- 1Institute for Bee Protection, Julius Kühn-Institute (JKI) – Federal Research Centre for Cultivated Plants, Braunschweig, Germany
- 2Zoological Institute, Technische Universität Braunschweig, Braunschweig, Germany
- 3Wageningen Plant Research, Business Unit Bioscience, Wageningen University and Research, Wageningen, Netherlands
- 4Université de Toulouse, Institut National de Recherche pour l’Agriculture, l’Alimentation et l’Environnement (INRAE) Unité Mixte de Recherche (UMR) Le Laboratoire des Interactions Plantes-Microbes-Environnement (LIPME), Castanet-Tolosan, France
Sunflower, Helianthus annuus L., is a prominent global oilseed crop with rising cultivation and appeal as a bee-friendly plant by providing abundant floral resources for pollinators. Mass-flowering crops can increase the availability of resources, and sunflower is a good opportunity to relieve pollen scarcity during the late summer in agricultural landscapes. Yet this should be taken with caution as they also provide a homogeneous source of nutrition. This study aimed to review and summarize the nutritional profile of sunflower pollen, nectar, bee bread, and honey, while assessing their effects on bee survival, development, and health. Furthermore, we present here the general state of knowledge on additional pollinator syndromes that extend beyond floral resources, including those influencing pollinator visual and olfactory attraction. We found that while sunflower pollen’s nutritional quality is questioned due to lower protein and amino acid deficiencies, its nutrient content, like nectar sugars, had large variability. Sunflower pollen consumption showed mixed effects on Apis mellifera and Bombus species, sometimes negatively impacting development and survival. However, studies have conveyed a positive impact on bee health as sunflower pollen consistently reduced the infection intensity of the gut parasite, Crithidia bombi, in Bombus species. This probes the question on defining the quality of floral resources, emphasizing the need for caution when categorizing sunflower as a low quality nutritional resource. This review also outlines the importance of sunflower nectar characteristics (sugar content and volume) and floral morphology (flower pigmentation and corolla length) on pollinator foraging preferences. A prominent knowledge gap persists regarding nectar chemistry and sunflowers’ extensive volatile profile to better understand the pollination syndromes that drive its pollinator interactions.
1 Introduction
Floral resource availability and nutritional composition play an essential role in pollinators’ development and survival. Our understanding of bee nutrition and foraging behaviors encompasses mainly honey bees, Apis mellifera (Haydak, 1970; Tsuruda et al., 2021; Winston, 1987) and more recently bumble bees, Bombus spp (Goulson, 2003; Prŷs-Jones and Corbet, 2011). Less is known regarding the species-specific balance between macro- and micronutrients and in regards to wild bee species. The honey bees’ diet consists mainly of pollen and nectar, including their stored products in the hive known as bee bread and honey. Pollen and bee bread are the primary sources of nutrition in the form of proteins, lipids, vitamins, and minerals that are crucial for normal growth and development, reproduction, brood rearing, and health (Winston, 1987). Bee bread differs from freshly collected pollen as it is stored in the hive with the addition of nectar or honey; various salivary enzymes; and diverse bacterial species, some responsible for natural fermentation (Ghosh et al., 2022; Winston, 1987). Nectar fulfils the essential energetic resource requirements, primarily through glucose, fructose, and sucrose needed for foraging and flight (Winston, 1987). Honey, stored nectar, is similarly consumed for its carbohydrates. Honey is characterized by its high sugar concentration (~80%); diverse sugar spectrum dependent on the nectar’s floral origin; bee-secreted enzymes; and antibacterial properties (Crane, 1975; Molan, 1992). All bee collected and processed products might be applied and shared within the hive for adult bee and larvae medication (self-medication, allo-medication), which means they are consumed for disease prevention or therapy, to cure infected individuals or boosting the immune system (Erler and Moritz, 2016; Erler et al., 2024). This hypothesis has been proven experimentally for several cases using honey bees and bumble bees of different species (see sections 3.1 and 3.3 of this manuscript).
The nutritional breakdown of pollen and nectar, and subsequently bee bread and honey, varies depending on the plant species (Pamminger et al., 2019a, b; Stephen et al., 2024). It is under current debate how to determine a nutritionally balanced diet for different pollinators (Bryś and Strachecka, 2024; Vaudo et al., 2024), yet this remains difficult when their specific nutritional requirements are still uncertain. The traditional concept, basing the quality of a pollinator’s diet solely on the crude protein and essential amino acid content, has been scrutinized for general validity. Rather, it might be essential to consider other factors such as protein:lipid ratios which may impact foraging decisions (Vaudo et al., 2020) and stoichiometric ratios of mineral nutrients (Filipiak et al., 2017). A rising topic in bee health research is the role of plant secondary metabolites ingested by bees from pollen, nectar, honey, and bee bread, including alkaloids, polyphenols, terpenoids, and many others, but our knowledge is limited to honey bees and bumble bees (Barberis et al., 2023). Secondary metabolites have shown to boost cognitive functioning (Baracchi et al., 2017) and immunity (Palmer-Young et al., 2017); or even restrict development (Rivest et al., 2024). Secondary metabolites can shape insect immunity against parasites and pathogens (Erler et al., 2024; Fitch et al., 2022). These metabolites can have direct effects on the host's immune system or exert antimicrobial effects independently, thereby indirectly enhancing insect fitness. We are just at the beginning of understanding how these metabolites may interact with pollinators.
It is acknowledged that mono-diets, consisting of a single pollen source, are not considered well-balanced and social bees need a mix of plant sources to balance the nutrients that are missing in some sources, but present in others, to maintain healthy colonies (Alaux et al., 2010; Di Pasquale et al., 2013, 2016). A mixed pollen diet is presumable also important for generalist solitary species, however research is lacking. Modern agricultural practices, monoculture, and pollen scarcity, especially in late summer, may be a key factor in the combined stressors impacting seasonal survival and contributing to pollinator decline (Balvino-Olvera et al., 2024; Di Pasquale et al., 2016; Dolezal et al., 2019; Donkersley et al., 2014; Harris et al., 2024; Lau et al., 2023; Requier et al., 2015, 2017; Wright et al., 2024). Mass-flowering crops can increase the availability of resources for flower-visiting insects in agricultural environments (Di Pasquale et al., 2016; Harris et al., 2024; Holzschuh et al., 2013; Westphal et al., 2003), especially sunflower (Helianthus annuus L.) which blooms during the critical summer period (Requier et al., 2015, 2017). The impact of mass-flowering crops should be put into perspective since they only offer a homogeneous resource over a limited period (Di Pasquale et al., 2013, 2016; Goulson et al., 2015; Potts et al., 2010, 2016). This makes it important to understand in greater detail the nutritional needs of pollinators, the nutritional value that large-scale flowering crops can provide, and how it may impact the development and health of pollinators.
Sunflower is one such late-flowering, large scale crop surpassing 29 Mha and seed production reaching 54 million tons in 2022 (FAO, 2024). Across Europe, the share of sunflower production in agricultural landscapes is primarily in Eastern and Southern regions, such as Ukraine, Romania, Bulgaria, France, and Spain (Figure 1). With the increasing demand of sunflower production and climate change, there is an expected shift in sunflower production towards Northern European regions. Sunflower production relies partly or completely on insect mediated pollen transfer for oilseed, confection and hybrid seed production (Chabert et al., 2022; Mallinger et al., 2019; Mallinger and Prasifka, 2017a; Oz et al., 2009). It is known to attract a diversity of wild and managed bees (references reviewed in Brown and Cunningham, 2019; Chabert et al., 2022), with higher diversity observed in North America, which is the native range of the Helianthus species (Heiser et al., 1969; Kantar et al., 2015; Mandel et al., 2011; Seiler and Jan, 2010).
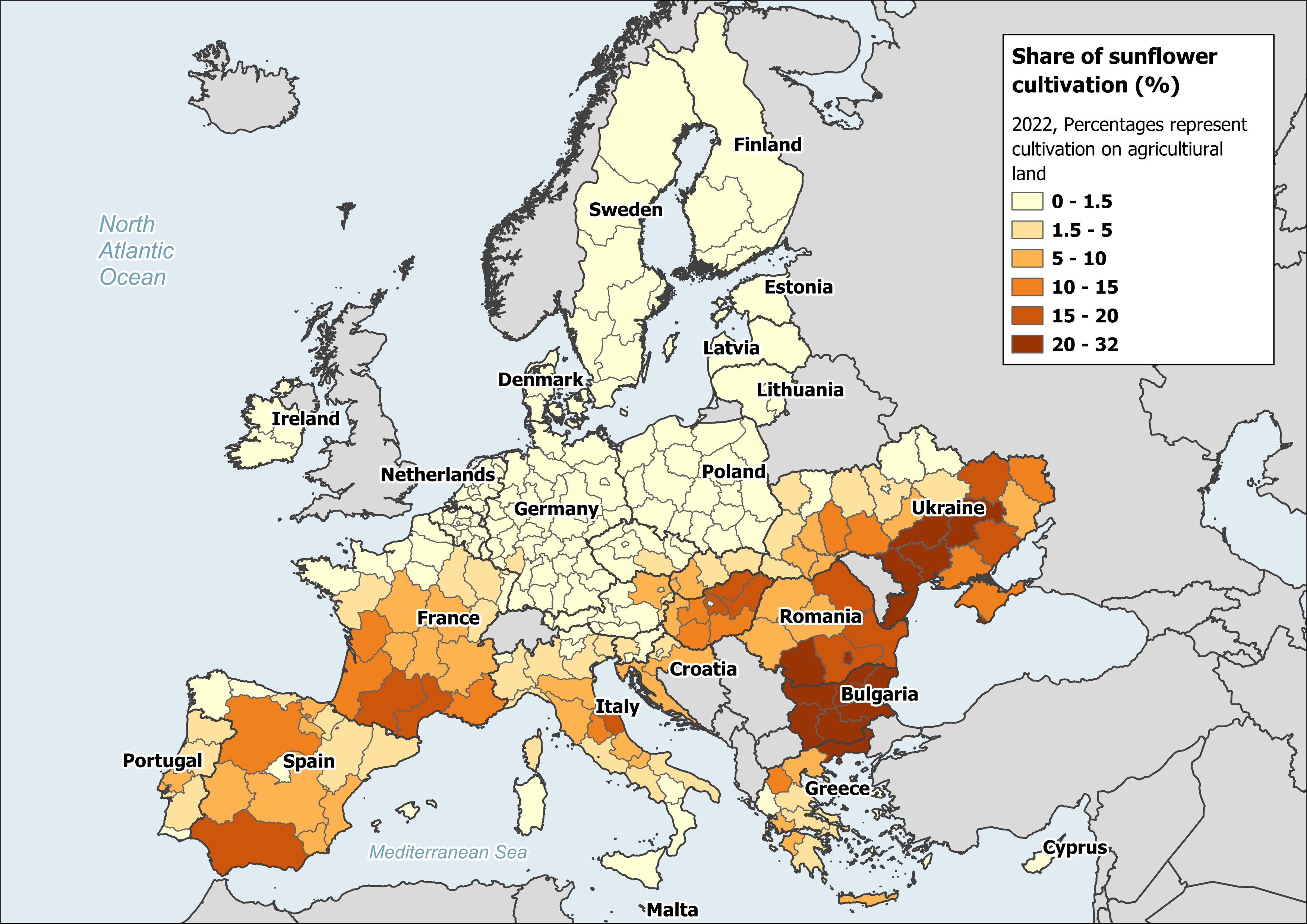
Figure 1. Share of sunflower cultivation on agricultural land in 2022. Source: Data extracted from European Commission, Joint Research Centre (JRC) (2022), for sunflower cultivation in EU 27 member states and Ukraine. (doi: 10.2905/555e5d1d-1aae-4320-a716-2e6d18aa1e7c).
Pollination syndromes is a general term used to describe floral traits mediating interaction between plants and pollinators (Knudsen and Tollsten, 1993). Pollen and nectar resources are called ‘rewards’ or ‘primary attractants’ (Armbruster, 2012; Shivanna, 2014) and as such were shown, with nectar access, to influence foraging preference in sunflowers; including preferences for different cultivars (Bergonzoli et al., 2022; Cerrutti and Pontet, 2016; Freund and Furgala, 1982; Mallinger and Prasifka, 2017b; Tepedino and Parker, 1982), nectar quantity (Mallinger and Prasifka, 2017b), nectar sucrose content (Pham-Delègue et al., 1990, 1994; but see Mallinger and Prasifka, 2017b), pollen production (Mallinger and Prasifka, 2017b), and nectar access through corolla length (Ferguson et al., 2021; Mallinger and Prasifka, 2017b; Portlas et al., 2018). Other flower traits are defined as ‘secondary attractants’, in the sense that they are cues associated with floral resources innately or through learning by bees (Armbruster, 2012; Frachon et al., 2021; Knauer and Schiestl, 2015; Ortiz et al., 2021; Shivanna, 2014; Wright and Schiestl, 2009). Such floral cues were associated with bee foraging preferences in sunflower, including floral volatile organic compounds (VOCs) (Pham-Delègue et al., 1986, 1990) and floral pigmentation (Todesco et al., 2022). This makes sunflower a particularly interesting crop which has gained renewed attention to enrich ecosystems by providing plentiful floral resources for bees in agricultural dominant landscapes. With the importance of sunflower in agricultural production and the substantial increase in cultivation, it is important to grasp the potential nutritional value of sunflower floral resources for pollinator communities and the pollinator syndromes which impact sunflower-pollinator interactions.
The aim of this review is to provide a foundation for sunflower nutritional research for pollinators and summarize the current state of knowledge on the chemistry of sunflower floral resources (pollen and nectar) and processed hive products (honey and bee bread). We also provide a comprehensive overview on targeted sunflower research investigating floral resource consumption on bee survival, development, and health. Furthermore, our review encompasses pollinator syndromes beyond floral rewards, examining additional factors that may shape pollinator attraction and foraging preferences in sunflowers. By doing so, this review seeks to identify future research directions and knowledge gaps to improve our understanding of sunflower plant-pollinator interactions.
2 Chemistry of sunflower floral resources, bee bread, and honey
2.1 Pollen
Sunflowers are often considered a “low-quality” resource since the crude protein content falls on the lower scale (Nicolson and Human, 2013; Pamminger et al., 2019a). Bee collected sunflower pollen protein content shows large variance ranging from 7.32–19.4 g/100 g, excluding the outlier 0.49 g/100 g from Stephen et al. (2024). Interestingly, hand-collected pollen had a higher range (11.6–26.5 g/100 g) (Table 1). In general, Asteraceae species crude protein has been reported in lower ranges (Vaudo et al., 2024), between 12–14% (Roulston et al., 2000) and 5.2–23.7% (Vaudo et al., 2020). In any case, valuing quality based on protein content alone should be taken with caution as wide ranges for crude protein values exists even for commonly reported “high-quality” pollen sources. For example, the crude protein from Brassica spp. pollen was reported between 22.2–33.8% by Pamminger et al. (2019a) but only 13.6% by Vaudo et al. (2020).
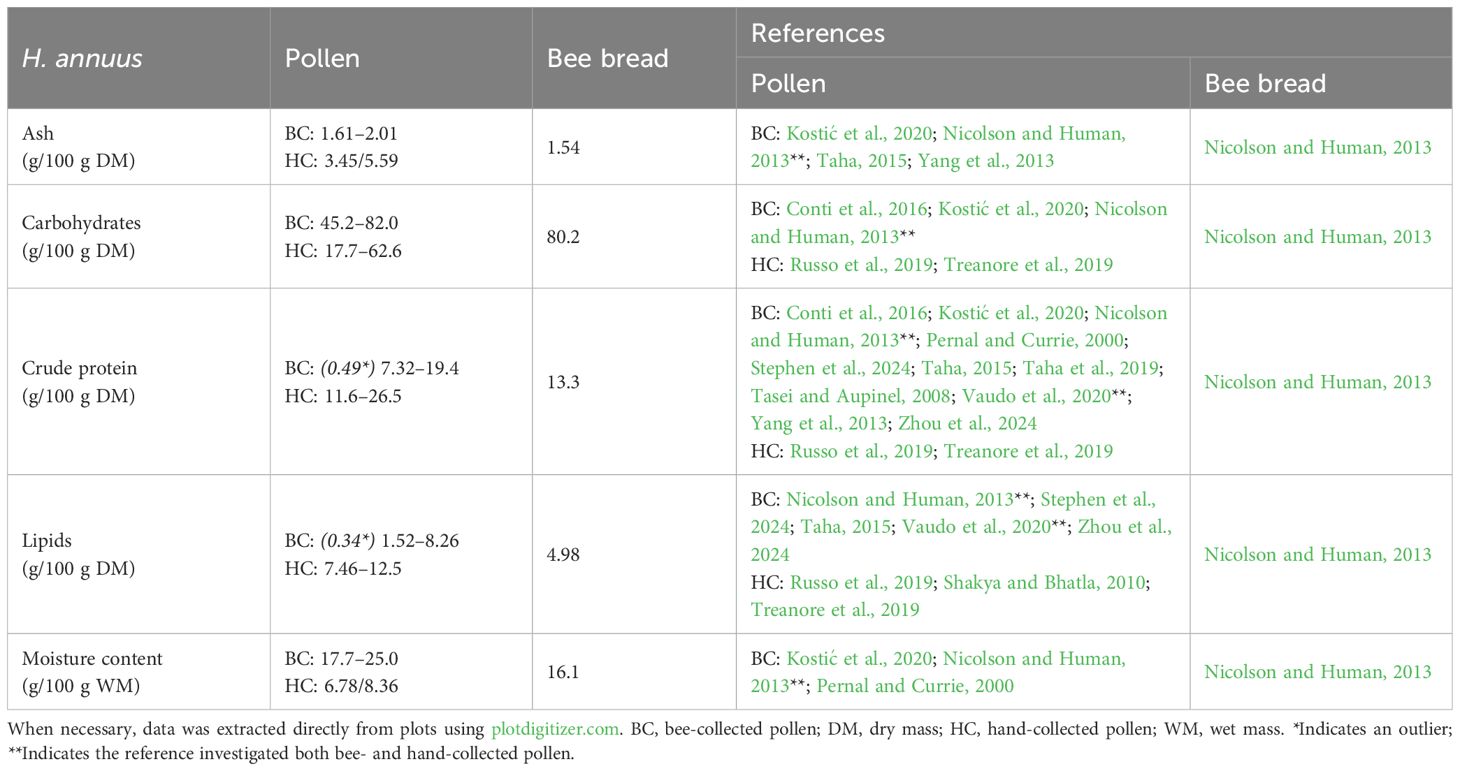
Table 1. Literature values of compositional parameters reported for Helianthus annuus bee- and hand-collected pollen and bee bread.
Amino acid profiles are important to consider in terms of protein quality. Based on the essential amino acid requirements for honey bees detailed in De Groot (1953), sunflower pollen was reported to have below required amounts of isoleucine, methionine, and tryptophan; and occasional deficiencies in arginine and phenylalanine (Table 2). It is not uncommon for pollen to have deficiencies in one or more amino acids, as it is the case for plant species within the Fabaceae and Boraginaceae families (Jeannerod et al., 2022). This review summarized only protein-bound amino acids to limit variability, since free amino acids may more readily be influenced or lost by different methods of pollen handling. Therefore, the amino acid content is not the absolute content available to bees, as other studies have shown that free amino acids contribute an additional 3.49–6.06% depending on species (McAulay and Forrest, 2019). It is also known that amino acids vary for the same species depending on analysis method (Jeannerod et al., 2022). Abiotic conditions, such as fertilization levels, were also found to be positively correlated with total amino acid content in pollen (Leach et al., 2021). Therefore, values should be interpreted with caution as the deficient amino acids may not be realistic in all natural settings, especially when free amino acid availability and other environmental factors may impact composition.
