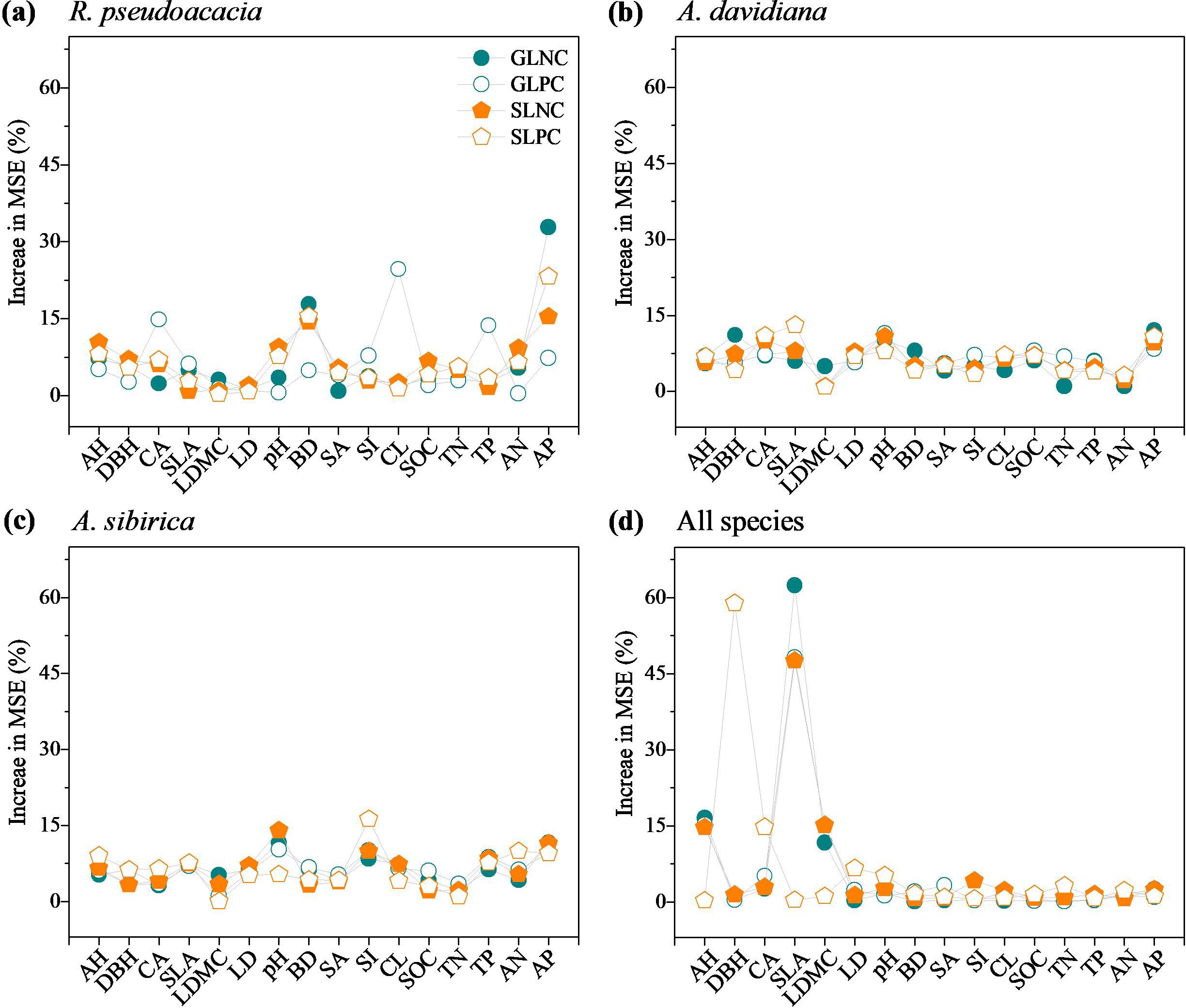
Figure 5. Random forest model analysis of the percentage increases in the mean square error (MSE, %) of variables in monocultures and mixed stands contributing to the changes in leaf nutrient concentrations of R. pseudoacacia (a), A. davidiana (b), A. sibirica (c), and all species (d). GLNC, green leaf nitrogen concentration; GLPC, green leaf phosphorus concentration; SLNC, senescent leaf nitrogen concentration; SLPC, senescent leaf phosphorus concentration; AH, average tree height; DBH, diameter at breast height; CA, crown area; SLA, specific leaf area; LDMC, leaf dry matter content; LD, leaf tissue density; pH, soil pH; BD, soil bulk density; SA, soil sand content; SI, soil silt content; CL, soil clay content; SOC, soil organic carbon concentration; TN, soil total nitrogen concentration; TP, soil total phosphorus concentration; AN, soil available nitrogen concentration; AP, soil available phosphorus concentration.
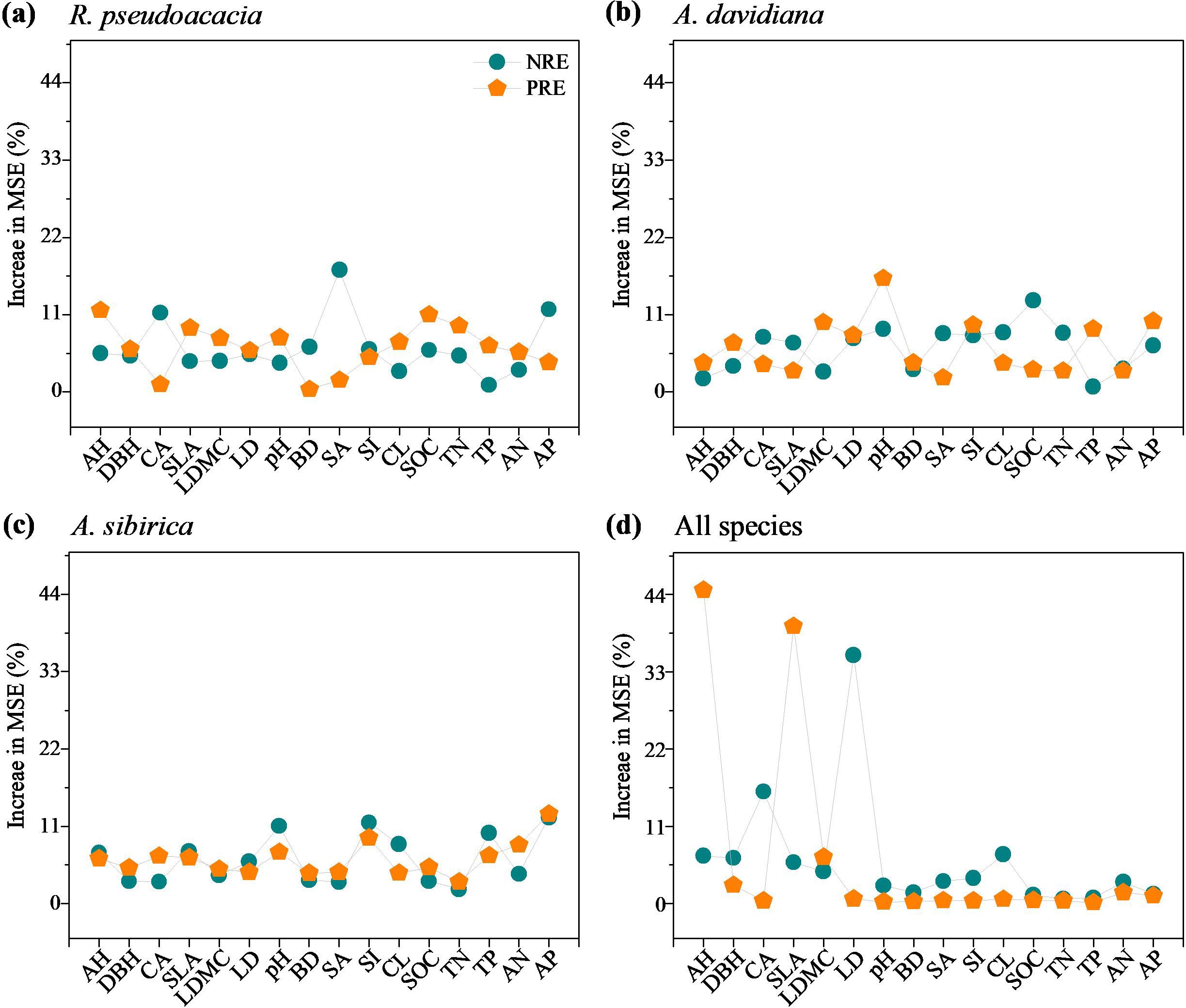
Figure 6. Random forest model analysis of the percentage increases in the mean square error (MSE, %) of variables in monocultures and mixed stands contributing to the changes in leaf nutrient resorption efficiencies of R. pseudoacacia (a), A. davidiana (b), A. sibirica (c), and all species (d). NRE, nitrogen resorption efficiency; PRE, phosphorus resorption efficiency; AH, average tree height; DBH, diameter at breast height; CA, crown area; SLA, specific leaf area; LDMC, leaf dry matter content; LD, leaf tissue density; pH, soil pH; BD, soil bulk density; SA, soil sand content; SI, soil silt content; CL, soil clay content; SOC, soil organic carbon concentration; TN, soil total nitrogen concentration; TP, soil total phosphorus concentration; AN, soil available nitrogen concentration; AP, soil available phosphorus concentration.
For individual species, soil sand content (17.36%) had large effects on the NRE of R. pseudoacacia, soil organic C concentration (13.1%) had effects on the NRE of A. davidiana, soil available P concentration (12.73%) had effects on the NRE of A. sibirica (Figures 6a–c). The average tree height (11.62%) had strong effects on the NRE of R. pseudoacacia, soil pH (16.15%) had great effects on the NRE of A. davidiana, soil available P (12.73%) concentrations had effects on NRE of A. sibirica. For all species, leaf dry matter content (35.34%) effected the NRE, and average tree height (44.64%) had effects on the PRE (Figure 6d).
3.5 Relationships between leaf nutrient concentrations and nutrient resorption efficiencies
Linear regression analysis revealed different correlations between leaf nutrient concentrations and nutrient resorption across individuals and all species (Figure 7). For specific species, the PRE of R. pseudoacacia was significantly positively correlated with the GLNC (P < 0.05, Figure 7b), and the opposite was true between the NRE and SLNC (P < 0.05, Figure 7c). The NREs of A. davidiana and A. sibirica were significantly negatively correlated with GLNC and SLNC (P < 0.05; Figures 7a, c) and were the same between PRE and SLPC (P < 0.05, Figure 7d). For all species, PRE was significantly positively correlated with GLPC (P < 0.05, Figure 7b) and significantly negatively correlated with SLNC (P < 0.001, Figure 7d).