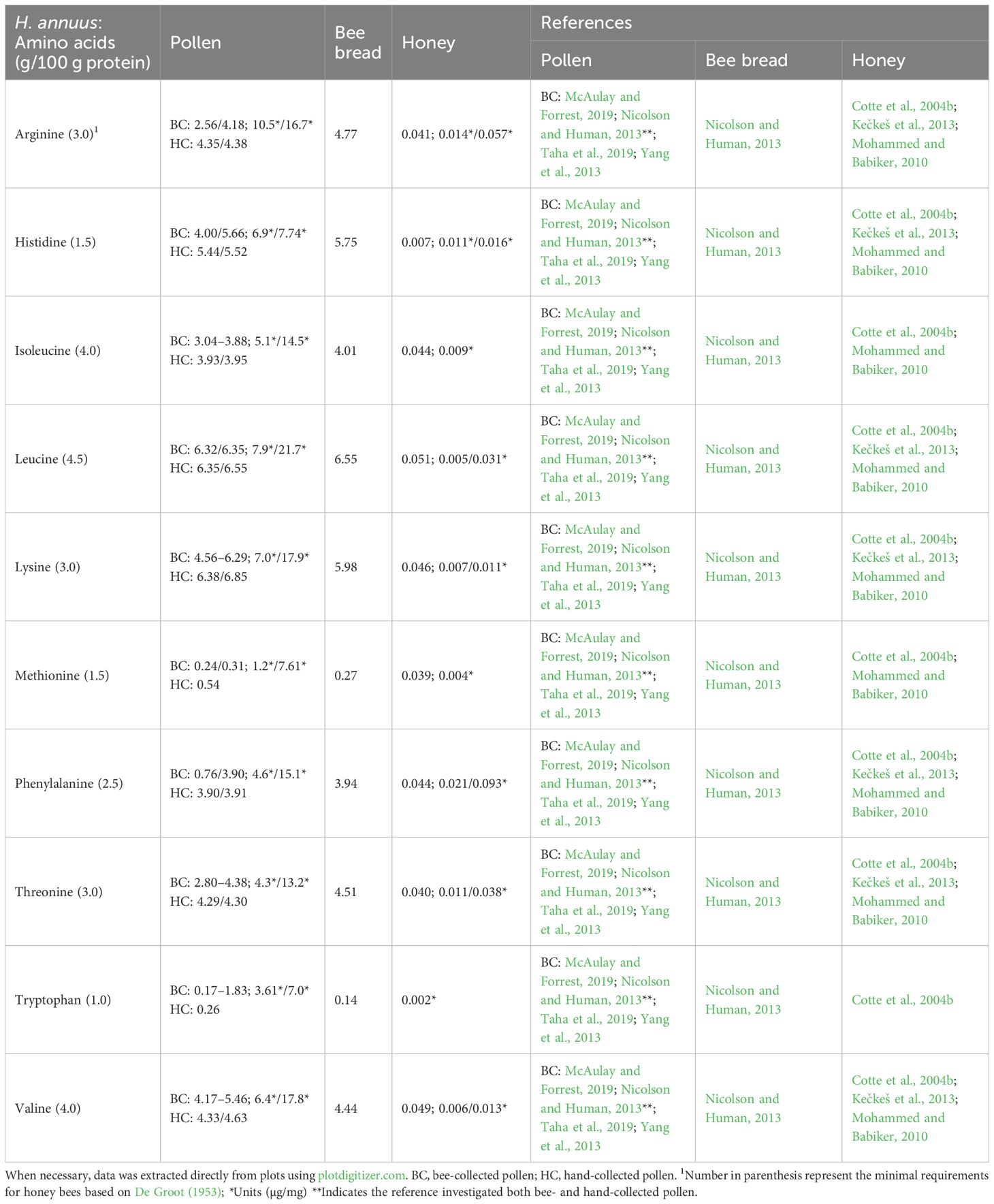
Table 2. Literature values for amino acids reported in Helianthus annuus bee- and hand-collected pollen, bee bread, and honey.
Pollen lipid content is another important parameter to consider for bee nutrition. Not all lipids are synthesized endogenously (Arien et al., 2018; Winston, 1987), therefore it is vital to investigate their detailed composition obtained in bees’ diets. The lipid content of bee-collected sunflower pollen (Table 1), is reported within the range 1.52–8.26%, excluding the outlier 0.34% from Stephen et al. (2024). Sunflower lipid content falls on the lower end of the typical range (1.5–24.6%) found in bee-pollinated crops (Vaudo et al., 2020). Schulz et al. (2000) conducted a detailed analysis of lipid compounds in sunflower pollen and detailed 35% terpenes and terpene esters, 17% diketones, 8% fatty acids (primarily 11-eicosenoic acid), 8% ketols, 12% diols, 4% dioxoalkanoic acids, 12% esters, 2% alkanes, and 2% others. Only a few studies analyzed, in detail, the profile of fatty acid content. Sunflower pollen differed in their distributions of individual fatty acids (Table 3). For example, stearic acid content was recorded at 1.72% (Nicolson and Human, 2013), but was also measured at 31.4% (Kostić et al., 2020). Palmitic acid had a similar variability between studies. Furthermore, α-linolenic acid, is the predominant unsaturated fatty acid (20.5–42.1%) found in sunflower pollen. In fact, sunflower pollen was reported to have a significantly greater content of linoleic and α-linolenic acid compared to almond and mixed pollen sources (Yokota et al., 2024). Sterols (i.e., β-sitosterol and 24-methylene-cholesterol) are another important group as they are not synthesized endogenously and are required to be ingested via bees’ pollen diet (Winston, 1987). Farag et al. (1980) identified the presence of cholesterol, stigmasterol, and β-sitosterol in sunflower pollen. More specifically, Takatsuto and Omote (1989) identified isofucosterol (42%), β-sitosterol (20.4%), 24-methylene-cholesterol (18.1%), 24-methylene-cholestanol (12.5%), and 23-dehydrocholesterol (4%). They identified six more phytosterols but they were all less than 1%. More recent studies on sterol identification and composition are lacking.
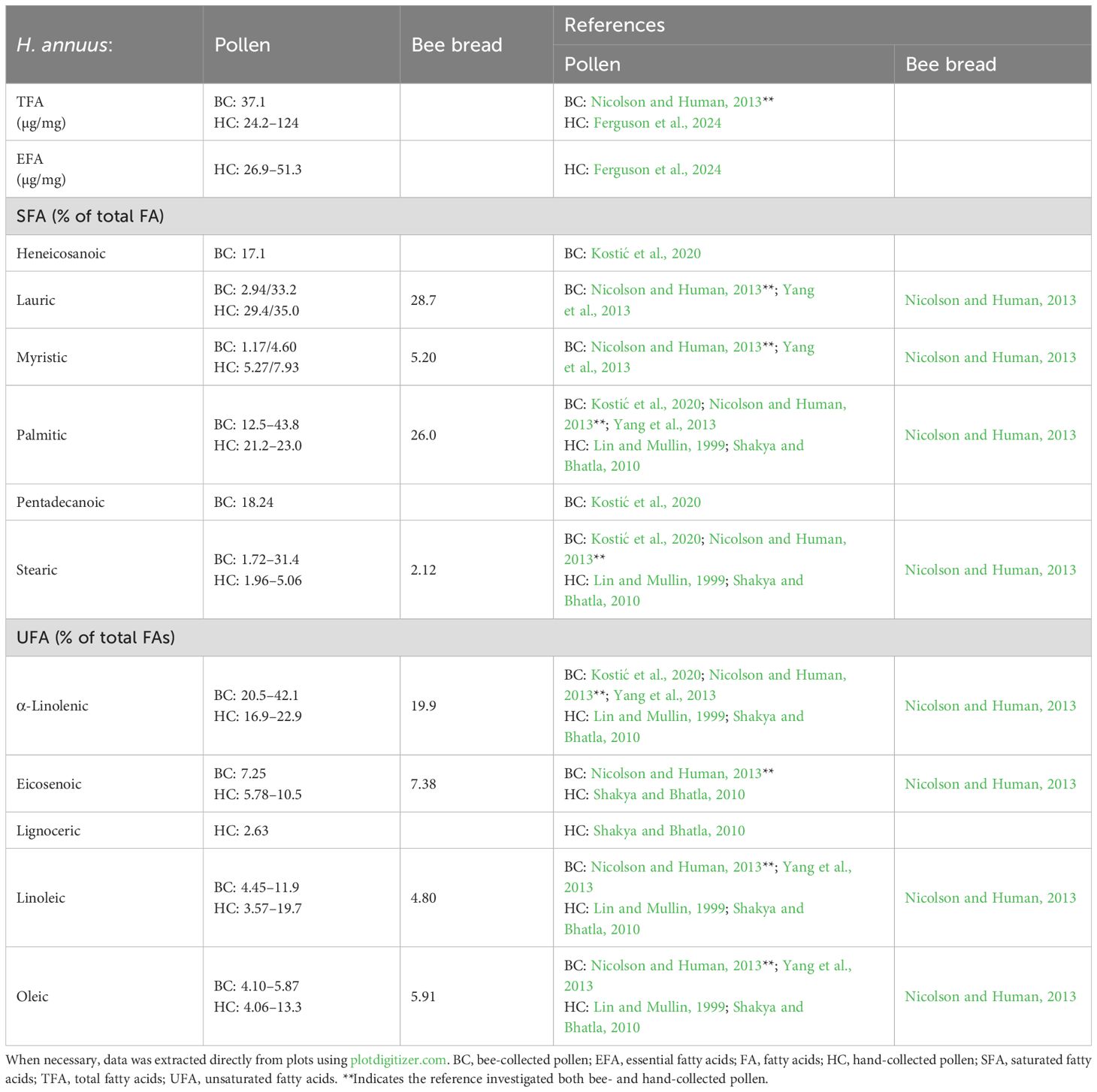
Table 3. Literature values for fatty acids reported in Helianthus annuus bee- and hand-collected pollen and bee bread.
In addition to key macronutrients, pollen offers other important micronutrients, including minerals and plant secondary metabolites. Mineral nutrients are important molecular compounds needed for essential roles in physiological function, and 12 minerals (C, N, P, K, S, Ca, Mg, Na, Zn, Fe, Mn, and Cu) have been considered as biologically significant elements (Filipiak, 2019; Lau et al., 2022). In total, 17 minerals, including heavy metals, were reported present in sunflower pollen (Table 4). Most reported mineral nutrients had a high degree of variability. Ca, K, and P were consistently reported as major minerals in multiple studies (> 1000 mg/kg), yet they still had ranges varying 2-fold.
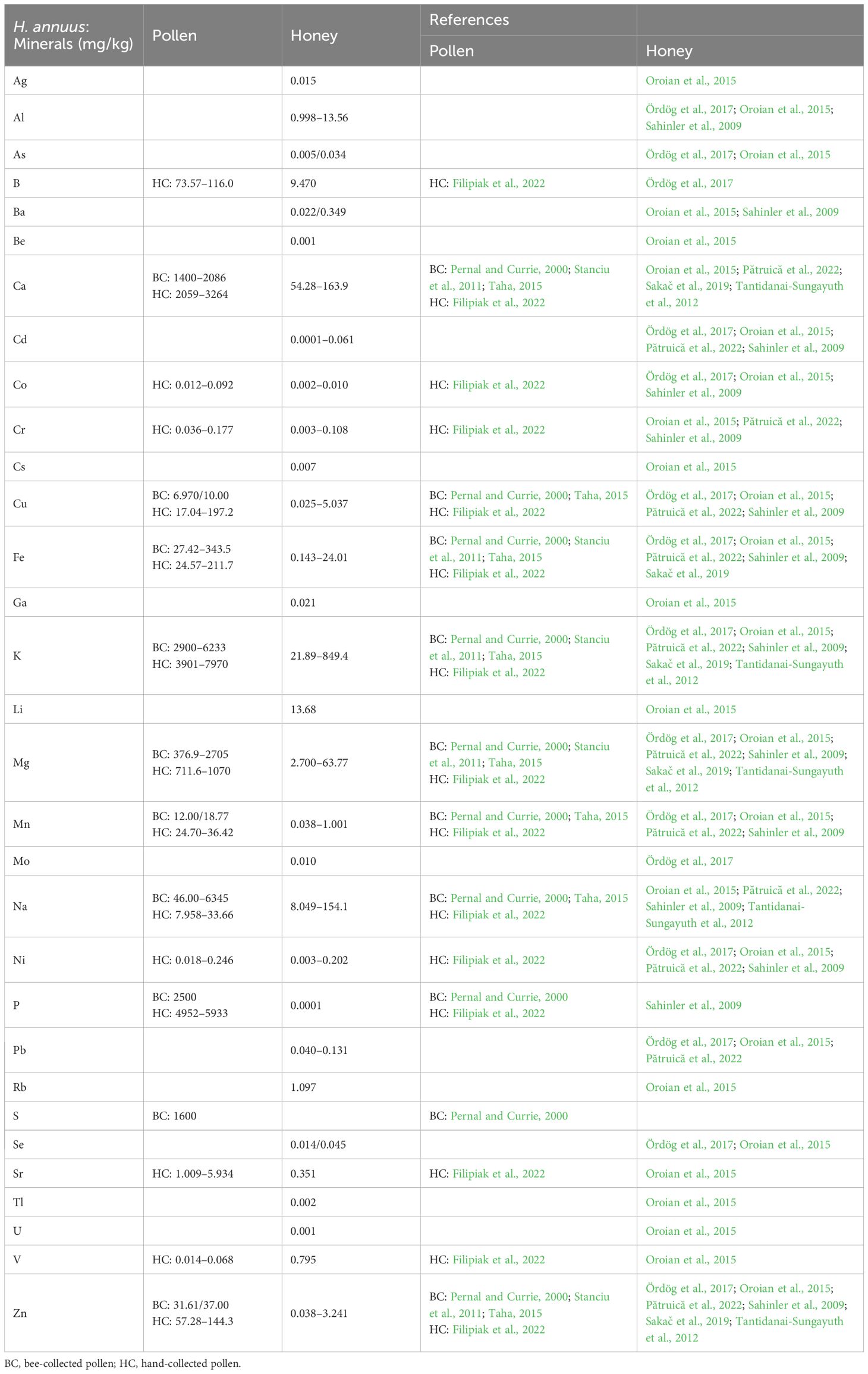
Table 4. Literature values for mineral content in Helianthus annuus bee- and hand-collected pollen and honey.
The composition of plant secondary metabolites in pollen is becoming a popular topic due to their potential role in plant-pollinator interactions, bee physiology, and bee health. Sunflower pollen consists mainly of phenolamides, primarily tricoumaroyl spermidine, a diverse array of flavonoids, and phenolic acids (Table 5). Among phenolamides, sunflower pollen contains fewer types overall but is particularly rich in different spermidine isomers, including high levels of dicoumaroyl spermidine and tricoumaroyl spermidine (Qiao et al., 2023; Zhang et al., 2022). Flavonoids and phenolic acids are other major groups of metabolites present in sunflower pollen, particularly compounds such as apigenin, luteolin, kaempferol, quercetin glycosides, ferulic acid, and p-coumaric acid have been consistently reported (Table 5). In diverse samples of pollen from various floral resources, flavonoids accounted for more than half of the 467 total polyphenols (Rocchetti et al., 2019), and the predominant flavonoids between different floral pollen samples were mainly quercetin, isorhamnetin, and kaempferol glycosides (Qiao et al., 2023). It is important to keep in mind that the overview presented in this review does not indicate the specific isomers of each metabolite present. In fact, Qiao et al. (2023) found that the phenolics and flavonoids present were always cis/trans isomers and not in the free form. Whether this may have an impact on biological activity of these metabolites and may further influence plant-pollinator interactions is beyond the scope of this review. However, it is an interesting question that could arise when understanding the full picture of nutrition combined with how chemical variances could impact managed and wild bees’ foraging preferences.
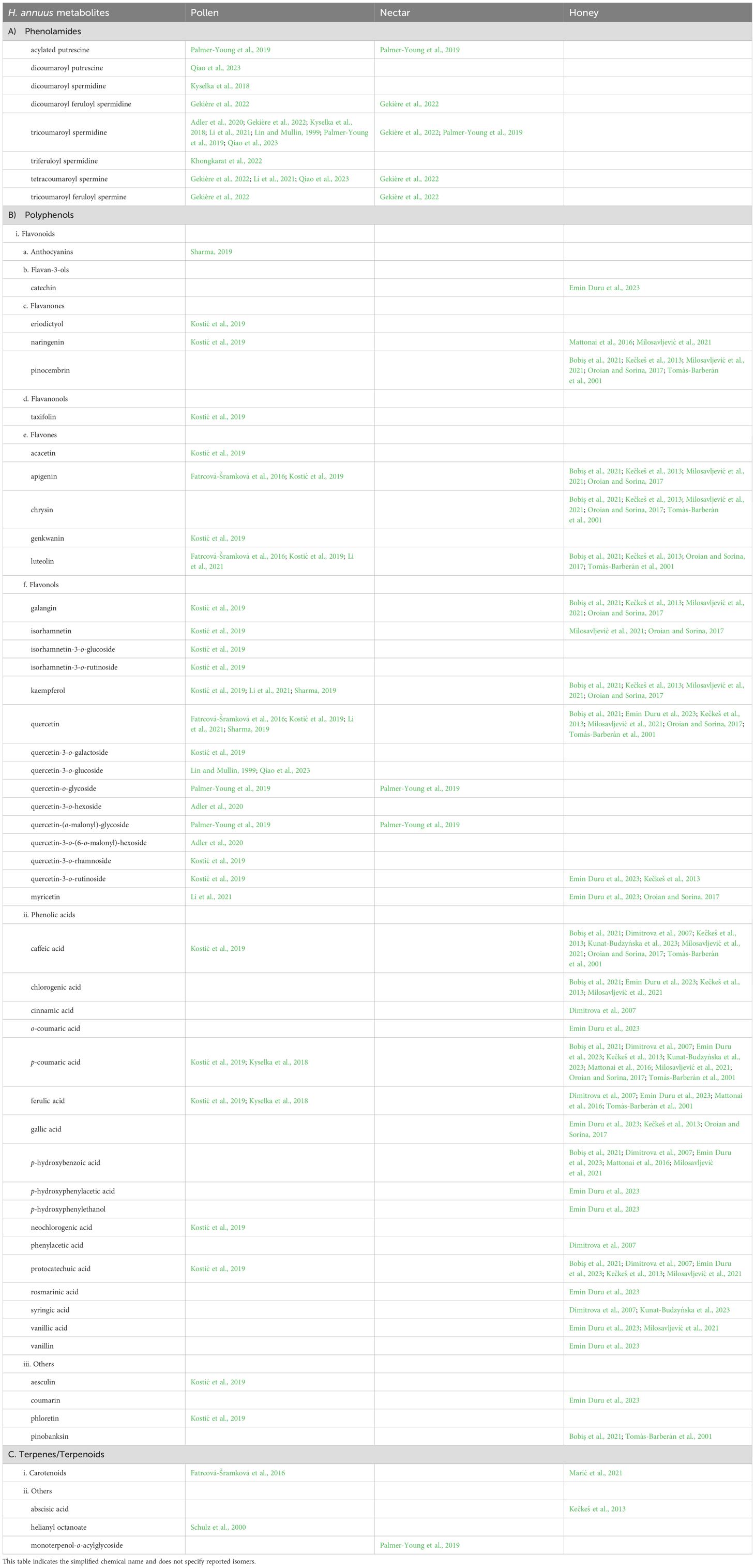
Table 5. Identified plant secondary metabolites reported in the literature from Helianthus annuus pollen, nectar, and honey.
In general, both macro- and micronutrients in sunflower pollen is highly variable, making it difficult to establish a complete picture of its nutritional profile. Inconsistency exists between studies and could be due to differences in methods used for chemical analysis (e.g., Westreich and Tobin, 2021), pollen handling and storage, intraspecific variation, and/or due to an environmental × genotype interaction of the pollen producing plant. For example, pollen protein content of Brassica varied from 8.9–31.3% depending on if the pollen was intact or disrupted (Lau et al., 2022). Additionally, a meta-analysis by Ruedenauer et al. (2019), found that variation of pollen nutrients did not clearly correlate with phylogenetic relatedness. Zimmermann et al. (2017) found that pollen of Poa alpina and P. hybrida had higher levels of proteins and P. hybrida had higher carbohydrates in warmer compared to colder climates. Ultimately, with more extensive and reliable chemical analysis and synergistic protocols (e.g., Villagómez et al., 2023), future research could address if a unique physiochemical profile for sunflower pollen in different locations and cultivars could be determined. This would be immensely beneficial to predict the nutritional availability in sunflower cultivation environments for neighboring pollinator populations.
2.2 Nectar
Nectar chemistry studies are largely focused on sugar contents, as sugar is one of the major attractants to pollinators and is the primary energy source for foraging bees. Sunflower nectar volume and sugar mass per floret are strikingly variable across studies. Nectar volume was reported to range from 0.002–0.59 μl/floret in the staminate (male) floret stage and from 0.00–1.13 μl/floret in the pistillate (female) floret stage (Table 6). Sugar mass was also reported to range from 34.0–216 μg sugar/floret in the staminate stage and from 23.3–491 μg sugar/floret in the pistillate stage (Table 6). In terms of individual sugars, sunflower nectar is generally characterized by higher glucose (46.0–50.1%) and fructose (49.9–54.0%), and lower sucrose contents, yet some genotypes have exhibited high sucrose contents reaching up to 62% (Aquino et al., 2021; Mallinger and Prasifka, 2017b; Pham-Delègue et al., 1990, 1994; Prasifka et al., 2023; Vear et al., 1990) (Table 6).
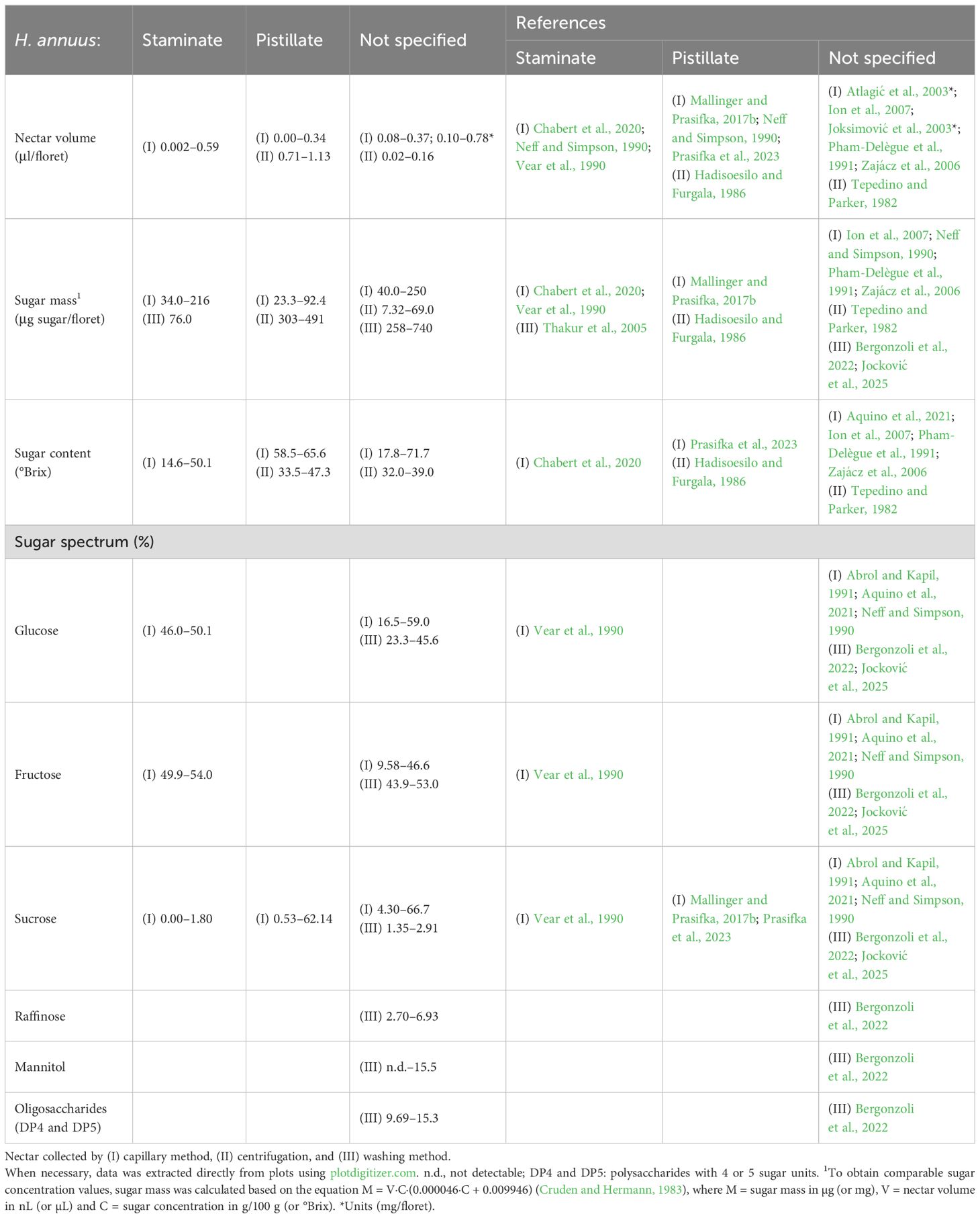
Table 6. Literature values for volume and sugar characteristics of nectar collected from different floret stages (staminate, pistillate, or not specified) of Helianthus annuus.
High variance reported for nectar volume and sugar content comes with no surprise as many studies have suggested that these characteristics are strongly influenced by abiotic factors and genotype. Studies have shown that floret stage (staminate vs. pistillate) (Chabert et al., 2020; Hadisoesilo and Furgala, 1986), vapor pressure deficit (Chabert et al., 2020), time of day (Chabert et al., 2020; Neff and Simpson, 1990; Thakur et al., 2005), temperature (Chabert et al., 2020; Prasifka et al., 2023), and fertilization treatment (Bergonzoli et al., 2022) can impact nectar volume and/or sugar concentration. Furthermore, during a long term evaluation of sunflower hybrids (2002–2010), Terzić et al. (2017) found that the growing condition caused the largest variation in nectar production. Nectar extraction, whether by centrifugation, washing method, or microcapillary method, is also suggested to influence nectar volume and sugar concentration, in which all three methods were conducted in the literature for sunflower nectar extraction (Table 6). For example, Mesquida et al. (1988) compared centrifugation and micropipette extraction for Brassica napus and found centrifugation artificially diluted the nectar samples by 4 to 6 times.
It is known that nectar contains many non-sugar metabolites, such as amino acids, lipids, vitamins, minerals, plant secondary metabolites, hormones, and proteins (Roy et al., 2017). Sunflower nectar chemistry in terms of non-sugar metabolites is lacking. It is not new knowledge that nectar contains amino acids (Baker and Baker, 1973). To the best of our knowledge, only one study identified the amino acid phenylalanine in sunflower nectar (Palmer-Young et al., 2019) and only a few studies investigated the secondary metabolite composition, in which they found various alkaloids, flavonoids, and terpenoids (Gekière et al., 2022; Palmer-Young et al., 2019) (Table 5). Noticeably, Helianthus nectar had a relatively high concentration of alkaloids compared to the other nectar sources (Palmer-Young et al., 2019). The composition of different secondary metabolites among plant structures has been found to vary. Palmer-Young et al. (2019) found that among nectar, flower, and pollen sources from 26 floral species, nectar contained the highest proportion of free amino acids and terpenoids. Nonetheless, it is important to emphasize that total amino acid content is still far higher in pollen (Nicolson, 2022) and some non-sugar metabolites in nectar might result from pollen contamination. However, Palmer-Young et al. (2019) reported that 18% of the metabolites in nectar were unique from those found only in pollen or shared between pollen and nectar. This presents an intriguing question regarding these unique nectar metabolites and whether they play a specific role in plant-pollinator interactions. This topic is almost unexplored for sunflower nectar.
Nectar is a complex topic, which is likely why we have yet to fully understand the extent of its components, mechanisms that govern its chemistry, and subsequent impacts on ecological interactions. Nectar chemistry contains more than just macro- and micronutrients, but it also contains nectar specific proteins (nectarins) in which a majority play key antimicrobial roles for nectar protection (Heil, 2011; Nepi, 2017; Roy et al., 2017). Recently, using LC-MS/MS-based comparative proteomic analysis, 144 proteins were identified in nectar of various species of Nicotiana (Silva et al., 2020). This study exemplifies how vast protein diversity is in nectar. They also found several proteins in nectar with roles in metabolic processes, such as regulating organic acids (malate dehydrogenase) and carbohydrate metabolism (sucrose synthase, fructokinase, and α-galactosidase). Thus, proteins in nectar impact its chemistry, which may influence its nutritional value for pollinators. Refocusing on sunflowers, unfortunately, no studies have identified or described their nectar proteins. Therefore, this would be an essential addition to the comprehensive view on the nutritional aspects of its floral resources and sunflower-pollinator interactions.
2.3 Bee bread
When considering honey bee nutrition, it is important to address both raw and processed floral resources. Sunflower bee bread is relatively understudied and only one study addressed its compositional parameters (Table 1), amino acids (Table 2), and fatty acids (Table 3) compared to fresh pollen. For example, Nicolson and Human (2013) found a significantly higher arginine concentration in bee bread, while all other macronutrient properties remained unchanged. In a separate study, Kaplan et al. (2016) analyzed sunflower bee bread and the protein content was 24.26 g/100 g, which falls on the higher end of pollen crude protein content. However, their bee bread samples contained only 45.4% H. annuus pollen. Whether the pollen nutritional value changes throughout the storage process is still controversial. Studies have shown no nutritional value differences (Herbert and Shimanuki, 1978; Fernandes-da-Silva and Serrão, 2000) or slightly decreased nutrition (Mayda et al., 2020). These studies did not compare fresh pollen and bee bread from the same plant source or sometimes failed to indicate the botanical origin, which is important to consider when discussing nutritional variance. Recently, Bayram et al. (2021) compared pollen and bee bread from similar plant sources and found a significantly higher concentration of protocatechuic acid, 2,5-dihydroxybenzoic acid and kaempferol in bee bread. Whereas, Degirmenci et al. (2024) and Mayda et al. (2020) found that the total phenolic content decreased. To the best of our knowledge, no studies have investigated mineral compounds and plant secondary metabolites specifically for sunflower bee bread. Similar to pollen content, it can be inferred that many factors can impact its chemical composition; for example, botanical origin, geographic location, climate, abiotic and biotic stressors, bee bread fermentation length, season, and even the presence and composition of the microbiome communities. There remains a significant gap in research comparing the compositional differences between pollen and bee bread and most research regarding such resource compositions are viewed through the human perspective. Whether these differences make raw or stored resources more nutritious for pollinators, especially bees, remains relatively unexplored.
2.4 Honey
Inside the honey bee hive, the conversion of nectar to honey offers many advantages as a stored energy and nutrient source. It serves to maintain colonies though the winter months and aids nurse bees with an in-hive source for their own care and for feeding the larval brood (Crane, 1975; Winston, 1987). When classifying honey, it is of course important to consider both the chemical and physical properties and potentially one of the most important factors is the nectar chemistry from where it originates (Crane, 1975). It is critical to consider that physicochemical properties of honey differ based on natural variation; thus the entire physical and chemical picture of honey are matched to the ‘ideal reference model’ of that botanical source (Persano Oddo and Bogdanov, 2004). The reference model of classifying honey has further challenges as the legislation for honey criteria and standards depend on the country (Thrasyvoulou et al., 2018). Sunflower honey has generally been described as having high glucose content (Cotte et al., 2004a; Juan-Borrás et al., 2014; Persano Oddo et al., 1995; Persano Oddo and Piro, 2004), proline, and acidity values (Persano Oddo and Piro, 2004). Pollen content of sunflower honey was also found with extreme variability from 20–90% (Persano Oddo and Piro, 2004). However, this could be explained by country regulation as Germany requires > 50% pollen content, whereas Greece requires > 20% (Thrasyvoulou et al., 2018).
In general, based on our compilation of sunflower honey studies, the general sugar content ranged from 73.1–80.8% and sixteen different sugars have been identified (Table 7). Threonine (Cotte et al., 2004b) and phenylalanine (Kečkeš et al., 2013) were also reported higher in sunflower honey compared to other honey types (Table 2), and K and Mg were higher compared to rapeseed and black locust honeys (Ördög et al., 2017) (Table 4). In contrast to pollen, nectar, and bee bread, many studies have investigated the specific secondary metabolites found in sunflower honey. The metabolite profile is diverse, primarily consisting of polyphenols, specifically flavonoids and phenolic acids (Table 5). The compounds identified varied between studies and sunflower honey samples. Mattonai et al. (2016) consistently found p-hydroxybenzoic acid, p-coumaric acid, ferulic acid, and naringenin in all sunflower honey samples. The overall concentration of flavonoids and phenolics (Table 8) had large ranges from 19.5–269 mg QE/100 g and 6.90–854 mg GAE/100 g, respectively. This also depended on the study as Pătruică et al. (2022) found higher concentration of phenolic acids in Romanian sunflower honey compared to other literature sources. As noted, honey is characterized by its’ antioxidant and antimicrobial properties, with the latter discussed in greater detail in a subsequent section. Interpreting honey’s antioxidant properties is challenging due to the lack of standardized methods and the use of various methodologies with differing units (Table 9). Castiglioni et al. (2017) observed that antioxidant levels varied depending on the free radical scavenging assay, such that some samples showing significant differences in ABTS but not in DPPH. This highlights the need for standardized procedures, as antioxidant values can only be relatively compared under consistent testing conditions.
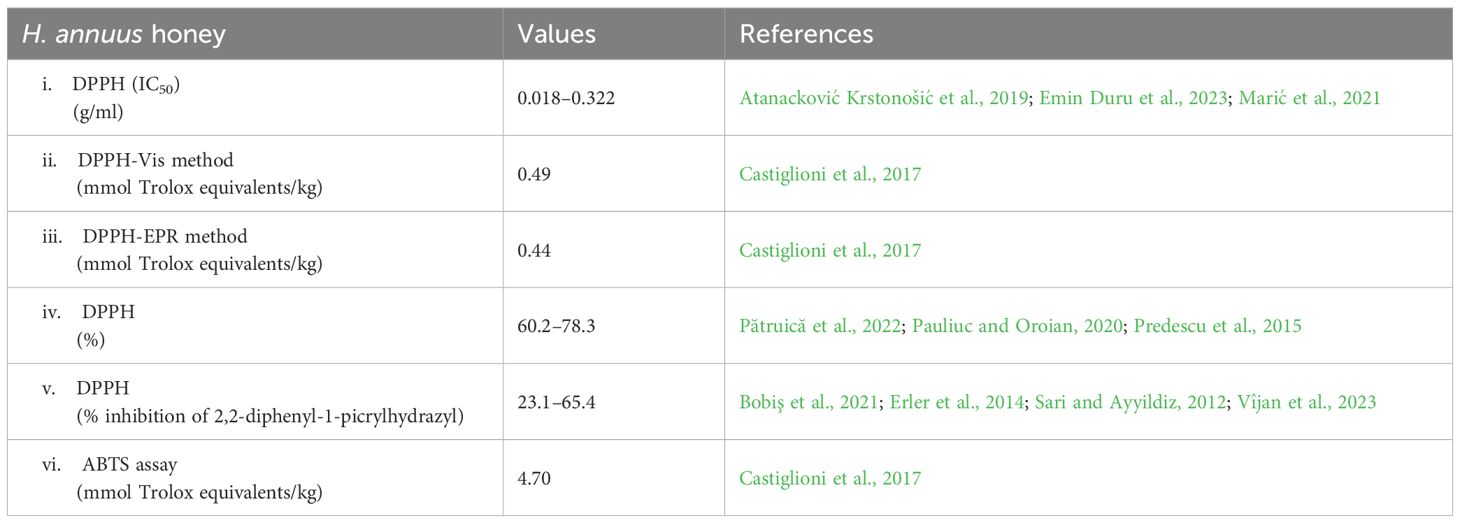
Table 9. Literature values of antioxidant capacity measured with various methods reported for Helianthus annuus honey.
As mentioned, summarizing sunflower honey’s characteristics is challenging due to the many physical and chemical properties considered in honey classification, inconsistencies reported across studies, and differing country legislations. Furthermore, honey characteristics are impacted by the botanical origin, year and their interaction (Vîjan et al., 2023); intra-sample variation (Mattonai et al., 2016), and climate (Kečkeš et al., 2013). It is also known that sugars in honey can be influenced by factors such as the ripening period and storage conditions (Crane, 1975). Many studies lack clarity regarding their processing methods, ripening duration, and storage conditions before measuring sugar characteristics; which further complicates the overall picture.
3 Sunflower as a nutritional resource for bee development and health
3.1 Pollen
The increasing cultivation of sunflowers across Europe highlights the need to understand their nutritional impact on pollinator communities and identify areas for further research. Sunflower pollen is often reported as a low quality nutritional resource for honey bees, as a result of lower levels of protein (Nicolson and Human, 2013; Pamminger et al., 2019a) and a few essential amino acids reported below minimum requirements for honey bees (Nicolson and Human, 2013; Taha et al., 2019). One commonly used method for testing the nutritional quality of pollen is done by using controlled feeding cage assays and are primarily focused on the western honey bee, Apis mellifera; the common eastern bumble bee, Bombus impatiens; and the buff-tailed bumble bee, B. terrestris, which is not surprising due to their economic importance, commercial rearing and availability. Figure 2 summarizes the results from literature investigating sunflower pollen diets on bee reproductive, development, and health parameters, including antiparasitic, antimicrobial, and antiviral effects. Cage experiments with a pure sunflower pollen diet often resulted in negative reproductive parameters for B. terrestris, including reduced mass and production of larvae and pupae; and reduced male emergence (Gekière et al., 2022; Regali and Rasmont, 1995; Tasei and Aupinel, 2008). In queen-right B. terrestris colonies, Zhou et al. (2024) found negative developmental effects in those fed sunflower and buckwheat pollen, including decreased worker mass and number, reduced wing length, and absence of a reproductive phase. On the contrary, one study investigated B. impatiens and found an increase in egg, larvae, and pupae production (Giacomini et al., 2018). Negative impacts on development were also observed by reduced fat body content in B. terrestris (Gekière et al., 2022) and reduced hypopharyngeal gland protein content or volume in A. mellifera (Omar et al., 2017; Pernal and Currie, 2000). However, varying results were found for worker ovary development within A. mellifera and B. impatiens. Even more interesting was the extensive inconsistency of worker survival within and between the investigated bee species.