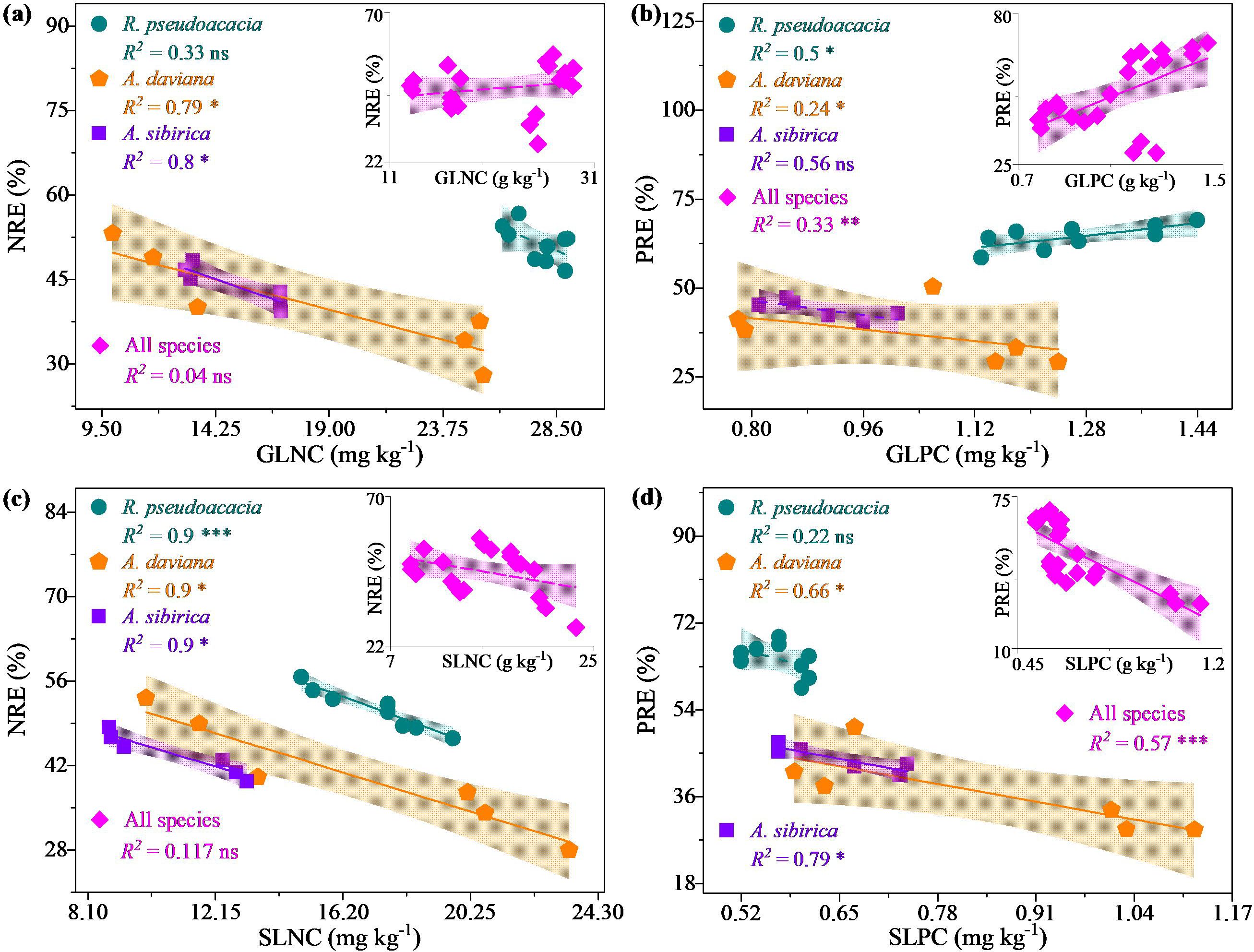
Figure 7. Linear regression analysis of the associations between nitrogen resorption efficiency (NRE) and green leaf nitrogen concentration (GLNC) (a), phosphorus resorption efficiency (PRE) and green leaf phosphorus concentration (GLPC) (b), NRE and senescent leaf nitrogen concentration (GLNC) (c), and PRE and senescent leaf phosphorus concentration (SLPC) (d) of specific and all species in monoculture and mixed stands. ***, P < 0.001; **, P < 0.01; *, P < 0.05; ns, P > 0.05.
4 Discussion
4.1 Leaf nutrient concentrations and their influencing factors
Previous studies have reported that N2-fixing species promoted the expansion of root networks and that mycorrhizal systems increase rhizobial activity, thereby increasing the concentration of soil available N (Forrester et al., 2006; Nygren and Leblanc, 2015; Yuan et al., 2016). Additionally, the decomposition of senescent leaves of R. pseudoacacia can further increase the N resources of A. davidiana and A. sibirica (Forrester et al., 2006; Voigtlaender et al., 2012; Paula et al., 2015). Thus, the soil total N concentration was higher in the mixed stands than in the monoculture stands (Supplementary Table S3). However, the GLNC and specific leaf area were not significantly positively correlated for R. pseudoacacia but were significantly positively correlated for A. davidiana and A. sibirica (Figures 4a–c), partially supporting Hypothesis 1. One reasonable explanation is that the canopy of mixed R. pseudoacacia is located above A. davidiana and A. sibirica, the decrease in light intensity is lower by the mixed R. pseudoacacia but higher for mixed A. davidiana and A. sibirica compared to mono species. Particularly, in the mixed stand, the light intensity of R. pseudoacacia was less affected by A. davidiana, while that of which was more affected by A. sibirica. Thus, the GLNC of R. pseudoacacia did not differ between the RPAD and RP stands, whereas lower in the RPAS than in the RP stand (Figure 1a), contrary to Hypothesis 1. These findings indicate that the GLNC of R. pseudoacacia is not only affected by the total N content of soil but also by the specific leaf area (Sanz-Perez et al., 2009; Liu et al., 2014; Zhu et al., 2020).
The GLPC of R. pseudoacacia was greater in the RPAD stand than in the RP stand, but there was no difference between the RPAS and RP stands (Figure 1b; Supplementary Table S4), which was partially consistent with Hypothesis 1. This could be involved stand characteristics and soil properties interact to affect the GLPC values of R. pseudoacacia in monoculture and mixed stands (Xu et al., 2020; Sardans et al., 2021). For instance, crown area, clay content, soil total P concentration were not significantly correlated with the GLPC values of R. pseudoacacia (Figure 4a), but they had great relative contributions to the GLPC of R. pseudoacacia (Figure 5a). Consistent with the fact that the GLPC values of A. davidiana and A. sibirica in mixed stands were significantly positively correlated with crown area (Figures 4b, c), the GLPC and crown area values of which were greater in the mixed plantations than in the monocultures (Figure 1b; Supplementary Table S4). It can be inferred that understory trees tend to allocate more P to increase leaf metabolic activity. Additionally, low soil sand content resulted in high soil porosity and water storage (Sardans et al., 2011; Metz et al., 2016), beneficial for root expansion and microbial activity (Nygren and Leblanc, 2015; Ma et al., 2017) and indirectly improves the rapid release of P from organic matter (Blank and Morgan, 2014; Coll et al., 2018; Jiang et al., 2019; Waithaisong et al., 2020). As a result, the soil sand content was lower in the RPAS stand than in the AS stand (Supplementary Table S3).
Decomposing senescent leaves play an important role in nutrient cycling in the plant–soil ecosystem. In particular, mixed plantings can increase the decomposition rate of senescent leaves and rapidly release nutrients into the topsoil through microenvironmental changes such as reduced soil temperature, increased soil moisture, and improved microbial activity (Wang et al., 2014; Paula et al., 2015; Lin et al., 2022). Therefore, the SLNC and SLPC did not differ between the RPAD and RP stands but were lower in the RPAS stand than in the RP stand for R. pseudoacacia (Figures 1c, d; Supplementary Table S4), whereas the soil total N and P concentrations were greater in the mixed plantations than in the monocultures (Supplementary Table S3). Additionally, senescent leaves with high nutrient concentrations can enhance soil nutrient availability by altering the amount of belowground N and P inputs (Forrester et al., 2006; Voigtlaender et al., 2012; Ma et al., 2017; Waithaisong et al., 2020). Thus, the SLNC and SLPC of A. davidiana and A. sibirica were greater in mixed plantations than in monocultures (Figures 1c, d), suggesting that senescent leaves of these mixed species than the monocultures returned more nutrients into the soil. However, whether the amounts of P released by the high-quality senescent leaves of A. davidiana and A. sibirica were much greater than that consumed by rhizobia from R. pseudoacacia is still unclear. First, mixed species growth under water stress conditions may not compete for soil available-P because of the strongly reduced mobility of soil P (Lambers et al., 2008). Second, tree structure can affect the nutrient concentration of senescent leaves in plants (Hayes et al., 2014; Lin et al., 2022). For example, the SLNC and SLPC of A. davidiana and A. sibirica in monocultures and mixed stands were positively associated with the average tree height and crown area (Figures 4b, c). It follows that more N and P elements were released from senescent leaves and transported into two shorter, non-N2-fixing species.
4.2 Leaf nutrient resorption efficiency and its influencing factors
In general, the process of N fixation by R. pseudoacacia rhizobacteria could increase soil available N concentration (Nygren and Leblanc, 2015). However, the difference in R. pseudoacacia NRE between pure and mixed stands was not significant (Figure 2a), inconsistent with Hypothesis 2. Thus, the mixed planted R. pseudoacacia was not changed its demand for N. However, the PRE of R. pseudoacacia was lower in the RP stand than in the RPAD stand, whereas it was not significantly different between the RP and RPAS stands (Figure 2b). A possible explanation is that: 1) less P consumed during the N fixation than the soil-available P that absorbed by R. pseudoacacia, 2) senescent leaves were released enough P for R. pseudoacacia, and 2) microclimate could improved the process (Aerts, 1996; Paula et al., 2015; Sardans et al., 2021). For this reason, the decomposition of senescent leaves may release more P to the soil in the RPAS stand than in the RPAD stand. However, the NRE and PRE of A. davidiana and A. sibirica were lower in mixed stands than in monocultures (Figure 2), consistent with Hypothesis 2. These findings suggest that the growth of A. davidiana and A. sibirica in the mixed plantation was less limited by soil N and P than that in the monoculture. Thus, mixed A. davidiana and A. sibirica tend to fully utilize N fixed by rhizobia and reduce NRE (Augusto et al., 2013; Paula et al., 2015; Bu et al., 2019) and therefore better adapted to nutrient-poor soils (Brant and Chen, 2015; Sardans et al., 2017). Additionally, the NRE and PRE of A. davidiana and A. sibirica were negatively correlated with crown area (Figures 4b, c), suggesting that the N and P in stems and branches were mainly obtained from the soil but less from reabsorbed nutrients by senescent leaves. Furthermore, the NRE of A. davidiana was negatively correlated with the soil total N concentration (Figure 4b), and the PRE of A. sibirica was negatively correlated with the soil total P concentration (Figure 4c). Therefore, in mixed plantations, A. davidiana releases N to the soil through senescent leaves, whereas A. sibirica releases P.
Consistent with Hypothesis 2, the relative nutrient rsorption efficiency (RRE) of R. pseudoacacia in pure and mixed plantations was < 100% (Figure 3a; Supplementary Table S4), which agree with the greater capacity of R. pseudoacaia to uptake and retain P during N fixation (Blank and Morgan, 2014; Liu et al., 2017). Thus, the growth of R. pseudoacacia was mostly limited by soil P (Reed et al., 2012; Han et al., 2013; Cao and Chen, 2017). Particularly, the growth of R. pseudoacacia was more easily limited by soil P in the RPAD stand than in the RP stand (Figure 3a; Supplementary Table S4). However, inconsistent with Hypothesis 2, A. davidiana and A. sibirica in the pure plantations presented values of RRE > 100%, which were not significantly different between the pure and mixed plantations (Figure 3a). These findings suggest that mixed planting of R. pseudoacacia did not significantly alleviate the N limitation imposed on A. davidiana or A. sibirica. This may be related to the fact that the availability of elemental N has a critical effect on the growth and photosynthesis of plants (Elser et al., 2010), especially the higher N requirements of A. davidiana and A. sibirica, which have larger crown areas in mixed plantations than in monocultures (Supplementary Table S2).
The NRE of individuals and all species were positively correlated with PRE (Figure 3b), consistent with previous studies (Han et al., 2013; Deng et al., 2019). Particularly, A. sibirica NRE and PRE were significantly positively correlated (Figure 3b), indicating the positive regulation of A. sibirica NuRE in pure and mixed plantations by soil N and P limitation. Thus, the N and P distributed in the green/senescent leaves of A. sibirica were derived mainly from the soil. However, the nutrient sources for the green leaves of R. pseudoacacia was also influenced by the rhizobial N fixation (Aerts, 1996; Forrester et al., 2006; Paula et al., 2015). Specifically, rhizobia during N fixation could consume large amounts of soil P, resulting in the differences between soil available N and P concentrations in the rhizosphere (Nygren and Leblanc, 2015; Yuan et al., 2016; Sardans et al., 2021). Thus, NRE and PRE were not significantly positively correlated for R. pseudoacacia in pure and mixed plantations (Figure 3b). However, NRE and PRE were significantly positively associated for all species (Figure 3b). Therefore, tree mixing is conducive to coordinating the relationship between NRE and PRE for different species through soil N and P availability, although its effect on N2- and non-N2-fixing species shows individual variation (Forrester et al., 2006; Coll et al., 2018).