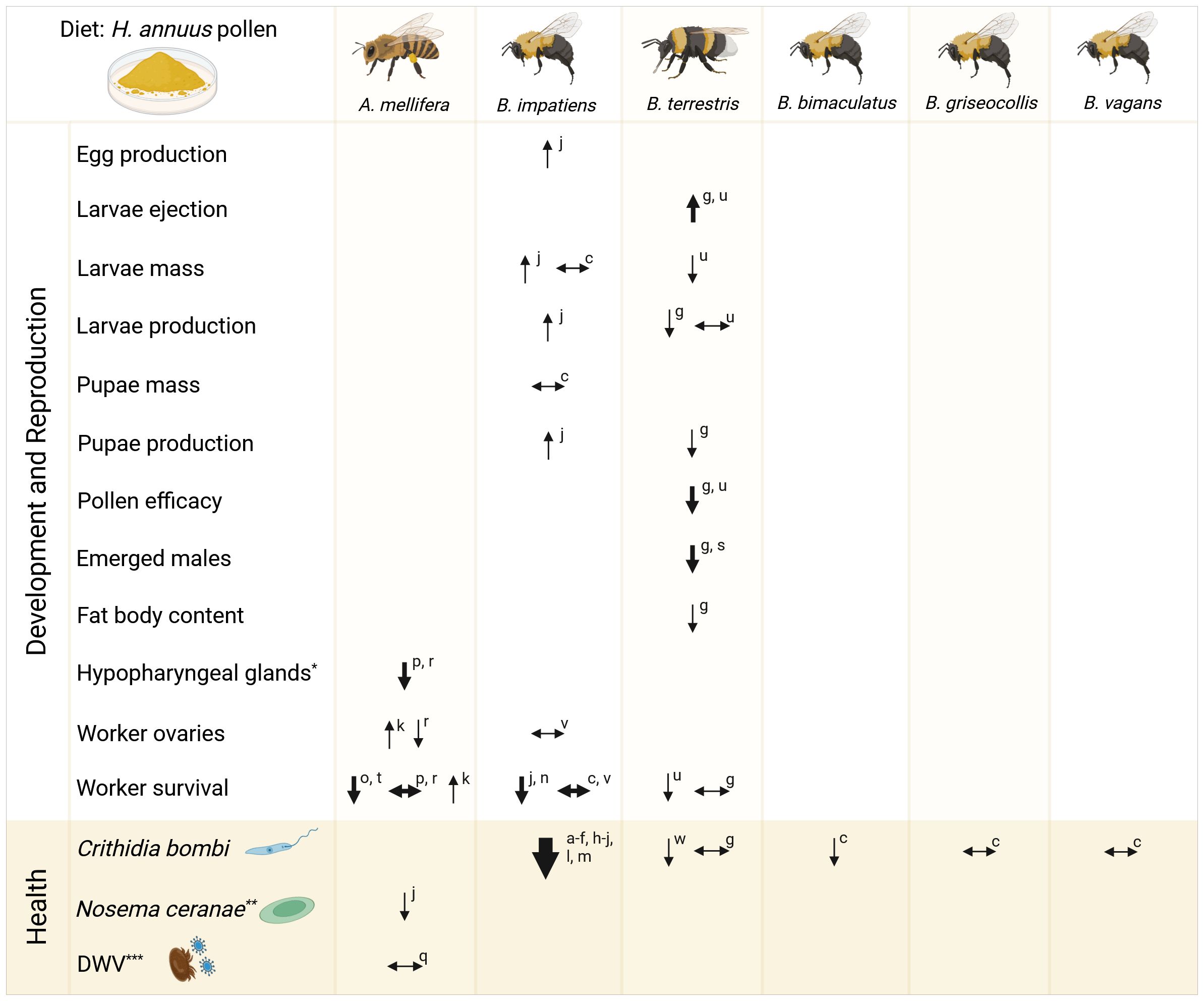
Figure 2. Sunflower pollen diet on bee development and health. Reported literature results on the impact of Helianthus annuus pollen diet on survival, development, physiological, and health parameters in experimental queen-less cage assays. Arrow thickness indicates the number of studies for the corresponding parameter (thin: 1 study; medium: 2 studies; thick: > 10 studies). *Measured as protein content or volume; **recently renamed Vairimorpha ceranae; ***DWV, Deformed wing virus transmitted by Varroa destructor. Created in BioRender Reference letters: (a) Adler et al., 2020; (b) Figueroa et al., 2023; (c) Fowler et al., 2022a; (d) Fowler et al., 2023; (e) Fowler et al., 2022b; (f) Fowler et al., 2020; (g) Gekière et al., 2022; (h) Giacomini et al., 2023; (i) Giacomini et al., 2021; (j) Giacomini et al., 2018; (k) Human et al., 2007; (l) LoCascio et al., 2019a; (m) LoCascio et al., 2019b; (n) McAulay and Forrest, 2019; (o) Nicolson et al., 2018; (p) Omar et al., 2017; (q) Palmer-Young et al., 2023; (r) Pernal and Currie, 2000; (s) Regali and Rasmont, 1995; (t) Schmidt et al., 1995; (u) Tasei and Aupinel, 2008; (v) Treanore et al., 2019; (w) Vanderplanck et al., 2023.
Overall the variable results, partial investigations for A. mellifera and B. impatiens, and lack of research regarding wild bee species, the nutritional impact of sunflower pollen is still not fully understood. In general, there is limited research on the potential impact of specific nutritional components on reproduction, development, cognition, and even foraging preferences. It was found that a deficiency in omega-3 fatty acid resulted in smaller hypopharyngeal glands (Arien et al., 2015), omega-3 and oleic acid deficiencies reduced learning abilities (Arien et al., 2015; Muth et al., 2018), and high ratios of omega-6:3 was detrimental to cognitive performance (Arien et al., 2018). Pollen sterol content was also found to be a key nutrient for bumble bee larvae growth, likely due to their role in hormone synthesis and cell membrane function (Moerman et al., 2017). Regarding pollen mineral nutrients, Filipiak and Filipiak (2020) found that K deficiency reduced survival and impaired cocoon development for the mason bee Osmia bicornis.
Assessing colony health encompasses more than just reproductive and developmental metrics, but also evaluating their resistance and susceptibility to pathogens and parasites. Numerous studies have challenged the perception of sunflower pollen as “low-quality”, as it consistently shows a positive health impact by reducing the gut parasite Crithidia bombi in Bombus species, particularly B. impatiens (Figure 2). Sunflower pollen’s impact on C. bombi has been discovered repetitively for B. impatiens; nevertheless, variable results were found investigating sunflower pollen on C. bombi infection intensity in B. terrestris (Gekière et al., 2022; Vanderplanck et al., 2023) and only one study found variable results between various wild Bombus species (Fowler et al., 2022a). Not much is known regarding the impact on other pathogens or parasites. Nosema ceranae (recently renamed Vairimorpha ceranae) cell counts decreased in A. mellifera fed sunflower pollen, but it increased mortality as if they underwent pollen starvation (Giacomini et al., 2018). Furthermore, one study reported no impact on infection intensity of deformed wing virus (DWV) in sunflower fed honey bees (Palmer-Young et al., 2023).
Many studies have tested whether the effect from sunflower pollen was due to specific pollen nutrient components, such as individual fatty acids or secondary metabolites rutin or tricoumaroyl spermidine (Adler et al., 2020) or other unique phenolamides (Gekière et al., 2022). Furthermore, Yost et al. (2023) had the hypothesis that bumble bees fed sunflower pollen might have an influenced gut microbiome impacting pathogen resistance. They fed B. impatiens (inoculated with C. bombi) a solution of guts dissected from bumble bees fed either sunflower or buckwheat pollen while on a control diet of wildflower pollen. However, they found no differences in C. bombi cell counts between the recipient bees fed either gut solution. Interestingly, Giacomini et al. (2023) found that sunflower pollen consumption upregulated immune transcripts linked to the maintenance and repair of gut epithelial cells, theorizing pollen’s role in disrupting infection from C. bombi by potentially removing or preventing the attachment of flagellated pathogens. Figueroa et al.’s (2023) clever study design found that sunflower exines alone reduced infection intensity (along with 4 of 7 additional Asteraceae species compared to non-Asteraceae pollen); thus, supporting the role of spiny morphology for infection suppression (Figure 3). Yet, a reduction in C. bombi infection intensity was not present in all spiny pollen treatments (Figueroa et al., 2023). If the morphological features play a large role in infection intensity, further research needs to be done regarding more pollen external features, for example: spine density, spine length, and pollen grain size.
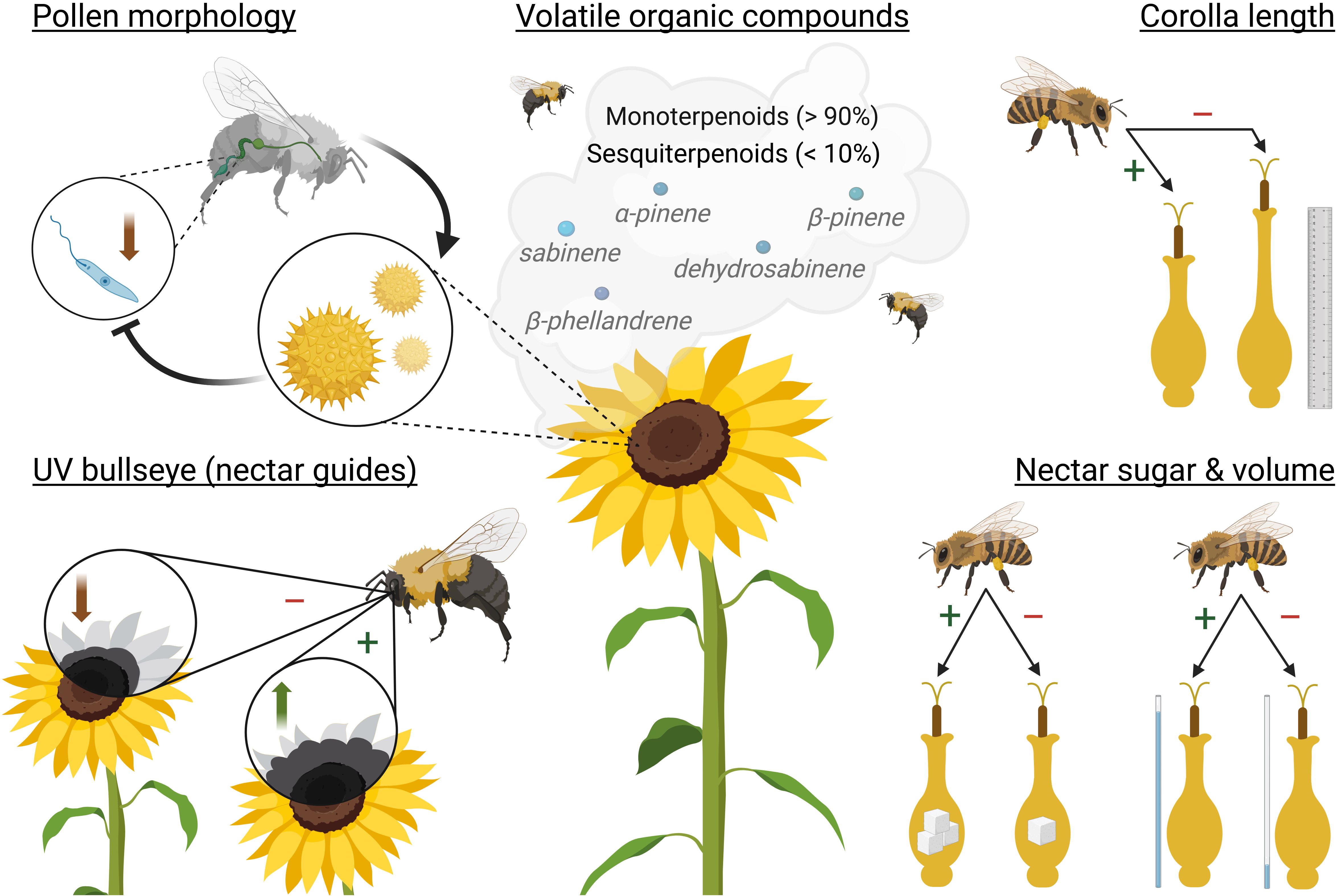
Figure 3. Key sunflower pollination syndromes. Clockwise from the top left with respective section in review: sunflower pollen consumption on Crithidia bombi infection intensity in Bombus spp. (section 3.1); dominant sunflower volatile organic compounds (section 4.2); corolla length on pollinator attraction (section 4.1); sunflower nectar sugar content and volume on pollinator attraction (section 4.4); and increased UV bullseye size (nectar guides) on pollinator attraction (section 4.1). Plus indicates increased pollinator attraction and minus indicates less attraction. Created in BioRender.
With the focus on the morphological aspect of sunflower pollen, Knoerr et al. (2024) followed up this finding on whether the spiny structure could result in intestinal damage. They found that sunflower exines added to diets resulted in no differences in B. impatiens mortality or intestinal damage, however those fed spines reduced larval and microcolony weights. As suggested by Knoerr et al. (2024), these negative findings could be a consequence of larvae consuming higher amounts of empty exines, rather than full pollen grains. Likewise, Vanderplanck et al. (2020) found no intestinal damage in the digestive tract of B. terrestris fed spiny whole Taraxacum pollen, but they also reported higher larval ejection compared to non-spiny treatments. Ultimately, the studies addressing C. bombi are limited mostly to B. impatiens (Figure 2); therefore, a knowledge gap persists for species specific interactions. This is particularly relevant given that both sunflower and B. impatiens are native to North America. Thus far, developmental parameters appear to be less detrimental, and the medicinal benefits of sunflower pollen more consistent for B. impatiens compared to B. terrestris. Further research is needed to explore these interactions in the context of sunflowers impact on native vs. non-native pollinators.
Major limitations of the studies summarized in Figure 2 include their confinement to laboratory environments, the use of queen-less bumble bee colonies, variations in pollen collection methods used for feeding (hand- vs. honey bee-collected pollen), and comparisons between sunflower pollen and other pollen sources that differ in seasonal availability (often constrained by commercial availability). This further complicates efforts to obtain a clear picture on the impacts of sunflower pollen consumption. Only a few studies have investigated field realistic conditions. Charrière et al. (2010) found no difference in brood production between their honey bee colonies placed next to sunflower fields and control colonies placed 3 km away. Queen production of B. impatiens increased 30% with every order of magnitude increase in sunflower area (Malfi et al., 2023). Considering sunflowers apparent medicinal properties, an increase in sunflower area was also associated with reduced C. bombi prevalence, however no effect on Apicystis bombi or the black queen cell virus (BQCV) was found (Malfi et al., 2023). It was also found that every 10-fold increase in sunflower area decreased C. bombi infection intensity 23.2% (Giacomini et al., 2018) and a two-fold increase in sunflower area resulted in a 28% decrease in Varroa mite infestation for A. mellifera, without effects on Nosema ceranae (Palmer-Young et al., 2023). These studies are difficult to associate these effects solely to sunflower resource availability. Either these studies did not consider how much sunflower pollen was collected or they found relatively low quantities. Malfi et al. (2023) recorded that only 8.5% of bumble bees carried sunflower pollen compared to 46% carrying other Asteraceae pollen, but this was proportional to the Helianthus annuus cover compared to other flowering sources. Charrière et al. (2010) found that their treatment colonies placed at the edge of sunflower fields collected only 2.2–3% sunflower pollen in 2003, and 11–38% in 2004, depending on the colony and location, as they favored other sources despite sunflower abundance. This highlights a key point that should not be overlooked, such that lab experiments only provide a snapshot on how colony health may be impacted. They do not show colonies ability to compensate for nutritional imbalances or the realistic conditions that pollinators forage on a diversity of pollen sources. As previously mentioned, it is known that mono-diets are not considered well-balanced diets for social bees (Alaux et al., 2010; Di Pasquale et al., 2013, 2016; Leponiemi et al., 2023), presumably also not for solitary bees. Given that bees forage from a variety of pollen sources, it’s unclear if a single source, like sunflower pollen, can be classified as health detrimental or beneficial.
3.2 Nectar
When assessing the nutritional impact of floral resources on bees, it is crucial to consider more than just pollen. Bees’ diets also include nectar, and some species consume bee bread and honey, all of which contribute to their overall nutrition. Except the basic nutritional summary, no studies have investigated sunflower nectar consumption on pollinator nutrition. Nectar nutritional studies often focus on the predominant secondary metabolites found in nectar from various plant species, using commercially sourced metabolites for experimentation. The consumption of anabasine, catalpol, nicotine, and thymol reduced the cell count of C. bombi infection in B. impatiens (Richardson et al., 2015); as well as a reduced fecal intensity of C. bombi from an alkaloid, gelsemine, found in nectar (Manson et al., 2010). Moreover, abscisic acid enhanced immune responses in Apis mellifera (Negri et al., 2015). On that note, it cannot be ignored that metabolites in sunflower nectar could also contribute to bee physiology and health, though we first need to understand the composition of non-sugar metabolites offered.
3.3 Bee bread and honey
Stored pollen and nectar in the hive provide essential support to workers, offering resources during times of need and aiding honey bee workers which are conducting their roles inside the hive. Of course, the question arises on whether these stored resources might offer an advantage in their nutritional nature compared to raw nectar and pollen. As previously mentioned, bee bread is debated on whether it differs in nutritional quality compared to pollen. Only one study investigated sunflower pollen vs. bee bread and found no impacts on A. melliferas’ survival or extraction efficiency (digestion efficiency of individual pollen grains), meaning that pollen was not digested better in either fresh or stored form (Nicolson et al., 2018). Despite some studies supporting the notion that pollen and bee bread do differ (see section 2.3), there is no current support indicating this difference has realistic impacts for bee fitness.
Similarly, this knowledge gap holds true for sunflower honey. Honey offers unique properties in terms of its antimicrobial nature. The antimicrobial properties come from its’ high sugar content which results in an osmotic pressure dehydrating bacterial cells (Molan, 1992); an accumulation of hydrogen peroxide from the glucose oxidase system (White et al., 1963); and the presence of phenolic acids and flavonoids (Čižmárik and Matel, 1970; Ferreres et al., 1994). An expansive nutritional profile for sunflower honey exists, presumably since honey is consumed by humans. Our knowledge on how honey’s high antimicrobial and antioxidant properties can enhance honey bee health is limited. Studies have shown that sunflower honey displays mild antimicrobial activity against Staphylococcus aureus (Emin Duru et al., 2023), Escherichia coli, Candida parapsilopsis, C. albicans, Listeria monocitogenes, and Bacillus cereus (Pătruică et al., 2022). Thus, it is hypothesized that honey could have medicinal properties against bee relevant bacteria or pathogens. Gherman et al. (2014) observed in a choice assay using honey bees, that honey bees infected with Nosema ceranae increasing preferred sunflower honey with increasing infection intensity compared to honeydew honey. Furthermore, they also showed that sunflower honey fed honey bees had significantly lower N. ceranae spore load compared to honeydew honey. In a bacterial growth inhibitory study, sunflower honey inhibited American foulbrood bacterial strains more effectively than black locust honey and completely inhibited Paenibacillus alvei growth; yet, black locust honey was more effective on reducing European foulbrood related bacteria (Erler et al., 2014). Based on this study, the antimicrobial and antibiotic properties of honey seems to be honey and bacterial strain specific. It could be worth exploring how the availability of various floral resources and subsequent honey types present in a honey bee hive might drive the pathogen susceptibility of a colony, or whether they can selectively consume honey of a certain nature depending on need. Understanding this interaction could provide useful colony health indicators for scientists and beekeepers.
4 Drivers of sunflower-pollinator interaction
4.1 Floral morphology and pigments
This review has primarily focused on sunflower pollination syndromes that provide nutrition to pollinators, primarily bees. However, pollinator preferences and foraging decisions are influenced by the association of the reward, potentially the nutritional quality, and to certain floral traits (i.e., flower size, color, scent, etc.) (Chittka and Raine, 2006; Frachon et al., 2021; Knauer and Schiestl, 2015). Sunflower ligules, the modified petals on the outermost part of the inflorescence, often appear yellow or orange due to the presence of secondary metabolites, primarily carotenoids (responsible for yellow to orange pigmentation) and flavonoids. Flavonoids are a diverse group of phenolic secondary metabolites encompassing anthocyanins (orange, blue, purple, or even black hues), and other groups, such as flavonols and flavones, which provide pale yellow hues or are mostly colorless to the human eye (reviewed by Iwashina, 2015). The regulation of ligule color in sunflower has been recently proposed to be governed by the HaMYBA-HabHLH1 and HaMYBF complex which regulates anthocyanin and flavonol accumulation, respectively (Jiang et al., 2024). Likewise, Ma et al. (2024) reported HanMYB1 plays a role in regulation of anthocyanin accumulation. They found that chrysanthemin and epigallocatechin are major anthocyanins in red sunflowers and rutin and kaempferol are major flavones and flavonols in yellow sunflowers. See Galiseo et al.’s (2024) review on the identified carotenoid compounds found in H. annuus.
Flower pigments that are most interesting to pollinator attraction are the ultraviolet (UV)-absorbing pigments that are not visible to humans, but are responsible for UV patterns on flowers called nectar guides (Leonard and Papaj, 2011; Penny, 1983; Todesco et al., 2022). UV-absorbing pigments accumulate in the ligules base and the outermost edge reflects UV radiation resulting in a bullseye pattern visible to foraging bees (Moyers et al. 2017; Todesco et al. 2022). These UV-absorbing pigments consist primarily from flavonols and flavones. In particular for sunflower, biosynthesis of flavonol glycoside pigments is governed by the transcription factor, HaMYB111 (Todesco et al., 2022). In an elaborate study to test bullseye pattern size and pollinator attraction, Todesco et al. (2022) found that within 1484 individuals from 106 H. annuus populations, individuals with intermediate UV patterns had the highest pollinator visitation rates compared to small or large patterns (Figure 3). Similarly, Rudbeckia flowers with smaller bullseye patterns (reduced nectar guides) had significantly less pollinator visitation (Horth et al., 2014).
Accumulation of these flavonoids have been shown to vary tremendously in the Helianthus genus and between H. annuus individuals (Scogin, 1978; Todesco et al., 2022). Thus, questions arise regarding their ecological significance. Todesco et al. (2022) found that the bullseye patterns are correlated to geoclimate factors, which is not surprising as flavonols are involved in mitigating abiotic stressors (reviewed in Shomali et al., 2022). Larger UV bullseyes were present in colder environments; however the variability in size was predominantly correlated to lower humidity climates. This suggests flavonoids’ dual role for pollinator attraction and water conservation in sunflowers.
Floral color and nectar guides facilitate visual identification, but upon floral visitation there are additional features that can enable easier access to the floral reward. The sunflower inflorescence consists of many individual flowers, disc florets, each formed by a corolla composed of 5 fused petals. Inside the corolla are the male and female reproductive parts and the nectary is at the base (Sammataro et al., 1985). Many studies have found that the corolla length of sunflower has an impact on pollinator visitation frequency (Figure 3). Shorter corollas, thus easier nectar access, increased both wild bee and honey bee visits (du Toit and Coetzer, 1991; Ferguson et al., 2024; Mallinger and Prasifka, 2017b; Portlas et al., 2018). Ferguson et al. (2021) also found that bee community differed based on corolla length relative to tongue length. Most bees preferred shorter corollas, whereas Bombus spp. showed a preference for sunflower lines with larger florets. Significant foraging preferences relative to corolla length was also reported dependent on the year or sowing period (Ferguson et al., 2021, 2024).
Ultimately, sunflower-pollinator interactions have been shown to be impacted by floral UV patterns and corolla length, but research is lacking in regards to other floral traits. For example, sunflowers are known to have a high density of glandular trichomes present on both leaves and florets, and have been shown to produce allelochemicals which may be important for plant defense again pathogens and herbivores (Brentan Silva et al., 2017). Sesquiterpene lactones are present in glandular trichomes of sunflower and have been shown to defend against insect pests (Chou and Mullin, 1993; Mullin et al., 1991; Prasifka et al., 2015). Little research has been done on trichomes impact on pollinators. It has been theorized that oleiferous trichomes in Bulbophyllum saltatorium might play a role in attracting pollinators (Stpiczyńska et al., 2018). This is an important future topic to address through the pollinator perspective, as we cannot exclude the influence of sunflower trichomes on their interactions.
4.2 Olfactory attraction
Pollinators use both visual and olfactory cues to navigate the complicated environment (Burger et al., 2010; Leonard et al., 2012). Volatile organic compounds (VOCs) include a vast diversity of terpenes, among those are monoterpenoids and sesquiterpenoids which play a major role in floral scent (Pichersky and Raguso, 2018). We are only beginning to unravel their complexity and interactions with the biotic environment, including their roles in attracting or repelling pollinators and herbivores (Raguso, 2008; Pichersky and Raguso, 2018; Slavković and Bendahmane, 2023; Wright and Schiestl, 2009). Research regarding sunflower VOCs began in the late 1980s to early 1990s with a few studies identifying potential pollinator discriminatory compounds (Pham-Delègue et al., 1986, 1990; Thiery et al., 1990). A long gap succeeded this research and only recently interest has surged, likely driven by growing sunflower breeding efforts, leading to extensive identification of between 100 to 500 VOCs in wild and cultivated Helianthus species (Anandappa et al., 2023; Bahmani et al., 2022, 2023; Jocković et al., 2024). Few studies have also addressed sunflower VOCs to understand plant-pest interactions for thrips (Qu et al., 2024) and yellow peach moth (Zhou et al., 2023).
Based on recent studies, the volatile profile of petals or disc florets of wild and domesticated H. annuus were dominated by monoterpenoids (> 90%), followed by sesquiterpenoids (< 10%), and even fewer non-terpene compounds (Anandappa et al., 2023; Bahmani et al., 2022, 2023). Of the monoterpenoids, α-pinene always had the highest relative abundance (Anandappa et al., 2023; Bahmani et al., 2022, 2023; Qu et al., 2024; Zhou et al., 2023). This consistency is surprising considering studies have investigated different floral tissues (petals vs. disc florets), used different sample preparation (fresh vs. frozen), and analysis methods. Thus, monoterpenoid-sesquiterpenoid balance and the high abundance of α-pinene appears characteristic for H. annuus (Figure 3). Though there is some variance in the less abundant VOCs. Cultivated H. annuus contained 6–20% sabinene, which was only present in 1% or less of wild H. annuus (Anandappa et al., 2023). They also found higher proportions of VOCs in cultivated H. annuus which are considered pleasant to human smell, such as D-limonene, eucalyptol, and βphellandrene (Anandappa et al., 2023). The second most prevalent monoterpenoid was either sabinene (Anandappa et al., 2023; Bahmani et al., 2023), dehydrosabinene (Bahmani et al., 2022), β-pinene (Qu et al., 2024), or β-phellandrene (Zhou et al., 2023) (Figure 3). The remaining major VOCs reported across H. annuus studies were D-limonene, β-pinene, and γ-terpinene (Bahmani et al., 2022); D-limonene, (+)-calarene, and germacrene D (Qu et al., 2024); β-pinene, D-limonene, α-terpinene, γ-terpinene, terpinene-4-ol, o-cymene, and bornyl acetate (Bahmani et al., 2023); and camphene, β-pinene, 3-carene, D-limonene, eucalyptol, γ-terpinene, and valencene (Zhou et al., 2023).
The total relative abundance of VOCs between wild and cultivated H. annuus is up for debate. Bahmani et al. (2022, 2023) found that total VOC abundance was significantly lower in cultivated sunflowers, and sesquiterpenoids were reduced by 6–8 times. However, this was not supported by Anandappa et al. (2023). The potential impact of reduced volatile abundance on pollinator attraction and decreased sesquiterpenoids on herbivore defense in cultivated sunflowers warrants further investigation (see Prasifka et al., 2015). Overall, H. annuus appears to have relatively consistent compound diversity and monoterpenoid-sesquiterpenoid balance. This is not the case when looking at other species in the Helianthus genus. Species are found to vary in their floral monoterpenoid-sesquiterpenoid composition, compound abundance, and major volatiles (Bahmani et al., 2022; Jocković et al., 2024). Bahmani et al. (2022) also found that among the wild Helianthus species, higher monoterpenoids were found in arid climates for annuals and erect perennials, and sesquiterpenoids were higher in mesic habitats for erect perennials. Interestingly, Todesco et al. (2022) found that arid climates had larger nectar guides to attract pollinators, proposing this was driven by geoclimate conditions. Nonetheless, it is an interesting connection, as arid climates may have both increased monoterpenoids and larger nectar guides.
Pollen and nectar VOCs are less studied but are important, likely contributing to the floral VOC profile and both serve as a key nutritional resources for pollinators. No studies have addressed nectar specific VOCs, and only a few have for pollen. Bertoli et al. (2011) found a greater abundance of sesquiterpenoids compared to monoterpenoids. The major VOCs consisted of the sesquiterpenoids β-elemene, β-gurjunene, β-chamigrene, germacrene D, and trans-γ-cadinene, as well as the monoterpenoids α-pinene oxide, trans-verbenol, pinocarvone, myrtenol, verbenone, and isobornyl acetate. In contrast, Qu et al. (2024) found that the major compounds mirrored their VOCs reported in disc florets, with the exception of α-terpinene and γ-selinene found in pollen. Leaf VOCs are another dimension to consider for plant-insect interactions. As our focus is on floral structures, we exclude them here; see Galisteo et al. (2024) for a review on sunflower terpenoid diversity.
Identifying VOCs driving plant-pollinator interactions is challenging, as production and emission are complex, and VOCs often exist in a bouquet rather than single compounds. We are just starting to understand which specific compound or mix of VOCs correspond to pollinator attraction or deterrence. Studies from Pham-Delègue et al. (1986, 1990) and Thiery et al. (1990) identified a fraction of compounds isolated from VOCs of sunflower that either elicited behavior or antennae responses by honey bees. When comparing their proposed “pollinator recognizable” compounds to recently reported major floral and pollen VOCs in sunflower, only bornyl acetate, eucalyptol, and germacrene D were found in floral VOCs, while verbenone, β-elemene, myrtenol, and germacrene D were found in pollen. In other studies, Blight et al. (1997) found eight VOCs from Brassica napus flowers were recognized by honey bees. Those that also occurred as major VOCs in sunflower were α-pinene, α-terpinene, and 3-carene. Farina et al. (2020) used the mechanism of associative bee learning with floral rewards to elicit a recruitment behavior of honey bees towards sunflower fields by feeding honey bee colonies with sugar syrup scented with sabinene, beta-pinene and limonene (see also Estravis-Barcala et al., 2021; Farina et al., 2023), all three identified as major sunflower VOCs. Nonetheless, the highest abundance does not necessarily indicate the most relevant, as volatiles with low abundance or their combinations may also be important, as either attractive or deterrents. In particular, honey bees are able to detect subtle differences in the ratio of two odors, and identify individual compounds in complex floral scents (Wright and Schiestl, 2009). Although not related to sunflowers, recent studies have identified some VOCs as either innate attractants or repellents to bees. For example, ß-trans-bergamotene was found to be an innate “dishonest” attractant for bumble bees, as they preferred it in higher amounts even from non-rewarding flowers (Haber et al., 2019). Conversely, repellent VOCs discovered were α- and β-selinenes found in carrot genotypes (Quarrell et al., 2023); E-2-hexanal, Z-3-hexenol, and Z-3-hexenyl acetate found in strawberry cultivars (Ceuppens et al., 2015; Klatt et al., 2013); and dioxolanes, piperidines and organosulfur compounds found in onion genotypes (Soto et al., 2015). The evidence we have for VOCs and pollinators is quite limited to laboratory settings which do not directly translate to real environment interactions. There is a lot more we can learn from understanding the chemical world, including those pertaining to sunflower-pollinator interactions.
4.3 Pollen
We do not know much about what drives specific choices of pollen collection by pollinators, let alone understanding how the nutritional components of particular species of pollen might influence foraging decisions. For sunflowers, studies have made more observational links to foraging preferences based on sunflower pollen production. Such that, honey bees have shown to exhibit preference to male sterile sunflowers (lacking pollen) (Estravis Barcala et al., 2019; Mallinger and Prasifka, 2017b; Pham-Delègue et al., 1990; Skinner, 1987; Tepedino and Parker, 1982), whereas Melissodes spp. and other wild bees were most abundant on male fertile sunflowers (Estravis Barcala et al., 2019; Mallinger and Prasifka, 2017b; Tepedino and Parker, 1982). Furthermore, few studies have addressed foraging preferences linked to pollen chemistry. Ferguson et al. (2024) investigated the fatty acid content of pollen across different sunflower lines to examine its association with pollinator visitation. However, they found high variability between years, suggesting that the nutritional composition of sunflower pollen may be more variable than previously expected. Although not specific to sunflower, foraging preferences were linked to protein:lipid (P:L) ratios, which differed among bee species. B. impatiens favored higher P:L ratios in the range from 4:1 to 10:1 (Vaudo et al., 2016, 2020), while honey bees preferred 1:1 and 2:1 ratios (Vaudo et al., 2020). The nutritional requirements of pollinators may influence their foraging preferences. However, linking specific nutritional compounds to these choices is highly complex, as various plant attraction mechanisms, such as nectar, flower color, morphology, and olfactory compounds, also play a role.
It is known that pollen is a more specialized resource to collect than nectar. Pollen’s primary function is for plant reproduction; thus there is inherent conflict to the double function of pollen, between plant reproduction and food resources for the Anthophila clade (Michez et al., 2012; Murray et al., 2018). It is therefore logical that pollen contains defenses to deter herbivores and over consumers (pollen defense on bee ecology reviewed in Rivest and Forrest, 2020). Major pollen defenses are hypothesized to be chemical; however, very few studies have linked plant secondary metabolites, mostly alkaloids, to negative pollinator effects (see Rivest and Forrest, 2020; Rivest et al., 2024). Furthermore, Asteraceae species like sunflower, have spined pollen which may act as a morphological defense against consumption and may influence the visiting pollinator community (see Rivest et al., 2024), but this topic needs more research. How the bioactive properties or morphological aspects of pollen can govern foraging preferences is not well understood (see Tourbez et al., 2023; Vanderplanck et al., 2019). Especially in regards to how these factors may drive foraging preferences based on nutritional needs, pollinator specific adaptions, or potential medicinal benefits (as discussed in section 3.1).
4.4 Nectar
Focusing on the interactions between nectar traits and pollinators, nectar volume and sugar content are key drivers influencing visitation in sunflowers (Figure 3). Wild bees and honey bees increased visitation with increased total sugar content (Estravis Barcala et al., 2019; Mallinger and Prasifka, 2017b), but not by the specific sugar composition of nectar (Mallinger and Prasifka, 2017b, see also Soto et al., 2021 with onion). In contrast, Pham-Delègue et al. (1990), assumed sucrose to be the main driver of visitation in honey bees, but this was concluded based on testing only two parental lines of two hybrids. Pham-Delègue et al. (1990) did not measure additional floral characteristics or nectar volume, thus visitations cannot be only associated to sucrose content. Nectar volume and total sugar content are influenced by genotype, environment, and their interactions (Chabert et al., 2020; Terzić et al., 2017). Many studies have found significant differences between foraging preferences among different sunflower genotypes (Bergonzoli et al., 2022; Cerrutti and Pontet, 2016; Chambó et al., 2011; Fell, 1986; Sapir, 2009; Stejskalová et al., 2018). With numerous studies indicating variations in cultivar attractiveness, genetic mapping of traits responsible for nectar production and chemistry is emerging as a key focus in sunflower breeding efforts aimed at enhancing plant appeal to pollinators. Two homologous genes, ARF8 and DAD1, governing nectar production in Arabidopsis, have been reported as candidate genes governing sunflower nectar volume (Barstow et al., 2022). Additionally, HaCWINV2 gene, putatively involved in sucrose metabolism, had a strong linear association between gene expression and glucose and fructose content (Aquino et al., 2021). Despite the significant influence of genetic × environmental interactions, sunflower breeding efforts have identified strong genotype effects (Chabert et al., 2020; Terzić et al., 2017). Consequently, the breeding of sunflowers with both stable and specific nectar volume and sugar quantity and quality remains appealing for optimizing floral resources for bees.
Nectar is no longer seen as a simple sugar reward for pollinators, but in fact has a complicated chemistry. Recent literature focused on the presence of amino acids, nectarins, and both attractive and toxic secondary metabolites (reviewed in the following studies, Adler, 2000; Barberis et al., 2023; Heil, 2011; Nepi, 2017; Roy et al., 2017). In general, though not specifically for sunflowers, numerous nectar studies investigated the diverse roles of specific chemical compounds in herbivore defense, antimicrobial activity, health benefits, and their physiological effects on pollinator cognition and foraging preferences (Barberis et al., 2023). Honey bees showed a preference for phenylalanine enriched solutions (Hendriksma et al., 2014) and varying concentrations of proline (Carter et al., 2006). Further intriguing findings revealed the presence of components of the honey bee queen mandibular pheromone in the nectar of buckwheat and Mexican sunflower, Tithonia diversifolia (Liu et al., 2015). They found that in an artificial nectar solution, Apis cerana exhibited a preference for these compounds. Nicotine and caffeine consumption in nectar have been shown to enhance foraging memory and learning (Baracchi et al., 2017; Wright et al., 2013). Specifically for caffeine, its concentration plays a crucial role. Wright et al. (2013) demonstrated that caffeine enhances bees’ memory for floral rewards, whereas at higher concentrations, such as those found in Citrus × meyeri, it elicited an aversive response in bees (Muth et al., 2022). The complexity continues as Muth et al. (2022) further showed that two insect neurotransmitters regulating foraging behaviors found in nectar (octopamine and tyramine), when present with caffeine, eliminated bees distaste to caffeine rich floral nectar.
Specific deterrent properties of nectar have been associated to presence of potassium in some onion and avocado genotypes (Afik et al., 2006a, b; Hernández et al., 2019; Soto et al., 2013) and the terpenoid triptolide, which reduced olfactory learning and memory (Zhang et al., 2018). These deterrents have the dual effect of (i) reducing the palatability of nectar and (ii) altering the associated learning of floral cues with the presence of such compounds. As has been shown with caffeine, we know very little about nectar modulators and whether deterrent properties in nectar are counteracted by other compounds influencing pollinator visitation.
Nectar chemistry is also complicated to investigate due to other interesting and understudied factors, such as the presence and community of floral microbes. To the best of our knowledge no literature has investigated the nectar microbiome of sunflower nectar. Various microbe nectar specialists including yeasts (i.e., Metschnikowia spp.) and bacteria (i.e., Acinetobacter spp. and Rosenbergiella spp.) have been identified and may play roles on altering floral attraction and foraging preferences, or impacting the nutritional availability of nectar to pollinators (reviewed in Martin et al., 2022; Quevedo-Caraballo et al., 2025; Vannette, 2020). In particular, yeasts, Merremia aegyptia and Cordia sebestena, significantly affected the concentration of individual nectar sugars and their proportions (Canto and Herrera, 2012). Metschnikowia reukaufii was found to be a deterrent to honey bees and decreased the concentration of amino acids and increased the amount of volatiles emitted in nectar (Rering et al., 2021). Nectar bacteria, Acinetobacter, can stimulate pollen germination and bursting (Christensen et al., 2021), potentially influencing the nutritional availability to pollinators. How the precise interactions guiding synergistic or antagonistic relationships between plant and microbe and further extended to pollinators needs more study.
5 Conclusion
Sunflower pollen and nectar show significant variability in their macro- and micronutrient chemistry. Nectar chemistry is a complicated blend of various compounds, but our knowledge of sunflower nectar is limited to sugar compounds with much still left to be studied. Additionally, inconsistency was reported for developmental parameters measured after bee consumption of sunflower pollen. Both aspects of variability in nutrition and subsequent bee development makes it challenging to determine the nutritional potential sunflowers may provide neighboring pollinator communities. Despite these variable and undefined nutritional aspects, it has been continuously reported that sunflower pollen has a medicinal effect on reducing C. bombi in Bombus spp. This encourages an additional factor to consider when defining the quality of sunflower floral resources. The extent of sunflower-pollinator interaction goes beyond nutrition, as we know that certain sunflower cultivars seem to have increased pollinator attractiveness, presumably from key pollination syndromes (Figure 3). Ultimately, with the rise of breeding efforts and sunflower cultivation as a bee-friendly crop, it is important to continue understanding the balance between sunflower as a nutritional source versus its’ attractive pollination syndromes.
Author contributions
SH: Conceptualization, Data curation, Investigation, Visualization, Writing – original draft, Writing – review & editing. KC: Funding acquisition, Investigation, Writing – review & editing. OC: Investigation, Writing – review & editing. SC: Investigation, Writing – review & editing. SE: Conceptualization, Funding acquisition, Investigation, Supervision, Writing – review & editing.
Funding
The author(s) declare that financial support was received for the research and/or publication of this article. Project HelEx is funded by the European Union’s Horizon Europe Research and Innovation Actions program under grant agreement N°101081974.
Acknowledgments
We would like to sincerely thank Manuel Strohmaier from Joanneum Research in Graz, Austria for generously contributing his expertise, time, and valuable assistance in creating the sunflower map (Figure 1) featured in this manuscript. We would also like to thank both reviewers who offered valuable insights to improve the strength of this manuscript.
Conflict of interest
The authors declare that the research was conducted in the absence of any commercial or financial relationships that could be construed as a potential conflict of interest.
The author(s) declared that they were an editorial board member of Frontiers, at the time of submission. This had no impact on the peer review process and the final decision.
Generative AI statement
The author(s) declare that no Generative AI was used in the creation of this manuscript.
Publisher’s note
All claims expressed in this article are solely those of the authors and do not necessarily represent those of their affiliated organizations, or those of the publisher, the editors and the reviewers. Any product that may be evaluated in this article, or claim that may be made by its manufacturer, is not guaranteed or endorsed by the publisher.