4.3 Relationships between leaf nutrient concentrations and nutrient resorption efficiencies
Consistent with Hypothesis 3, the negative correlation between NRE and the GLNC of different plantations were not significant for R. pseudoacacia, but they were significant for A. davidiana and A. sibirica (Figure 7a). These findings suggest that rhizobial activity can significantly affect the NRE of R. pseudoacacia but not those of A. davidiana and A. sibirica. The reason for this may be that the mixed R. pseudoacacia maintains NRE by enhancing rhizobial N fixation and fully utilizes the fixed N, allowing A. davidiana and A. sibirica to benefit from the N fixation of R. pseudoacacia, which does not compete with the other plants for soil available N (Augusto et al., 2013; Bu et al., 2019; Chen and Chen, 2022). Additionally, the GLNC of mixed A. davidiana and A. sibirica can also originate from other sources, such as N deposition, available N and minerals. However, soil P is the main resource of the GLPC for different species (Blank and Morgan, 2014); thus, soil-available P is the main nutrient limiting plant growth, whether it is an N2-fixing species or not. Thus, the PRE and GLPC were positively associated in R. pseudoacacia but negatively associated in A. davidiana and all species (Figure 7b), indicating a difference in P uptake strategies between R. pseudoacacia and A. davidiana and A. sibirica. Sardans et al. (2012), Liu et al. (2017), and Li et al. (2018) reported that plant strategies for nutrient uptake were based on energy allocation to leaves and roots, with plants preferring to absorb more nutrients at a lower energy cost. On this basis, the energy cost of the green leaves of R. pseudoacacia was lower to retain P to compensate for soil P deficiency, whereas the energy cost of the roots of A. davidiana was lower during root nutrient uptake.
According to the theory of nutrient uplift, plants growth were mostly dependented on nutrients in the topsoil (Jobbagy and Jackson, 2001), and therefore the high nutrient concentrations in senescent leaves were associated with low nutrient resorption efficiency but high soil nutrient concentration (Aerts and Chapin, 1999; Sardans et al., 2017). Thus, the NRE of individual species was significantly negatively correlated with SLNC (Figure 7c), which is consistent with Hypothesis 3 and suggests an inverse control of SLNC on NRE. Furthermore, plants growing under high soil total N concentrations tend to withdraw a lower percentage of N from their leaves, resulted in more N returning to the topsoil via SLNC (Jobbagy and Jackson, 2001; Hayes et al., 2014; Brant and Chen, 2015). However, the negative relationship between PRE and SLPC was not significant for R. pseudoacacia, but the relationships were significant for A. davidiana and A. sibirica (Figure 7d), consistent with Hypothesis 3. These results indicate that other factors influence the process of P supply to R. pseudoacacia, such as P consumption by rhizobia during N fixation; however, tree mixture has a weak effect on P cycling in A. davidiana and A. sibirica (Sardans et al., 2012; Cierjacks et al., 2013). Therefore, the decomposition of senescent leaves can provide sufficient N for plant growth, but the P concentration does not meet the requirements of R. pseudoacacia, although it is sufficient for A. davidiana and A. sibirica (Jobbagy and Jackson, 2001; Ma et al., 2017).
Over, the results demonstrated that A. davidiana and A. sibirica are suitable mixing species to be planted with R. pseudoacacia to improve N and P cycling. In future forest management and ecosystem restoration planning, appropriate mixed species compositions and their effects on stand characteristics should be the first considerations to maintain the nutrient cycling of temperate forests.
5 Conclusions
In the Loess Hilly Region, Robinia pseudoacacia is often mixed with Amygdalus davidiana and Armeniaca sibirica to increase the ecological and economic benefits. However, how these plant mixtures affect the nutrient status of the individual species remains uncertain. The results revealed the variations in leaf N and P concentrations and resorption efficiencies between monocultures and mixed species and their relationships with influencing factors. The main conclusions are as follows:
1. The GLNC of R. pseudoacacia in mixed stands was influenced by specific leaf area and soil total N cocentration, whereas that of A. davidiana and A. sibirica more by soil total N concentration than specific leaf area. The GLPC of R. pseudoacacia in mixed plantations was influenced by the soil sand content and total P concentration; however, the higher GLPC in mixed plantations than in monocultures for A. davidiana and A. sibirica was influenced by the crown area, soil sand content, and senescent leaves. The SLNC and SLPC of A. davidiana and A. sibirica in monocultures and mixed stands were positively associated with the average tree height and crown area, suggests that amounts of N and P released from senescent leaves and transported into the non-N2-fixing species.
2. Mixed planting led to the variation in the PRE of R. pseudoacacia but decreased the NRE and PRE of A. davidiana and A. sibirica, because mixed R. pseudoacacia utilized more P during the N fixation, and the decomposition of senescent leaves supplied enough nutrients for A. davidiana and A. sibirica. Mixed planting did not significantly alleviated P limitation for R. pseudoacacia or N limitation for A. davidiana and A. sibirica because the N fixation process consumes P, and the greater crown areas of A. davidiana and A. sibirica require more N in mixed plantations than in monocultures. Regardless of the individual differences in the effects of the tree mixture on N2- and non-N2-fixing species, the coordinated association between NRE and PRE was still enhanced by the soil N and P concentrations.
3. In general, R. pseudoacacia prefers to reabsorb P by green leaves, whereas A. davidiana uptake nutrient to compensate for soil P deficiency. Rhizobacterial activity can significantly affect the NRE of R. pseudoacacia but not those of A. davidiana and A. sibirica. Decomposition of senescent leaves provides sufficient N and insufficient P for R. pseudoacacia and sufficient N and P for A. davidiana and A. sibirica.
Data availability statement
The original contributions presented in the study are included in the article/Supplementary Material. Further inquiries can be directed to the corresponding authors.
Author contributions
SL: Conceptualization, Data curation, Formal analysis, Investigation, Methodology, Resources, Software, Validation, Visualization, Writing – original draft. YC: Conceptualization, Data curation, Formal analysis, Funding acquisition, Investigation, Methodology, Project administration, Resources, Software, Supervision, Validation, Visualization, Writing – review & editing. JS: Conceptualization, Data curation, Methodology, Resources, Software, Supervision, Validation, Visualization, Writing – review & editing. JP: Conceptualization, Data curation, Methodology, Resources, Software, Supervision, Validation, Visualization, Writing – review & editing.
Funding
The author(s) declare that financial support was received for the research and/or publication of this article. This study was funded by the National Nature Science Foundation of China (No. 41771556). JS and JP were supported by the Spanish Government grants PID2020115770RB-I, PID2022-1408 08NB-I00, and TED2021-132627 B-I00 funded by MCIN, AEI/10.13039/501100011033 European Union Next Generation EU/PRTR, the Fundación Ramón Areces grant CIVP20A6621, and the Catalan Government grants SGR 2021-1333 and AGAUR2023 CLIMA 00118.
Conflict of interest
The authors declare that the research was conducted in the absence of any commercial or financial relationships that could be construed as a potential conflict of interest.
Generative AI statement
The author(s) declare that no Generative AI was used in the creation of this manuscript.