References
Abrol, D. P., Kapil, R. P. (1991). Foraging strategies of honeybees and solitary bees as determined by nectar sugar components. Proc. Indian Natn. Sci. Acad. B57, 127–132.
Adler, L. S. (2000). The ecological significance of toxic nectar. Oikos. 91 (3), 409–420. doi: 10.1034/j.1600-0706.2000.910301.x
Adler, L. S., Fowler, A. E., Malfi, R. L., Anderson, P. R., Coppinger, L. M., Deneen, P. M., et al. (2020). Assessing chemical mechanisms underlying the effects of sunflower pollen on a gut pathogen in bumble bees. J. Chem. Ecol. 46, 649–658. doi: 10.1007/s10886-020-01168-4
Afik, O., Dag, A., Kerem, Z., Shafir, S. (2006b). Analyses of avocado (Persea americana) nectar properties and their perception by honey bees (Apis mellifera). J. Chem. Ecol. 32, 1949–1963. doi: 10.1007/s10886-006-9120-1
Afik, O., Dag, A., Shafir, S. (2006a). The effect of avocado (Persea americana) nectar composition on its attractiveness to honey bees (Apis mellifera). Apidologie 37, 317–325. doi: 10.1051/apido:2005064
Alaux, C., Ducloz, F., Crauser, D., Le Conte, Y. (2010). Diet effects on honeybee immunocompetence. Biol. Letters. 6, 562–565. doi: 10.1098/rsbl.2009.0986
Anandappa, J. A., Stanford, H. E., Marek, L. F., Goolsby, E. W., Mason, C. M. (2023). Bioprospecting for improved floral fragrance in wild sunflowers. Helia 46, 143–167. doi: 10.1515/helia-2023-0008
Aquino, J. D. C., Juan, X. P., Gaban, P. B. V. (2021). Sunflower (Helianthus annuus L.) floral nectar characterization and gene expression analysis of sucrose hydrolyzing gene HaCWINV2. Philippine J. Sci. 150, 1079–1087. doi: 10.56899/150.05.20
Arien, Y., Dag, A., Shafir, S. (2018). Omega-6:3 ratio more than absolute lipid level in diet affects associative learning in honey bees. Front. Psychol. 9. doi: 10.3389/fpsyg.2018.01001
Arien, Y., Dag, A., Zarchin, S., Masci, T., Shafir, S. (2015). Omega-3 deficiency impairs honey bee learning. Proc. Natl. Acad. Sci. U.S.A. 112, 15761–15766. doi: 10.1073/pnas.1517375112
Armbruster, W. S. (2012). “Evolution and ecological implications of “specialized” pollinator rewards,” in Evolution of Plant-Pollinator Relationships. Ed. Patiny, S. Systematics Association Special Volume Series (Cambridge University Press, Cambridge), 44–67.
Atanacković Krstonošić, M., Cvejić Hogervorst, J., Krstonošić, V., Mikulić, M. (2019). Phenolic content and in vitro antioxidant capacity of mono-and polyfloral honeys originating from Serbia. Food Feed Res. 46, 83–89. doi: 10.5937/FFR1901083A
Atlagić, J., Joksimović, J., Sakač, Z., Miklič, V., Dušanić, N. (2003). Mode of inheritance and heritability of disc flower corolla length and nectar content in sunflower. Genetika 35, 59–65. doi: 10.2298/GENSR0301059A
Bahmani, K., Giguere, M., Dowell, J. A., Mason, C. M. (2023). Germplasm diversity of sunflower volatile terpenoid profiles across vegetative and reproductive organs. Agron. Res. 21, 4–27. doi: 10.15159/AR.22.084
Bahmani, K., Robinson, A., Majumder, S., LaVardera, A., Dowell, J. A., Goolsby, E. W., et al. (2022). Broad diversity in monoterpene-sesquiterpene balance across wild sunflowers: Implications of leaf and floral volatiles for biotic interactions. Am. J. Bot. 109, 2051–2067. doi: 10.1002/ajb2.16093
Baker, H., Baker, I. (1973). Amino-acids in the nectar and their evolutionary significance. Nature 241, 543–545. doi: 10.1038/241543b0
Balvino-Olvera, F. J., Olivares-Pinto, U., González-Rodríguez, A., Aguilar-Aguilar, M. J., Ruiz-Guzmán, G., Lobo-Segura, J., et al. (2024). Effects of floral resources on honey bee populations in Mexico: Using dietary metabarcoding to examine landscape quality in agroecosystems. Ecol. Evolution. 14, e11456. doi: 10.1002/ece3.11456
Baracchi, D., Marples, A., Jenkins, A. J., Leitch, A. R., Chittka, L. (2017). Nicotine in floral nectar pharmacologically influences bumblebee learning of floral features. Sci. Rep. 7, 1951. doi: 10.1038/s41598-017-01980-1
Barberis, M., Calabrese, D., Galloni, M., Nepi, M. (2023). Secondary metabolites in nectar-mediated plant-pollinator relationships. Plants 12, 550. doi: 10.3390/plants12030550
Barstow, A. C., Prasifka, J. R., Attia, Z., Kane, N. C., Hulke, B. S. (2022). Genetic mapping of a pollinator preference trait: Nectar volume in sunflower (Helianthus annuus L.). Front. Plant Sci. 13. doi: 10.3389/fpls.2022.1056278
Bath, P. K., Singh, N. (1999). A comparison between Helianthus annuus and Eucalyptus lanceolatus honey. Food Chem. 67, 389–397. doi: 10.1016/S0308-8146(99)00132-6
Bayram, N. E., Gercek, Y. C., Çelik, S., Mayda, N., Kostić, A., Dramićanin, A. M., et al. (2021). Phenolic and free amino acid profiles of bee bread and bee pollen with the same botanical origin – similarities and differences. Arabian J. Chem. 14, 103004. doi: 10.1016/j.arabjc.2021.103004
Bergonzoli, S., Romano, E., Beni, C., Latterini, F., Lo Scalzo, R., Scarfone, A. (2022). Nectar dynamics and pollinators preference in sunflower. Insects 13, 717. doi: 10.3390/insects13080717
Bertoli, A., Fambrini, M., Doveri, S., Leonardi, M., Pugliesi, C., Pistelli, L. (2011). Pollen aroma fingerprint of two sunflower (Helianthus annuus L.) genotypes characterized by different pollen colors. Chem. Biodiv. 8 (9), 1766–1775. doi: 10.1002/cbdv.201100045
Blight, M. M., Métayer, M. L., Pham-Delègue, M. H., Pickett, J. A., Marion-Poll, F., Wadhams, L. J. (1997). Identification of Floral Volatiles Involved in Recognition of Oilseed Rape Flowers, Brassica napus by Honeybees, Apis mellifera. J. Chem. Ecol. 23, 1715–1727. doi: 10.1023/B:JOEC.0000006446.21160.c1
Bobiş, O., Bonta, V., Cornea-Cipcigan, M., Nayik, G. A., Dezmirean, D. S. (2021). Bioactive molecules for discriminating Robinia and Helianthus honey: high-performance liquid chromatography-electron spray ionization-mass spectrometry polyphenolic profile and physicochemical determinations. Molecules 26, 4433–4453. doi: 10.3390/molecules26154433
Brentan Silva, D., Aschenbrenner, A.-K., Lopes, N. P., Spring, O. (2017). Direct analyses of secondary metabolites by mass spectrometry imaging (MSI) from sunflower (Helianthus annuus L.) trichomes. Molecules 22, 774. doi: 10.3390/molecules22050774
Brown, J., Cunningham, S. A. (2019). Global-scale drivers of crop visitor diversity and the historical development of agriculture. Proc. R. Soc B. 286, 20192096. doi: 10.1098/rspb.2019.2096
Bryś, M. S., Strachecka, A. (2024). The key role of amino acids in pollen quality and honey bee physiology-A review. Molecules 29, 2605. doi: 10.3390/molecules29112605
Burger, H., Dotterl, S., Ayasse, M. (2010). Host-plant finding and recognition by visual and olfactory floral cues in an oligolectic bee. Funct. Ecol. 24, 1234–1240. doi: 10.1111/j.1365-2435.2010.01744.x
Canto, A., Herrera, C. M. (2012). Micro-organisms behind the pollination scenes: Microbial imprint on floral nectar sugar variation in a tropical plant community. Ann. Botany. 110, 1173–1183. doi: 10.1093/aob/mcs183
Carter, C., Shafir, S., Yehonatan, L., Palmer, R. G., Thornburg, R. (2006). A novel role for proline in plant floral nectars. Die Naturwissenschaften. 93, 72–79. doi: 10.1007/s00114-005-0062-1
Castiglioni, S., Stefano, M., Astolfi, P., Carloni, P. (2017). Chemometric approach to the analysis of antioxidant properties and colour of typical Italian monofloral honeys. Int. J. Food Sci. Technol. 52, 1138–1146. doi: 10.1111/ijfs.13397
Cerrutti, N., Pontet, C. (2016). Differential attractiveness of sunflower cultivars to the honeybee Apis mellifera L. OCL 23, D204. doi: 10.1051/ocl/2016005
Ceuppens, B., Ameye, M., Van Langenhove, H., Roldan-Ruiz, I., Smagghe, G. (2015). Characterization of volatiles in strawberry varieties ‘Elsanta’ and ‘Sonata’ and their effect on bumblebee flower visiting. Arthropod-Plant Interactions. 9, 281–287. doi: 10.1007/s11829-015-9375-y
Chabert, S., Mallinger, R. E., Senechal, C., Fougeroux, A., Geist, O., Guillemard, V., et al. (2022). Importance of maternal resources in pollen limitation studies with pollinator gradients: A case study with sunflower. Agricul. Ecosyst. Environ. 330, 107887. doi: 10.1016/j.agee.2022.107887
Chabert, S., Sénéchal, C., Fougeroux, A., Pousse, J., Richard, F., Nozières, E., et al. (2020). Effect of environmental conditions and genotype on nectar secretion in sunflower (Helianthus annuus L.). OCL 27, 51. doi: 10.1051/ocl/2020040
Chambó, E. D., Garcia, R. C., Escocard de Oliveira, N. T., Duarte-Júnior, J. B. (2011). Honey bee visitation to sunflower: effects on pollination and plant genotype. Sci. Agric. 68, 647–651. doi: 10.1590/S0103-90162011000600007
Charrière, J.−D., Imdorf, A., Koenig, C., Gallmann, S., Kuhn, R. (2010). Do sunflowers influence the development of honey bee, Apis mellifera, colonies in areas with diversified crop farming? J. Apicultural Res. 49, 227–235. doi: 10.3896/IBRA.1.49.3.01
Chittka, L., Raine, N. E. (2006). Recognition of flowers by pollinators. Curr. Opin. Plant Biol. 9, 428–435. doi: 10.1016/j.pbi.2006.05.002
Chou, J.−C., Mullin, C. A. (1993). Phenologic and tissue distribution of sesquiterpene lactones in cultivated sunflower (Helianthus annuus L.). J. Plant Physiol. 142, 657–663. doi: 10.1016/S0176-1617(11)80898-9
Christensen, S. M., Munkres, I., Vannette, R. L. (2021). Nectar bacteria stimulate pollen germination and bursting to enhance microbial fitness. Curr. Biol. 31, 4373–4380.e6. doi: 10.1016/j.cub.2021.07.016
Čižmárik, L., Matel, I. (1970). Examination of the chemical composition of propolis. 1: Isolation and identification of 3, 4 dihydroxycinnamic acid (caffeic acid) from propolis. Experientia 26, 713. doi: 10.1007/BF02232498
Conti, I., Medrzycki, P., Argenti, C., Meloni, M., Vecchione, V., Boi, M., et al. (2016). Sugar and protein content in different monofloral pollens - building a database. Bull. Insectoly. 69, 318–320. doi: 10.1007/s13592-016-0435-9
Cotte, J. F., Casabianca, H., Chardon, S., Lheritier, J., Grenier-Loustalot, M. F. (2004a). Chromatographic analysis of sugars applied to the characterisation of monofloral honey. Analytical Bioanalytical Chem. 380, 698–705. doi: 10.1007/s00216-004-2764-1
Cotte, J. F., Casabianca, H., Giroud, B., Albert, M., Lheritier, J., Grenier-Loustalot, M. F. (2004b). Characterization of honey amino acid profiles using high-pressure liquid chromatography to control authenticity. Analytical Bioanalytical Chem. 378, 1342–1350. doi: 10.1007/s00216-003-2430-z
Cruden, R. W., Hermann, S. M. (1983). “Studying nectar? Some observations on the art,” in The Biology of Nectaries. Eds. Bentley, B., Elias, T. (Columbia University Press, New York), 223–241.
Degirmenci, A., Yildiz, O., Boyraci, G. M., Er Kemal, M., Simsek, O. (2024). The process of pollen transformation into bee bread: Changes in bioactivity, bioaccessibility, and microbial dynamics. Food Funct. 15, 2550–2562. doi: 10.1039/d3fo04466a
De Groot, A. P. (1953). Protein and amino acid requirements of the honey bee (Apis mellifera L.). Physiol. Comp. Oecol. 3, 197–285.
Devillers, J., Morlot, M., Pham-Delègue, M. H., Doré, J. C. (2004). Classification of monofloral honeys based on their quality control data. Food Chem. 86, 305–312. doi: 10.1016/j.foodchem.2003.09.029
Dimitrova, B., Gevrenova, R., Anklam, E. (2007). Analysis of phenolic acids in honeys of different floral origin by solid-phase extraction and high-performance liquid chromatography. Phytochemical Analysis. 18, 24–32. doi: 10.1002/pca.948
Di Pasquale, G., Alaux, C., Le Conte, Y., Odoux, J. F., Pioz, M., Vaissière, B. E., et al. (2016). Variations in the availability of pollen resources affect honey bee health. PLoS One 11, e0162818. doi: 10.1371/journal.pone.0162818
Di Pasquale, G., Salignon, M., Le Conte, Y., Belzunces, L. P., Decourtye, A., Kretzschmar, A., et al. (2013). Influence of pollen nutrition on honey bee health: do pollen quality and diversity matter? PLoS One 8, e72016. doi: 10.1371/journal.pone.0072016
Dolezal, A. G., St. Clair, A. L., Zhang, G., Toth, A. L., O’Neal, M. E. (2019). Native habitat mitigates feast–famine conditions faced by honey bees in an agricultural landscape. PNAS 116, 25147–25155. doi: 10.1073/pnas.1912801116
Donkersley, P., Rhodes, G., Pickup, R. W., Jones, K. C., Wilson, K. (2014). Honeybee nutrition is linked to landscape composition. Ecol. Evolution. 4, 4195–4206. doi: 10.1002/ece3.1293
du Toit, A. P., Coetzer, L. A. (1991). Difference in potential attractiveness to bees of seventeen South African sunflower (Helianthus annuus) cultivars. Acta Horticulturae. 288, 288–293. doi: 10.17660/ActaHortic.1991.288.45
Emin Duru, M., Eroğlu, B., Tel-Çayan, G., Taş-Küçükaydın, M., Küçükaydın, S., Çayan, F., et al. (2023). HPLC-DAD analysis and versatile bioactivities of turkish sunflower honeys using chemometric approaches. Chem. Biodiversity. 20, e202300486. doi: 10.1002/cbdv.202300486
Erler, S., Cotter, S. C., Freitak, D., Koch, H., Palmer-Young, E. C., de Roode, J. C., et al. (2024). Insects’ essential role in understanding and broadening animal medication. Trends Parasitol. 40, 338–349. doi: 10.1016/j.pt.2024.02.003
Erler, S., Denner, A., Bobiş, O., Forsgren, E., Moritz, R. F. A. (2014). Diversity of honey stores and their impact on pathogenic bacteria of the honeybee, Apis mellifera. Ecol. Evolution. 4, 3960–3967. doi: 10.1002/ece3.1252
Erler, S., Moritz, R. F. A. (2016). Pharmacophagy and pharmacophory: mechanisms of self-medication and disease prevention in the honeybee colony (Apis mellifera). Apidologie 47, 389–411. doi: 10.1007/s13592-015-0400-z
Estravis Barcala, M. C., Palottini, F., Farina, W. M. (2019). Honey bee and native solitary bee foraging behavior in a crop with dimorphic parental lines. PLoS One 14, e0223865. doi: 10.1371/journal.pone.0223865
Estravis-Barcala, M. C., Palottini, F., Farina, W. M. (2021). Learning of a mimic odor combined with nectar nonsugar compounds enhances honeybee pollination of a commercial crop. Sci. Rep. 11, 23918. doi: 10.1038/s41598-021-03305-9
European Commission, Joint Research Centre (JRC) (2022). “EUCROPMAP 2022,” in European Commission, Joint Research Centre (JRC). doi: 10.2905/555e5d1d-1aae-4320-a716-2e6d18aa1e7c
FAO (2024). FAOSTAT: Crops and livestock products (Sunflower seed). Available online at: www.fao.org/faostat/en/data/QCL/visualize (Accessed 06 March 2024).
Farag, R. S., Ahmed, A. I., Rashad, S. E., Ewies, M. A. (1980). Unsaponifiable matter of six pollens collected by honeybees in Egypt. J. Apicultural Res. 19, 248–254. doi: 10.1080/00218839.1980.11100032
Farina, W. M., Arenas, A., Díaz, P. C., Martin, C. S., Barcala, M. C. E. (2020). Learning of a mimic odor within beehives improves pollination service efficiency in a commercial crop. Curr. Biol. 30, 4284–4290. doi: 10.1016/j.cub.2020.08.018
Farina, W. M., Arenas, A., Estravis-Barcala, M. C., Palottini, F. (2023). Targeted crop pollination by training honey bees: advances and perspectives. Front. Bee Sci. 1. doi: 10.3389/frbee.2023.1253157
Fatrcová-Šramková, K., Nôžková, J., Máriássyová, M., Kačániová, M. (2016). Biologically active antimicrobial and antioxidant substances in the Helianthus annuus L. Bee pollen. J. Environ. Sci. Health Part. B Pesticides Food Contaminants Agric. Wastes 51, 176–181. doi: 10.1080/03601234.2015.1108811
Fell, R. D. (1986). Foraging Behaviors of Apis mellifera L. and Bombus spp. on Oilseed Sunflower (Helianthus annuus L.). J. Kansas Entomological Society. 59, 72–81.
Ferguson, B., Mallinger, R. E., Prasifka, J. R. (2021). Bee community composition, but not diversity, is influenced by floret size in cultivated sunflowers. Apidologie 52, 1210–1222. doi: 10.1007/s13592-021-00897-z
Ferguson, B., Prasifka, J. R., Carroll, M. J., Corby-Harris, V., DeGrandi-Hoffman, G. (2024). Honey bee (Apis mellifera L.) foraging rewards in sunflowers: effect of floral traits on visitation and variation in pollen quality over two consecutive years. J. Apicultural Res. 63, 1–9. doi: 10.1080/00218839.2024.2364948
Fernandes-da-Silva, P. G., Serrão, J. E. (2000). Nutritive value and apparent digestibility of bee-collected and bee-stored pollen in the stingless bee, Scaptotrigona postica Latr. (Hymenoptera, Apidae, Meliponini). Apidologie 31, 39–45. doi: 10.1051/apido:2000100
Ferreres, F., Tomás-Barberán, F. A., Soler, C., García-Viguera, C., Ortiz, A., Tomás-Lorente, F. (1994). A simple extractive technique for honey flavonoid HPLC analysis. Apidologie 25, 21–30. doi: 10.1051/apido:19940103
Figueroa, L. L., Fowler, A., Lopez, S., Amaral, V. E., Koch, H., Stevenson, P. C., et al. (2023). Sunflower spines and beyond: Mechanisms and breadth of pollen that reduce gut pathogen infection in the common eastern bumble bee. Funct. Ecol. 37, 1757–1769. doi: 10.1111/1365-2435.14320
Filipiak, M. (2019). Key pollen host plants provide balanced diets for wild bee larvae: A lesson for planting flower strips and hedgerows. J. Appl. Ecol. 56, 1410–1418. doi: 10.1111/1365-2664.13383
Filipiak, Z. M., Filipiak, M. (2020). The scarcity of specific nutrients in wild bee larval food negatively influences certain life history traits. Biology 9, 462. doi: 10.3390/biology9120462
Filipiak, M., Kuszewska, K., Asselman, M., Denisow, B., Stawiarz, E., Woyciechowski, M., et al. (2017). Ecological stoichiometry of the honeybee: Pollen diversity and adequate species composition are needed to mitigate limitations imposed on the growth and development of bees by pollen quality. PLoS One 12, e0183236. doi: 10.1371/journal.pone.0183236
Filipiak, M., Shields, M. W., Cairns, S. M., Grainger, M. N. C., Wratten, S. D. (2022). The conserved and high K-to-Na ratio in sunflower pollen: Possible implications for bee health and plant-bee interactions. Front. Plant Sci. 13. doi: 10.3389/fpls.2022.1042348
Fitch, G., Figueroa, L. L., Koch, H., Stevenson, P. C., Adler, L. S. (2022). Understanding effects of floral products on bee parasites: Mechanisms, synergism, and ecological complexity. Int. J. Parasitol.: Parasites Wildlife 17, 244–256. doi: 10.1016/j.ijppaw.2022.02.011
Fowler, A. E., Giacomini, J. J., Connon, S. J., Irwin, R. E., Adler, L. S. (2022a). Sunflower pollen reduces a gut pathogen in the model bee species, Bombus impatiens, but has weaker effects in three wild congeners. Proc. R. Soc B. 289, 20211909. doi: 10.1098/rspb.2021.1909
Fowler, A. E., Kola, E., Adler, L. S. (2023). The effect of sunflower pollen age and origin on pathogen infection in the common eastern bumble bee (Apidae: Hymenoptera). J. Economic Entomol. 116, 1939–1942. doi: 10.1093/jee/toad154
Fowler, A. E., Sadd, B. M., Bassingthwaite, T., Irwin, R. E., Adler, L. S. (2022b). Consuming sunflower pollen reduced pathogen infection but did not alter measures of immunity in bumblebees. Philos. Trans. R. Soc. London. Ser. B Biol. Sci. 377, 20210160. doi: 10.1098/rstb.2021.0160
Fowler, A. E., Stone, E. C., Irwin, R. E., Adler, L. S. (2020). Sunflower pollen reduces a gut pathogen in worker and queen but not male bumble bees. Ecol. Entomol. 45, 1318–1326. doi: 10.1111/een.12915
Frachon, L., Stirling, S. A., Schiestl, F. P., Dudareva, N. (2021). Combining biotechnology and evolution for understanding the mechanisms of pollinator attraction. Curr. Opin. Biotechnol. 70, 213–219. doi: 10.1016/j.copbio.2021.06.004
Freund, D. E., Furgala, B. (1982). Effect of pollination by insects on the seed set and yield of ten oilseed sunflower cultivars. Am. Bee J. 122, 648–652.
Galisteo, A., Pérez Rodríguez, Á., González, A., Barrero, A. F., Del Quílez Moral, J. F. (2024). Terpenoid diversity in sunflower (Helianthus annuus L.) and their potential in crop protection. Phytochem. Rev. 23, 583–623. doi: 10.1007/s11101-023-09903-x
Gekière, A., Semay, I., Gérard, M., Michez, D., Gerbaux, P., Vanderplanck, M. (2022). Poison or potion: effects of sunflower phenolamides on bumble bees and their gut parasite. Biology 11, 545. doi: 10.3390/biology11040545
Gherman, B. I., Denner, A., Bobiş, O., Dezmirean, D. S., Mărghitaş, L. A., Schlüns, H., et al. (2014). Pathogen-associated self-medication behavior in the honeybee Apis mellifera. Behav. Ecol. Sociobiol. 68, 1777–1784. doi: 10.1007/s00265-014-1786-8
Ghosh, S., Namin, S. M., Jung, C. (2022). Differential bacterial community of bee bread and bee pollen revealed by 16s rRNA high-throughput sequencing. Insects 13, 863. doi: 10.3390/insects13100863
Giacomini, J. J., Adler, L. S., Reading, B. J., Irwin, R. E. (2023). Differential bumble bee gene expression associated with pathogen infection and pollen diet. BMC Genomics 24, 157. doi: 10.1186/s12864-023-09143-5
Giacomini, J. J., Connon, S. J., Marulanda, D., Adler, L. S., Irwin, R. E. (2021). The costs and benefits of sunflower pollen diet on bumble bee colony disease and health. Ecosphere 12, e03663. doi: 10.1002/ecs2.3663
Giacomini, J. J., Leslie, J., Tarpy, D. R., Palmer-Young, E. C., Irwin, R. E., Adler, L. S. (2018). Medicinal value of sunflower pollen against bee pathogens. Sci. Rep. 8, 14394. doi: 10.1038/s41598-018-32681-y
Goulson, D., Nicholls, E., Botías, C., Rotheray, E. L. (2015). Bee declines driven by combined stress from parasites, pesticides, and lack of flowers. Science 347, 1255957. doi: 10.1126/science.1255957
Haber, A. I., Sims, J. W., Mescher, M. C., De Moraes, C. M., Carr, D. E. (2019). A key floral scent component (β-trans-bergamotene) drives pollinator preferences independently of pollen rewards in seep monkeyflower. Funct. Ecol. 33, 218–228. doi: 10.1111/1365-2435.13246
Hadisoesilo, S., Furgala, B. (1986). The effect of cultivar, floral stage and time of day on the quantity and quality of nectar extracted from oilseed sunflower (Helianthus annuus L.) in minnesota. Am. Bee J., 630–632.
Harris, C., Balfour, N. J., Ratnieks, F. L. (2024). Seasonal variation in the general availability of floral resources for pollinators in northwest Europe: A review of the data. Biol. Conserv. 298, 110774. doi: 10.1016/j.biocon.2024.110774
Haydak, M. H. (1970). Honey bee nutrition. Annu. Rev. Entomol. 15, 143–157. doi: 10.1146/annurev.en.15.010170.001043
Heil, M. (2011). Nectar: Generation, regulation and ecological functions. Trends Plant Sci. 16, 191–200. doi: 10.1016/j.tplants.2011.01.003
Heiser, C. B., Smith, D. M., Clevenger, S. B., Martin, W. C. (1969). The north american sunflowers (Helianthus). Memoirs Torrey Botanical Club. 22, 1–218.
Hendriksma, H. P., Oxman, K. L., Shafir, S. (2014). Amino acid and carbohydrate tradeoffs by honey bee nectar foragers and their implications for plant-pollinator interactions. J. Insect Physiol. 69, 56–64. doi: 10.1016/j.jinsphys.2014.05.025
Herbert, E. W., Jr., Shimanuki, H. (1978). Chemical composition and nutritive value of bee-collected and bee-stored pollen. Apidologie 9, 33–40. doi: 10.1051/apido:19780103
Hernández, I. G., Palottini, F., Macri, I., Galmarini, C. R., Farina, W. M. (2019). Appetitive behavior of the honey bee Apis mellifera in response to phenolic compounds naturally found in nectars. J. Exp. Biol. 222, jeb189910. doi: 10.1242/jeb.189910
Holzschuh, A., Dormann, C. F., Tscharntke, T., Steffan-Dewenter, I. (2013). Mass-flowering crops enhance wild bee abundance. Oecologia 172, 477–484. doi: 10.1007/s00442-012-2515-5
Horth, L., Campbell, L., Bray, R. (2014). Wild bees preferentially visit Rudbeckia flower heads with exaggerated ultraviolet absorbing floral guides. Biol. Open 3, 221–230. doi: 10.1242/bio.20146445
Human, H., Nicolson, S. W., Strauss, K., Pirk, C. W. W., Dietemann, V. (2007). Influence of pollen quality on ovarian development in honeybee workers (Apis mellifera scutellata). J. Insect Physiol. 53, 649–655. doi: 10.1016/j.jinsphys.2007.04.002
Ion, N., Ştefan, V., Ion, V., Fota, G., Coman, R. (2007). Results concerning the melliferous characteristics of the sunflower hybrids cultivated in Romania. Lucrări Ştiinţifice Zootehnie Şi Biotehnologii. 40, 80–90.
Isopescu, R. D., Joseanu, A. M., Minca, I., Colta, T., Postelnicescu, P., Matescu, C. (2014). Characterization of Romanian honey based on physico-chemical properties and multivariate analysis. Rev. Chimie. 65, 381–385.
Iwashina, T. (2015). Contribution to flower colors of flavonoids including anthocyanins: A review. Natural Product Commun. 10, 529–544. doi: 10.1177/1934578X1501000335
Jeannerod, L., Carlier, A., Schatz, B., Daise, C., Richel, A., Agnan, Y., et al. (2022). Some bee-pollinated plants provide nutritionally incomplete pollen amino acid resources to their pollinators. PLoS One 17, e0269992. doi: 10.1371/journal.pone.0269992
Jiang, W., Jiang, Q., Shui, Z., An, P., Shi, S., Liu, T., et al. (2024). Hamyba-HabHLH1 regulatory complex and HaMYBF fine-tune red flower coloration in the corolla of sunflower (Helianthus annuus L.). Plant Sci. 338, 111901. doi: 10.1016/j.plantsci.2023.111901
Jocković, J., Grahovac, N., Milovac, Ž., Jocković, M., Jocić, S., Marjanović Jeromela, A., et al. (2025). Exploring Helianthus species for resilience to drought during the critical reproductive stage. Plants 14, 631–650. doi: 10.3390/plants14040631
Jocković, J., Rajčević, N., Zorić, L., Jocković, M., Radanović, A., Cvejić, S., et al. (2024). Secretory tissues and volatile components of disc florets in several wild Helianthus L. Species. Plants 13, 345–365. doi: 10.3390/plants13030345
Joksimović, J., Atlagić, J., Sakač, Z., Miklič, V., Dušanić, N. (2003). Phenotypic and genotypic variability of disc flower corolla length and nectar content in sunflower. Genetika 35, 131–138. doi: 10.2298/GENSR0302131J
Juan-Borrás, M., Domenech, E., Hellebrandova, M., Escriche, I. (2014). Effect of country origin on physicochemical, sugar and volatile composition of acacia, sunflower and tilia honeys. Food Res. Int. 60, 86–94. doi: 10.1016/j.foodres.2013.11.045
Kádár, M., Juan-Borrás, M., Hellebrandova, M., Doménech, E., Escriche, I. (2010). Differentiation of acacia, sunflower and tilia honeys from different countries based on sugar composition, physicochemical and color parameters. Bulletin of university of agricultural sciences and veterinary medicine Cluj-Napoca. Agriculture 67, 1843–5386. doi: 10.15835/buasvmcn-agr:5084
Kantar, M. B., Sosa, C. C., Khoury, C. K., Castaneda-Alvarez, N. P., Achicanoy, H. A., Bernau, V., et al. (2015). Ecogeography and utility to plant breeding of the crop wild relatives of sunflower (Helianthus annuus L.). Front. Plant Sci. 6. doi: 10.3389/fpls.2015.00841
Kaplan, M., Karaoglu, Ö., Eroglu, N., Silici, S. (2016). Fatty acid and proximate composition of bee bread. Food Technol. Biotechnol. 54, 497–504. doi: 10.17113/ftb.54.04.16.4635
Kečkeš, J., Trifković, J., Andrić, F., Jovetić, M., Tešić, Z., Milojković-Opsenica, D. (2013). Amino acids profile of Serbian unifloral honeys. J. Sci. Food Agriculture. 93, 3368–3376. doi: 10.1002/jsfa.6187
Khongkarat, P., Phuwapraisirisan, P., Chanchao, C. (2022). Phytochemical content, especially spermidine derivatives, presenting antioxidant and antilipoxygenase activities in Thai bee pollens. PeerJ 10, e13506. doi: 10.7717/peerj.13506
Klatt, B. K., Burmeister, C., Westphal, C., Tscharntke, T., von Fragstein, M. (2013). Flower volatiles, crop varieties and bee responses. PloS One 8, e72724. doi: 10.1371/journal.pone.0072724
Knauer, A. C., Schiestl, F. P. (2015). Bees use honest floral signals as indicators of reward when visiting flowers. Ecol. Letters. 18, 135–143. doi: 10.1111/ele.12386
Knoerr, S. A., Rivest, S., Hotchkiss, M. Z., Forrest, J. R. K. (2024). Impacts of Asteraceae pollen spines on bumble bee survival and larval growth. Arthropod-Plant Interactions. 18, 417–424. doi: 10.1007/s11829-024-10058-5
Knudsen, J. T., Tollsten, L. (1993). Trends in floral scent chemistry in pollination syndromes: floral scent composition in moth-pollinated taxa. Bot. J. Linn. Soc 113, 263–284. doi: 10.1006/bojl.1993.1073
Kostić, A.Ž., Milinčić, D. D., Gašić, U. M., Nedić, N., Stanojević, S. P., Tešić, Ž.L., et al. (2019). Polyphenolic profile and antioxidant properties of bee-collected pollen from sunflower (Helianthus annuus L.) plant. LWT- Food Sci. Technol. 112, 108244. doi: 10.1016/j.lwt.2019.06.011
Kostić, A., Milinčić, D. D., Trifunović, B.D.Š., Stanojević, S. P., Lević, S., Nedić, N., et al. (2020). Nutritional and techno-functional properties of monofloral bee-collected sunflower (Helianthus annuus L.) pollen. Emirates J. Food Agriculture. 32, 768–777. doi: 10.9755/ejfa.2020.v32.i11.2188
Kunat-Budzyńska, M., Rysiak, A., Wiater, A., Grąz, M., Andrejko, M., Budzyński, M., et al. (2023). Chemical composition and antimicrobial activity of new honey varietals. Int. J. Environ. Res. Public Health 20, 2458. doi: 10.3390/ijerph20032458
Kyselka, J., Bleha, R., Dragoun, M., Bialasová, K., Horáčková, Š., Schätz, M., et al. (2018). Antifungal polyamides of hydroxycinnamic acids from sunflower bee pollen. J. Agric. Food Chem. 66, 11018–11026. doi: 10.1021/acs.jafc.8b03976
Lau, P. W., Esquivel, I. L., Parys, K. A., Hung, K. L. J., Chakrabarti, P. (2023). The nutritional landscape in agroecosystems: a review on how resources and management practices can shape pollinator health in agricultural environments. Ann. Entomological Soc. America 116, 261–275. doi: 10.1093/aesa/saad023
Lau, P., Lesne, P., Grebenok, R. J., Rangel, J., Behmer, S. T. (2022). Assessing pollen nutrient content: A unifying approach for the study of bee nutritional ecology. Philos. Trans. R. Soc. London. Ser. B Biol. Sci. 377, 20210510. doi: 10.1098/rstb.2021.0510
Lazarević, K. B., Andrić, F., Trifković, J., Tešić, Ž., Milojković-Opsenica, D. (2012). Characterisation of Serbian unifloral honeys according to their physicochemical parameters. Food Chem. 132, 2060–2064. doi: 10.1016/j.foodchem.2011.12.048
Leach, M., Dibble, A. C., Stack, L. B., Perkins, L. B., Drummond, F. A. (2021). The effect of plant nutrition on bee flower visitation. J. Kansas Entomological Society. 94, 277–300. doi: 10.2317/0022-8567-94.4.277
Leonard, A. S., Dornhaus, A., Papaj, D. R., Patiny, S. (2012). “Why are floral signals complex? An outline of functional hypotheses,” in Evolution of Plant-Pollinator Relationships. Ed. Patiny, S. (Cambridge, United Kingdom: Cambridge University Press), 261–282.
Leonard, A. S., Papaj, D. R. (2011). ‘X’ marks the spot: The possible benefits of nectar guides to bees and plants. Funct. Ecol. 25, 1293–1301. doi: 10.1111/j.1365-2435.2011.01885.x
Leponiemi, M., Freitak, D., Moreno-Torres, M., Pferschy-Wenzig, E. M., Becker-Scarpitta, A., Tiusanen, M., et al. (2023). Honeybees’ foraging choices for nectar and pollen revealed by DNA metabarcoding. Sci. Rep. 13, 14753. doi: 10.1038/s41598-023-42102-4
Li, S., Yaermaimaiti, S., Tian, X.−M., Wang, Z.−W., Xu, W.−J., Luo, J., et al. (2021). Dynamic metabolic and transcriptomic profiling reveals the biosynthetic characteristics of hydroxycinnamic acid amides (HCAAs) in sunflower pollen. Food Res. Int. 149, 110678. doi: 10.1016/j.foodres.2021.110678
Lin, S., Mullin, C. A. (1999). Lipid, polyamide, and flavonol phagostimulants for adult western corn rootworm from sunflower (Helianthus annuus L.) pollen. J. Agric. Food Chem. 47, 1223–1229. doi: 10.1021/jf980858w
Liu, F., Gao, J., Di, N., Adler, L. S. (2015). Nectar attracts foraging honey bees with components of their queen pheromones. J. Chem. Ecol. 41, 1028–1036. doi: 10.1007/s10886-015-0642-2
LoCascio, G. M., Aguirre, L., Irwin, R. E., Adler, L. S. (2019a). Pollen from multiple sunflower cultivars and species reduces a common bumblebee gut pathogen. R. Soc. Open Sci. 6, 190279. doi: 10.1098/rsos.190279
LoCascio, G. M., Pasquale, R., Amponsah, E., Irwin, R. E., Adler, L. S. (2019b). Effect of timing and exposure of sunflower pollen on a common gut pathogen of bumble bees. Ecol. Entomol. 44, 702–710. doi: 10.1111/een.12751
Ma, S., Zhou, H., Ren, T., Yu, E., Feng, B., Wang, J., et al. (2024). Integrated transcriptome and metabolome analysis revealed that HaMYB1 modulates anthocyanin accumulation to deepen sunflower flower color. Plant Cell Rep. 43, 74. doi: 10.1007/s00299-023-03098-3
Malfi, R. L., McFrederick, Q. S., Lozano, G., Irwin, R. E., Adler, L. S. (2023). Sunflower plantings reduce a common gut pathogen and increase queen production in common eastern bumblebee colonies. Proc. R. Soc B. 290, 20230055. doi: 10.1098/rspb.2023.0055
Mallinger, R. E., Bradshaw, J., Varenhorst, A. J., Prasifka, J. R. (2019). Native solitary bees provide economically significant pollination services to confection sunflowers (Helianthus annuus L.) (Asterales: Asteraceae) grown across the northern great plains. J. Economic Entomol. 112, 40–48. doi: 10.1093/jee/toy322
Mallinger, R., Prasifka, J. (2017a). Benefits of insect pollination to confection sunflowers differ across plant genotypes. Crop Sci. 57, 3264–3272. doi: 10.2135/cropsci2017.03.0148
Mallinger, R. E., Prasifka, J. R. (2017b). Bee visitation rates to cultivated sunflowers increase with the amount and accessibility of nectar sugars. J. Appl. Entomol. 141, 561–573. doi: 10.1111/jen.12375
Mandel, J. R., Dechaine, J. M., Marek, L. F., Burke, J. M. (2011). Genetic diversity and population structure in cultivated sunflower and a comparison to its wild progenitor, Helianthus annuus L. Theor. Appl. Genet. 123, 693–704. doi: 10.1007/s00122-011-1619-3
Manolova, V., Parvina, I., Yankovska–Stefanova, T., Balkanska, R. (2021). Physicochemical analysis of sunflower honey from Bulgaria. Uludağ Arıcılık Dergisi 21, 168–176. doi: 10.31467/uluaricilik.960751
Manson, J. S., Otterstatter, M. C., Thomson, J. D. (2010). Consumption of a nectar alkaloid reduces pathogen load in bumble bees. Oecologia 162, 81–89. doi: 10.1007/s00442-009-1431-9
Marić, A., Jovanov, P., Sakač, M., Novaković, A., Hadnađev, M., Pezo, L., et al. (2021). A comprehensive study of parameters correlated with honey health benefits. RSC Advances. 11, 12434–12441. doi: 10.1039/d0ra10887a
Martin, V. N., Schaeffer, R. N., Fukami, T. (2022). Potential effects of nectar microbes on pollinator health. Phil. Trans. R. Soc B. 377, 20210155. doi: 10.1098/rstb.2021.0155
Mateo, R., Bosch-Reig, F. (1997). Sugar profiles of Spanish unifloral honeys. Food Chem. 60, 33–41. doi: 10.1016/S0308-8146(96)00297-X
Mateo, R., Bosch-Reig, F. (1998). Classification of spanish unifloral honeys by discriminant analysis of electrical conductivity, color, water content, sugars, and pH. J. Agric. Food Chem. 46, 393–400. doi: 10.1021/jf970574w
Mattonai, M., Parri, E., Querci, D., Degano, I., Ribechini, E. (2016). Development and validation of an HPLC-DAD and HPLC/ESI-MS2 method for the determination of polyphenols in monofloral honeys from Tuscany (Italy). Microchemical J. 126, 220–229. doi: 10.1016/j.microc.2015.12.013
Mayda, N., Özkök, A., Ecem Bayram, N., Gerçek, Y. C., Sorkun, K. (2020). Bee bread and bee pollen of different plant sources: determination of phenolic content, antioxidant activity, fatty acid and element profiles. J. Food Measurement Characterization. 14, 1795–1809. doi: 10.1007/s11694-020-00427-y
McAulay, M. K., Forrest, J. R. K. (2019). How do sunflower pollen mixtures affect survival of queenless microcolonies of bumblebees (Bombus impatiens)? Arthropod-Plant Interactions. 13, 517–529. doi: 10.1007/s11829-018-9664-3
Mesquida, J., Marilleau, R., Pham-Delègue, M. H., Renard, M. (1988). A study of rapeseed (Brassica napus L. var. oleifera Metzger) flower nectar secretions. Apidologie 19, 307–318. doi: 10.1051/apido:19880309
Michez, D., Vanderplanck, M., Engel, M. S. (2012). “Fossil bees and their plant associates,” in Evolution of Plant-Pollinator Relationships. Ed. Patiny, S. (Cambridge, United Kingdom: Cambridge University Press), 103–164.