Publisher’s note
All claims expressed in this article are solely those of the authors and do not necessarily represent those of their affiliated organizations, or those of the publisher, the editors and the reviewers. Any product that may be evaluated in this article, or claim that may be made by its manufacturer, is not guaranteed or endorsed by the publisher.
Supplementary material
The Supplementary Material for this article can be found online at: https://www.frontiersin.org/articles/10.3389/fpls.2025.1527930/full#supplementary-material
Abbreviations
GLNC, green leaf nitrogen concentration; GLPC, green leaf phosphorus concentration; SLNC, senescent leaf nitrogen concentration; SLPC, senescent leaf phosphorus concentration; NRE, nitrogen resorption efficiency; PRE, phosphorus resorption efficiency; NuRE, nutrient resorption efficiency.
References
Aerts, R. (1996). Nutrient resorption from senescing leaves of perennials: are there general patterns? J. Ecol. 84, 597–608. doi: 10.2307/2261481
Aerts, R. and Chapin, F. S. (1999). The mineral nutrition of wild plants revisited: a re-evaluation of processes and patterns. Adv. Ecol. Res. 30, 1–67. doi: 10.1016/S0065-2504(08)60016-1
Augusto, L., Delerue, F., Gallet-Budynek, A., and Achat, D. L. (2013). Global assessment of limitation to symbiotic nitrogen fixation by phosphorus availability in terrestrial ecosystems using a meta-analysis approach. Global Biogeochem. Cy. 27, 804–815. doi: 10.1002/gbc.20069
Bao, S. (2000). Soil and agriculture chemistry analysis. 3rd ed. (Beijing: China Agriculture Press). (in Chinese).
Blank, R. R. and Morgan, T. (2014). Does a trend in declining stem density of Lepidium latifolium indicate a phosphorus limitation? A case study. Invasive Plant Sci. Manag. 7, 526–531. doi: 10.1614/IPSM-D-13-00103.1
Brant, A. N. and Chen, H. Y. H. (2015). Patterns and mechanisms of nutrient resorption in plants. Crit. Rev. Plant Sci. 34, 471–486. doi: 10.1080/07352689.2015.1078611
Bremner, J. M. and Mulvaney, C. S. (1982). “Nitrogen-total,” in Methods of soil analysis, part 2, chemical and microbiological properties, Agronomy Monograph, vol. 9. Ed. Page, A. L. (ASA and SSSA, Madison).
Bu, W. S., Chen, F. S., Wang, F. C., Fang, X. M., Mao, R., and Wang, H. M. (2019). The species-specific responses of nutrient resorption and carbohydrate accumulation in leaves and roots to nitrogen addition in a subtropical mixed plantation. Can. J. For Res. 49, 826–835. doi: 10.1139/cjfr-2018-0322
Cao, Y. and Chen, Y. M. (2017). Coupling of plant and soil C:N:P stoichiometry in black locust (Robinia pseudoacacia) plantations on the Loess Plateau, China. Trees 31, 1559–1570. doi: 10.1007/s00468-017-1569-8
Chen, X. L. and Chen, H. Y. H. (2022). Foliar nutrient resorption dynamics of trembling aspen and white birch during secondary succession in the boreal forest of central Canada. For Ecol. Manag. 505, 119876. doi: 10.1016/j.foreco.2021.119876
Chen, F. S., Niklas, K. J., Chen, G. S., and Guo, D. L. (2012). Leaf traits and relationships differ with season as well as among species groupings in a managed Southeastern China forest landscape. Plant Ecol. 213, 1489–1502. doi: 10.1007/s11258-012-0106-5
Chen, Y. P., Wang, K. B., Lin, Y. S., Shi, W. Y., and He, X. H. (2015). Balancing green and grain trade. Nat. Geosci. 8, 739–741. doi: 10.1038/ngeo2544
Cierjacks, A., Kowarik, I., Joshi, J., Hempel, S., Ristow, M., von der Lippe, M., et al. (2013). Biological flora of the British isles: Robinia pseudoacacia. J. Ecol. 101, 1623–1640. doi: 10.1111/1365-2745.12162
Coll, L., Ameztegui, A., Collet, C., Lof, M., Mason, B., Pach, M., et al. (2018). Knowledge gaps about mixed forests: what do european forest managers want to know and what answers can science provide? For Ecol. Manag. 407, 106–115. doi: 10.1016/j.foreco.2017.10.055
Delgado-Baquerizo, M., Maestre, F. T., Gallardo, A., Bowker, M. A., Wallenstein, M. D., Quero, J. L., et al. (2013). Decoupling of soil nutrient cycles as a function of aridity in global drylands. Nature 502, 672–676. doi: 10.1038/nature12670
Deng, J., Wang, S., Ren, C. J., Zhang, W., Zhao, F. Z., Li, X. F., et al. (2019). Nitrogen and phosphorus resorption in relation to nutrition limitation along the chronosequence of black locust (Robinia pseudoacacia L.) plantation. Forests 10, 261. doi: 10.3390/f10030261
Duan, B. L., Paquette, A., Juneau, P., Brisson, J., Fontaine, B., and Berninger, F. (2014). Nitrogen resorption in Acer platanoides and Acer saccharum: influence of light exposure and leaf pigmentation. Acta Physiologiae Plantarum 36, 3039–3050. doi: 10.1007/s11738-014-1674-x
Elser, J. J., Fagan, W. F., Kerkhoff, A. J., Swenson, N. G., and Enquist, B. J. (2010). Biological stoichiometry of plant production: Metabolism, scaling and ecological response to global change. New Phytol. 186, 593–608. doi: 10.1111/j.1469-8137.2010.03214.x
FAO (2020). Global forest resources assessment 2020: Key findings (Rome: FAO). doi: 10.4060/ca8753en
Forrester, D. I., Bauhus, J., Cowie, A. L., and Vanclay, J. K. (2006). Mixed-species plantations of Eucalyptus with nitrogen-fixing trees: A review. For Ecol. Manag. 233, 211–230. doi: 10.1016/j.foreco.2006.05.012
Han, W. X., Tang, L. Y., Chen, Y. H., and Fang, J. Y. (2013). Relationship between the relative limitation and resorption efficiency of nitrogen vs phosphorus in woody plants. PloS One 8, e83366. doi: 10.1371/journal.pone.0083366
Hayes, P., Turner, B. L., Lambers, H., and Laliberté, E. (2014). Foliar nutrient concentrations and resorption efficiency in plants of contrasting nutrient-acquisition strategies along a 2-million-year dune chronosequence. J. Ecol. 102, 396–410. doi: 10.1111/1365-2745.12196
Jiang, C., Zhang, H. Y., Wang, X. C., Feng, Y. Q., and Labzovskiig, L. (2019). Challenging the land degradation in China’s Loess Plateau: benefits, limitations, sustainability, and adaptive strategies of soil and water conservation. Ecol. Eng. 127, 135–150. doi: 10.1016/j.ecoleng.2018.11.018
Jobbagy, E. G. and Jackson, R. B. (2001). The distribution of soil nutrients with depth: global patterns and the imprint of plants. Biogeochemistry 53, 51–77. doi: 10.1023/A:1010760720215
Jucker, T., Bouriaud, O., and Coomes, D. A. (2015). Crown plasticity enables trees to optimize canopy packing in mixed-species forests. Func. Ecol. 29, 1078–1086. doi: 10.1111/1365-2435.12428
Kobe, R. K., Lepczyk, C. A., and Iyer, M. (2005). Resorption efficiency decreases with increasing green leaf nutrients in a global data set. Ecology 86, 2780–2792. doi: 10.1890/04-1830
Lamb, D., Erskine, P. D., and Parrotta, J. A. (2005). Restoration of degraded tropical forest landscapes. Science 310, 1628–1632. doi: 10.1126/science.1111773
Lambers, H., Chapin, F. S., and Pons, T. L. (2008). Plant physiological ecology. 2nd ed. (New York: Springer Science). doi: 10.1007/978-0-387-78341-3
Li, Q., Chen, X., and Zhou, D. (2018). Shoot nutrient content and nutrient resorption of Leymus chinensis in various legume mixtures. Front. Plant Sci. 9. doi: 10.3389/fpls.2018.01483
Lin, Y., Xia, C. K., Wu, G. Y., Wang, F. C., Wang, S. N., Liu, Y. Q., et al. (2022). Replanting of broadleaved trees alters internal nutrient cycles of native and exotic pines in subtropical plantations of China. For Ecosyst. 9, 100067. doi: 10.1016/j.fecs.2022.100067
Liu, C. L. C., Kuchma, O., and Krutovsky, K. V. (2018). Mixed-species versus monocultures in plantation forestry: development, benefits, ecosystem services and perspectives for the future. Glob. Ecol. Conserv. 15, e00419. doi: 10.1016/j.gecco.2018.e00419
Liu, J. X., Li, Y. Y., Xu, Y., Liu, S. G., Huang, W. J., Fang, X., et al. (2017). Phosphorus uptake in four tree species under nitrogen addition in subtropical China. Environ. Sci. pollut. Res. 24, 20005–20014. doi: 10.1007/s11356-017-9633-x
Liu, C. C., Liu, Y. G., Guo, K., Wang, S. J., and Yang, Y. (2014). Concentrations and resorption patterns of 13 nutrients in different plant functional types in the karst region of south-western China. Ann. Bot. 113, 873–885. doi: 10.1093/aob/mcu005
Ma, Z., Chen, H. Y. H., and Bellingham, P. (2017). Effects of species diversity on fine root productivity increase with stand development and associated mechanisms in a boreal forest. J. Ecol. 105, 237–245. doi: 10.1111/1365-2745.12667
Meng, Y. Y., Hou, B. W., Ding, C., Huang, L., Guo, Y. P., and Tang, Z. Y. (2023). Spatiotemporal patterns of planted forests on the Loess Plateau between 1986 and 2021 based on Landsat NDVI time-series analysis. GIsci. Remote Sens. 60, 2185980. doi: 10.1080/15481603.2023.2185980
Metz, J., Annighofer, P., Schall, P., Zimmermann, J., Kahl, T., Schulze, E. D., et al. (2016). Site-adapted admixed tree species reduce drought susceptibility of mature European beech. Glob. Change Biol. 22, 903–920. doi: 10.1111/gcb.13113
Murphy, J. and Riley, J. P. (1962). A modified single solution method for the determination of phosphate in natural waters. Anal. Chim. Acta 27, 31–36. doi: 10.1016/S0003-2670(00)88444-5
Nelson, D. W. and Sommers, L. E. (1982). “Total carbon, organic carbon and organic matter,” in Methods of Soil Analysis, Part 2. Eds. Page, A. L., Rh, M., and Keeney, D. R. (American Society of Agronomy, Madison), 539–579.
Nygren, P. and Leblanc, H. A. (2015). Dinitrogen fixation by legume shade trees and direct transfer of fixed N to associated cacao in a tropical agroforestry system. Tree Physiol. 35, 134–147. doi: 10.1093/treephys/tpu116
Paula, R. R., Bouillet, J. P., Trivelin, P. C. O., Zeller, B., Gonçalves, J. L. M., Nouvellon, Y., et al. (2015). Evidence of short-term belowground transfer of nitrogen from acacia mangium to eucalyptus grandis trees in a tropical planted forest. Soil Boil. Biochem. 91, 99–108. doi: 10.1016/j.soilbio.2015.08.017
Payn, T., Carnus, J. M., Freer-Smith, P., Kimberley, M., Kollert, W., Liu, S. R., et al. (2015). Changes in planted forests and future global implications. For Ecol. Manag. 352, 57–67. doi: 10.1016/j.foreco.2015.06.021
Peñuelas, J., Poulter, B., Sardans, J., Ciais, P., Velde, M., Bopp, L., et al. (2013). Human-induced nitrogen–phosphorus imbalances alter natural and managed ecosystems across the globe. Nat. Commun. 4, 2934. doi: 10.1038/ncomms3934
R Core Team (2022). R: A Language and Environment for Statistical Computing. Version 4.2.2 (Vienna, Austria: R. Foundation for Statistical Computing).
Reed, S. C., Townsend, A. R., Davidson, E. A., and Cleveland, C. C. (2012). Stoichiometric patterns in foliar nutrient resorption across multiple scales. New Phytol. 196, 173–180. doi: 10.1111/j.1469-8137.2012.04249.x
Sanz-Perez, V., Castro-Diez, P., and Millard, P. (2009). Effects of drought and shade on nitrogen cycling in the leaves and canopy of Mediterranean quercus seedlings. Plant Soil 316, 45–56. doi: 10.1007/s11104-008-9757-3
Sardans, J., Bartrons, M., Margalef, O., Gargallo-Garriga, A., Janssens, I. A., Ciais, P., et al. (2017). Plant invasion is associated with higher plant-soil nutrient concentrations in nutrient-poor environments. Global Change Biol. 23, 1282–1291. doi: 10.1111/gcb.13384
Sardans, J., Janssens, I. A., Ciais, P., Obersteiner, M., and Peñuelas, J. (2021). Recent advances and future research in ecological stoichiometry. Perspect. Plant Ecol. 50, 125611. doi: 10.1016/j.ppees.2021.125611
Sardans, J., Rivas-Ubach, H., and Peñuelas, J. (2011). Factors affecting nutrient concentration and stoichiometry of forest trees in Catalonia (NE Spain). For Ecol. Manag. 262, 2024–2034. doi: 10.1016/j.foreco.2011.08.019
Sardans, J., Rivas-Ubach, A., and Peñuelas, J. (2012). The elemental stoichiometry of aquatic and terrestrial ecosystems and its relationships with organismic lifestyle and ecosystem structure and function: a review and perspectives. Biogeochemistry 111, 1–39. doi: 10.1007/s10533-011-9640-9
Stewart, J. R., Kennedy, G. J., Landes, R. D., and Dawson, J. O. (2008). Foliar-nitrogen and phosphorus resorption patterns differ among nitrogen-fixing and non-fixing temperate-deciduous trees and shrubs. Int. J. Plant Sci. 169, 4. doi: 10.1086/528749
Sun, X., Kang, H., Chen, H. Y., Berg, B., Bartels, S. F., and Liu, C. (2015). Biogeographic patterns of nutrient resorption from Quercus variabilis Blume leaves across China. Plant Biol. 18, 505–513. doi: 10.1111/plb.12420
Tsunekawa, A., Liu, G., Yamanaka, N., and Du, S. (2014). Restoration and development of the degraded loess plateau, China (Springer).
Tully, K. L., Wood, T. E., Schwantes, A. M., and Lawrence, D. (2013). Soil nutrient availability and reproductive effort drive patterns in nutrient resorption in. Pentaclethra macroloba Ecol. 94, 930–940. doi: 10.1890/12-0781.1
Vergutz, L., Manzoni, S., Porporato, A., Novais, R. F., and Jackson, R. B. (2012). Global resorption efficiencies and concentrations of carbon and nutrients in leaves of terrestrial plants. Ecol. Monogr. 82, 205–220. doi: 10.1890/11-0416.1
Voigtlaender, M., Laclau, J. P., Gonçalves, J. L. M., Piccolo, M. C., Moreira, M. Z., Nouvellon, Y., et al. (2012). Introducing Acacia mangium trees in Eucalyptus grandis plantations: consequences for soil organic matter stocks and nitrogen mineralization. Plant Soil 352, 99–111. doi: 10.1007/s11104-011-0982-9
Waithaisong, K., Robin, A., Mareschal, L., Bouillet, J., Laclau, J., Deleporte, P., et al. (2020). Introducing n2-fixing trees (Acacia mangium) in eucalypt plantations rapidly modifies the pools of organic p and low molecular weight organic acids in tropical soils. Sci. Total Environ. 742, 140535. doi: 10.1016/j.scitotenv.2020.140535
Wang, M., Murphy, M. T., and Moore, T. R. (2014). Nutrient resorption of two evergreen shrubs in response to long-term fertilization in a bog. Oecologia 174, 365–377. doi: 10.1007/s00442-013-2784-7
Xu, M. P., Zhong, Z. K., Sun, Z. Y., Han, X. H., Ren, C. J., and Yang, G. H. (2020). Soil available phosphorus and moisture drive nutrient resorption patterns in plantations on the Loess Plateau. For Ecol. Manag. 461, 117910. doi: 10.1016/j.foreco.2020.117910
Yuan, Z. Q., Yu, K. L., Epstein, H., Fang, C., Li, J. T., Liu, Q. Q., et al. (2016). Effects of legume species introduction on vegetation and soil nutrient development on abandoned croplands in a semi-arid environment on the Loess Plateau, China. Sci. Total Environ. 541, 692–700. doi: 10.1016/j.scitotenv2015.09.108
Zhang, H., Guo, W., Yu, M., Wang, G. G., and Wu, T. (2018). Latitudinal patterns of leaf N, P stoichiometry and nutrient resorption of Metasequoia glyptostroboides along the eastern coastline of China. Sci. Total Environ. 618, 1–6. doi: 10.1016/j.scitotenv.2017.11.030
Zhou, L. L., Addo-Danso, S. D., Wu, P. F., Li, S. B., Zou, X. H., Zhang, Y., et al. (2016). Leaf resorption eficiency in relation to foliar and soil nutrient concentrations and stoichiometry of Cunninghamia lanceolata with stand development in southern China. J. Soil Sediment 16, 1448–1459. doi: 10.1007/s11368-016-1352-2
Keywords: mixed species, leaf N and P concentrations, nutrient resorption efficiency, stand characteristics, soil properties
Citation: Lu S, Chen Y, Sardans J and Peñuelas J (2025) Impact of mixed plantation strategies on the nutrient concentrations of green and senescent leaves and their nutrient resorption efficiencies in temperate forests of the Loess Hilly Region. Front. Plant Sci. 16:1527930. doi: 10.3389/fpls.2025.1527930
Received: 27 November 2024; Accepted: 11 March 2025;
Published: 13 May 2025.
Edited by:
Mehrdad Zarafshar, Linnaeus University, SwedenReviewed by:
Raquel Lobo-do-Vale, University of Lisbon, PortugalMahya Tafazoli, Sari Agricultural Sciences and Natural Resources University, Iran
Copyright © 2025 Lu, Chen, Sardans and Peñuelas. This is an open-access article distributed under the terms of the Creative Commons Attribution License (CC BY). The use, distribution or reproduction in other forums is permitted, provided the original author(s) and the copyright owner(s) are credited and that the original publication in this journal is cited, in accordance with accepted academic practice. No use, distribution or reproduction is permitted which does not comply with these terms.
*Correspondence: Yunming Chen, eW1jaGVuQG1zLmlzd2MuYWMuY24=; Senbao Lu, c2VuYmFvMjAyMUBud2FmdS5lZHUuY24=