Milosavljević, S., Jadranin, M., Mladenović, M., Tešević, V., Menković, N., Mutavdžić, D., et al. (2021). Physicochemical parameters as indicators of the authenticity of monofloral honey from the territory of the Republic of Serbia. Macedonian J. Chem. Chem. Engineering. 40, 49. doi: 10.20450/mjcce.2021.2186
Moerman, R., Vanderplanck, M., Fournier, D., Jacquemart, A.−L., Michez, D. (2017). Pollen nutrients better explain bumblebee colony development than pollen diversity. Insect Conserv. Diversity. 10, 171–179. doi: 10.1111/icad.12213
Mohammed, S. E. A. R., Babiker, E. E. (2010). Identification of the floral origin of honey by amino acids composition. Aust. J. Basic Appl. Sci. 4, 552–556.
Molan, P. C. (1992). The antibacterial activity of honey: 1. The nature of the antibacterial activity. Bee World. 73, 5–28. doi: 10.1080/0005772X.1992.11099109
Moyers, B. T., Owens, G. L., Baute, G. J., Rieseberg, L. H. (2017). The genetic architecture of UV floral patterning in sunflower. Ann. Botany. 120, 39–50. doi: 10.1093/aob/mcx038
Mullin, C. A., Alfatafta, A. A., Harman, J. L., Everett, S. L., Serino, A. A. (1991). Feeding and toxic effects of floral sesquiterpene lactones, diterpenes, and phenolics from sunflower (Helianthus annuus L.) on western corn rootworm. J. Agric. Food Chem. 39, 2293–2299. doi: 10.1021/jf00012a041
Murray, E. A., Bossert, S., Danforth, B. N. (2018). Pollinivory and the diversification dynamics of bees. Biol. Letters. 14, 20180530. doi: 10.1098/rsbl.2018.0530
Muth, F., Breslow, P. R., Masek, P., Leonard, A. S. (2018). A pollen fatty acid enhances learning and survival in bumblebees. Behav. Ecol. 29, 1371–1379. doi: 10.1093/beheco/ary111
Muth, F., Philbin, C. S., Jeffrey, C. S., Leonard, A. S. (2022). Discovery of octopamine and tyramine in nectar and their effects on bumblebee behavior. IScience 25, 104765. doi: 10.1016/j.isci.2022.104765
Neff, J. I., Simpson, B. B. (1990). The roles of phenology and reward structure in the pollination biology of wild sunflower (Helianthus annuus L., asteraceae). Israel J. Botany. 39, 197–216. doi: 10.1080/0021213x.1990.10677144
Negri, P., Maggi, M. D., Ramirez, L., Feudis, L., Szwarski, N., Quintana, S., et al. (2015). Abscisic acid enhances the immune response in Apis mellifera and contributes to the colony fitness. Apidologie 46, 542–557. doi: 10.1007/s13592-014-0345-7
Nepi, M. (2017). New perspectives in nectar evolution and ecology: simple alimentary reward or a complex multiorganism interaction? Acta Agrobotanica. 70, 1704. doi: 10.5586/aa.1704
Nicolson, S. W. (2022). Sweet solutions: nectar chemistry and quality. Phil. Trans. R. Soc B. 377, 20210163. doi: 10.1098/rstb.2021.0163
Nicolson, S. W., Da Silva Das Neves, S., Human, H., Pirk, C. W. W. (2018). Digestibility and nutritional value of fresh and stored pollen for honey bees (Apis mellifera scutellata). J. Insect Physiol. 107, 302–308. doi: 10.1016/j.jinsphys.2017.12.008
Nicolson, S. W., Human, H. (2013). Chemical composition of the ‘low quality’ pollen of sunflower (Helianthus annuus, Asteraceae). Apidologie 44, 144–152. doi: 10.1007/s13592-012-0166-5
Omar, E., Abd-Ella, A. A., Khodairy, M. M., Moosbeckhofer, R., Crailsheim, K., Brodschneider, R. (2017). Influence of different pollen diets on the development of hypopharyngeal glands and size of acid gland sacs in caged honey bees (Apis mellifera). Apidologie 48, 425–436. doi: 10.1007/s13592-016-0487-x
Ördög, A., Tari, I., Zoltán, B., Poór, P. (2017). Mineral content analysis of unifloral honeys from the hungarian great plain. J. Elementology. 22, 271–281. doi: 10.5601/jelem.2016.21.2.1067
Oroian, M., Amariei, S., Rosu, A., Gutt, G. (2015). Classification of unifloral honeys using multivariate analysis. J. Essential Oil Res. 27, 533–544. doi: 10.1080/10412905.2015.1073183
Oroian, M., Ropciuc, S., Paduret, S., Sanduleac, E. T. (2017). Authentication of Romanian honeys based on physicochemical properties, texture and chemometric. J. Food Sci. Technol. 54, 4240–4250. doi: 10.1007/s13197-017-2893-0
Oroian, M., Sorina, R. (2017). Honey authentication based on physicochemical parameters and phenolic compounds. Comput. Electron. Agric. 138, 148–156. doi: 10.1016/j.compag.2017.04.020
Ortiz, P. L., Fernández-Díaz, P., Pareja, D., Escudero, M., Arista, M. (2021). Do visual traits honestly signal floral rewards at community level? Funct. Ecol. 35, 369–383. doi: 10.1111/1365-2435.13709
Oz, M., Karasu, A., Cakmak, I., Goksoy, A. T., Turan, Z. M. (2009). Effects of honeybee (Apis mellifera) pollination on seed set in hybrid sunflower (Helianthus annuus L.). Afr. J. Biotechnol. 8, 1037–1043.
Palmer-Young, E. C., Farrell, I. W., Adler, L. S., Milano, N. J., Egan, P. A., Junker, R. R., et al. (2019). Chemistry of floral rewards: intra- and interspecific variability of nectar and pollen secondary metabolites across taxa. Ecol. Monographs. 89, e01335. doi: 10.1002/ecm.1335
Palmer-Young, E. C., Malfi, R., Zhou, Y., Joyce, B., Whitehead, H., van Wyk, J. I., et al. (2023). Sunflower-associated reductions in varroa mite infestation of honey bee colonies. J. Economic Entomol. 116, 68–77. doi: 10.1093/jee/toac196
Palmer-Young, E. C., Tozkar, C.Ö., Schwarz, R. S., Chen, Y., Irwin, R. E., Adler, L. S., et al. (2017). Nectar and pollen phytochemicals stimulate honey bee (Hymenoptera: apidae) immunity to viral infection. J. Economic Entomol. 110, 1959–1972. doi: 10.1093/jee/tox193
Pamminger, T., Becker, R., Himmelreich, S., Schneider, C. W., Bergtold, M. (2019a). Pollen report: Quantitative review of pollen crude protein concentrations offered by bee pollinated flowers in agricultural and non-agricultural landscapes. PeerJ 7, e7394. doi: 10.7717/peerj.7394
Pamminger, T., Becker, R., Himmelreich, S., Schneider, C. W., Bergtold, M. (2019b). The nectar report: Quantitative review of nectar sugar concentrations offered by bee visited flowers in agricultural and non-agricultural landscapes. PeerJ 7, e6329. doi: 10.7717/peerj.6329
Pătruică, S., Alexa, E., Obiștioiu, D., Cocan, I., Radulov, I., Berbecea, A., et al. (2022). Chemical composition, antioxidant and antimicrobial activity of some types of honey from Banat Region, Romania. Molecules 27, 4179. doi: 10.3390/molecules27134179
Pauliuc, D., Oroian, M. (2020). Organic acids and physico-chemical parameters of Romanian sunflower honey. J. Faculty Food Engineering. 19, 148–155.
Penny, J. H. J. (1983). Nectar guide color contrast: a possible relationship with pollination strategy. New Phytologist. 95, 707–721. doi: 10.1111/j.1469-8137.1983.tb03534.x
Pernal, S. F., Currie, R. W. (2000). Pollen quality of fresh and 1-year-old single pollen diets for worker honey bees (Apis mellifera L.). Apidologie 31, 387–409. doi: 10.1051/apido:2000130
Persano Oddo, L., Bogdanov, S. (2004). Determination of honey botanical origin: problems and issues. Apidologie 35, S2–S3. doi: 10.1051/apido:2004044
Persano Oddo, L., Piazza, M., Sabatini, A., Accorti, M. (1995). Characterization of unifloral honeys. Apidologie 26, 453–465. doi: 10.1051/apido:19950602
Persano Oddo, L., Piro, R. (2004). Main European unifloral honeys: descriptive sheets. Apidologie 35, S38–S81. doi: 10.1051/apido:2004049
Pham-Delègue, M. H., Etiévant, P., Guichard, E., Marilleau, R., Douault, P., Chauffaille, J., et al. (1990). Chemicals involved in honeybee-sunflower relationship. J. Chem. Ecol. 16, 3053–3065. doi: 10.1007/BF00979612
Pham-Delègue, M. H., Loublier, Y., Ducruet, V., Douault, P., Marilleau, R., Etiévant, P. (1994). Caractérisation de Signaux Chimiques Impliqués dans les Relations Plantes-Abeilles Domestiques. Grana 33, 184–190. doi: 10.1080/00173139409428999
Pham-Delègue, M. H., Masson, C., Etiévant, P., Azar, M. (1986). Selective olfactory choices of the honeybee among sunflower aromas: A study by combined olfactory conditioning and chemical analysis. J. Chem. Ecol. 12, 781–793. doi: 10.1007/BF01012110
Pham-Delègue, M. H., Etievant, P., Masson, C. (1991). Allelochemicals Mediating Foraging Behaviour: the Bee-Sunflower Model, in The Behaviour and Physiology of Bees. Eds. Goodman, L. J., Fisher, R. C. (Wallingford, UK: CAB International on behalf of the Royal Entomological Society of London and the International Bee Research Association), pp. 163–184.
Pichersky, E., Raguso, R. A. (2018). Why do plants produce so many terpenoid compounds? New Phytologist. 220, 692–702. doi: 10.1111/nph.14178
Portlas, Z. M., Tetlie, J. R., Prischmann-Voldseth, D., Hulke, B. S., Prasifka, J. R. (2018). Variation in floret size explains differences in wild bee visitation to cultivated sunflowers. Plant Genet. Resources: Characterization Utilization. 16, 498–503. doi: 10.1017/S1479262118000072
Potts, S. G., Biesmeijer, J. C., Kremen, C., Neumann, P., Schweiger, O., Kunin, W. E. (2010). Global pollinator declines: trends, impacts and drivers. Trends Ecol. Evol. 25, 345–353. doi: 10.1016/j.tree.2010.01.007
Potts, S., Imperatriz-Fonseca, V., Ngo, H., Aizen, M. A., Biesmeijer, J. C., Breeze, T. D., et al. (2016). Safeguarding pollinators and their values to human well-being. Nature 540, 220–229. doi: 10.1038/nature20588
Prasifka, J. R., Ferguson, B., Fugate, K. K. (2023). Genotype and environment effects on sunflower nectar and their relationships to crop pollination. J. Pollination Ecol. 33, 54–63. doi: 10.26786/1920-7603(2023)719
Prasifka, J. R., Spring, O., Conrad, J., Cook, L. W., Palmquist, D. E., Foley, M. E. (2015). Sesquiterpene lactone composition of wild and cultivated sunflowers and biological activity against an insect pest. J. Agric. Food Chem. 63, 4042–4049. doi: 10.1021/acs.jafc.5b00362
Predescu, C., Papuc, C., Nicorescu, V. (2015). Antioxidant activity of sunflower and meadow honey. Sci. Works Ser. C. Veterinary Med. 61, 45–50.
Prŷs-Jones, O., Corbet, S. (2011). Bumblebees: Naturalists’ Handbooks 6. 3rd edition (Exeter: Pelagic Publishing).
Qiao, J., Feng, Z., Zhang, Y., Xiao, X., Dong, J., Haubruge, E., et al. (2023). Phenolamide and flavonoid glycoside profiles of 20 types of monofloral bee pollen. Food Chem. 405, 134800. doi: 10.1016/j.foodchem.2022.134800
Qu, H., Chuai, Z.−R., Zhang, W.−B., Zhang, J., Yun, X.−P., Liu, J., et al. (2024). Host selection by thrips is affected by the floral volatile profile of sunflower. Plant Cell Environ. 47, 4354–4368. doi: 10.1111/pce.15028
Quarrell, S. R., Weinstein, A. M., Hannah, L., Bonavia, N., Del Borrello, O., Flematti, G. R., et al. (2023). Critical pollination chemistry: Specific sesquiterpene floral volatiles in carrot inhibit honey bee feeding. J. Agric. Food Chem. 71, 16079–16089. doi: 10.1021/acs.jafc.3c03392
Quevedo-Caraballo, S., de Vega, C., Lievens, B., Fukami, T., Álvarez-Pérez, S. (2025). Tiny but mighty? Overview of a decade of research on nectar bacteria. New Phytol. 245, 1897–1910. doi: 10.1111/nph.20369
Raguso, R. A. (2008). Wake up and smell the roses: the ecology and evolution of floral scent. Annu. Rev. Ecol. Systematics. 39, 549–569. doi: 10.1146/annurev.ecolsys.38.091206.095601
Regali, A., Rasmont, P. (1995). New bioassays to evaluate diet in Bombus terrestris. Apidologie 26, 273–281. doi: 10.1051/apido:19950401
Requier, F., Odoux, J. F., Henry, M., Bretagnolle, V. (2017). The carry-over effects of pollen shortage decrease the survival of honeybee colonies in farmlands. J. Appl. Ecol. 54, 1161–1170. doi: 10.1111/1365-2664.12836
Requier, F., Odoux, J. F., Tamic, T., Moreau, N., Henry, M., Decourtye, A., et al. (2015). Honey bee diet in intensive farmland habitats reveals an unexpectedly high flower richness and a major role of weeds. Ecol. Applications. 25, 881–890. doi: 10.1890/14-1011.1
Rering, C. C., Rudolph, A. B., Beck, J. J. (2021). Pollen and yeast change nectar aroma and nutritional content alone and together, but honey bee foraging reflects only the avoidance of yeast. Environ. Microbiol. 23, 4141–4150. doi: 10.1111/1462-2920.15528
Richardson, L. L., Adler, L. S., Leonard, A. S., Andicoechea, J., Regan, K. H., Anthony, W. E., et al. (2015). Secondary metabolites in floral nectar reduce parasite infections in bumblebees. Proc. R. Soc B. 282, 20142471. doi: 10.1098/rspb.2014.2471
Rivest, S., Forrest, J. R. K. (2020). Defence compounds in pollen: Why do they occur and how do they affect the ecology and evolution of bees? New Phytologist. 225, 1053–1064. doi: 10.1111/nph.16230
Rivest, S., Muralidhar, M., Forrest, J. R. K. (2024). Pollen chemical and mechanical defences restrict host-plant use by bees. Proc. R. Soc B. 291, 20232298. doi: 10.1098/rspb.2023.2298
Rocchetti, G., Castiglioni, S., Maldarizzi, G., Carloni, P., Lucini, L. (2019). UHPLC - ESI - QTOF - MS phenolic profiling and antioxidant capacity of bee pollen from different botanical origin. Int. J. Food Sci. Technol. 54, 335–346. doi: 10.1111/ijfs.13941
Roulston, T. H., Cane, J. H., Buchmann, S. L. (2000). What governs protein content of pollen: pollinator preferences, pollen–pistil interactions, or phylogeny?. Ecological Monographs 70 (4), 617–643. doi: 10.1890/0012-9615(2000)070[0617:WGPCOP]2.0.CO;2
Roy, R., Schmitt, A. J., Thomas, J. B., Carter, C. J. (2017). Review: Nectar biology: From molecules to ecosystems. Plant Science: Int. J. Exp. Plant Biol. 262, 148–164. doi: 10.1016/j.plantsci.2017.04.012
Ruedenauer, F. A., Spaethe, J., van der Kooi, C. J., Leonhardt, S. D. (2019). Pollinator or pedigree: Which factors determine the evolution of pollen nutrients? Oecologia 191, 349–358. doi: 10.1007/s00442-019-04494-x
Russo, L., Vaudo, A. D., Fisher, C. J., Grozinger, C. M., Shea, K. (2019). Bee community preference for an invasive thistle associated with higher pollen protein content. Oecologia 190, 901–912. doi: 10.1007/s00442-019-04462-5
Sahinler, N., Gül, A., Akyol, E., Öksüz, A. (2009). Heavy Metals, trace elements and biochemical composition of different honey produce in Turkey. Asian J. Chem. 21, 1887–1896.
Sakač, M. B., Jovanov, P. T., Marić, A. Z., Pezo, L. L., Kevrešan, Ž.S., Novaković, A. R., et al. (2019). Physicochemical properties and mineral content of honey samples from Vojvodina (Republic of Serbia). Food Chem. 276, 15–21. doi: 10.1016/j.foodchem.2018.09.149
Sammataro, D., Erickson, E. H., Garment, M. B. (1985). Ultrastructure of the sunflower nectary. J. Apicultural Res. 24, 150–160. doi: 10.1080/00218839.1985.11100665
Sapir, Y. (2009). Effects of floral traits and plant genetic composition on pollinator behavior. Arthropod-Plant Interactions. 3, 115–129. doi: 10.1007/s11829-009-9062-y
Sari, E., Ayyildiz, N. (2012). Biological activities and some physicochemical properties of sunflower honeys collected from the thrace region of Turkey. Pakistan J. Biol. Sci. 15, 1102–1110. doi: 10.3923/pjbs.2012.1102.1110
Schmidt, L. S., Schmidt, J. O., Rao, H., Wang, W., Xu, L. (1995). Feeding preference and survival of young worker honey bees (Hymenoptera: Apidae) fed rape, sesame, and sunflower pollen. J. Economic Entomol. 88, 1591–1595. doi: 10.1093/jee/88.6.1591
Schulz, S., Arsene, C., Tauber, M., McNeil, J. N. (2000). Composition of lipids from sunflower pollen (Helianthus annuus). Phytochemistry 54, 325–336. doi: 10.1016/s0031-9422(00)00089-3
Scogin, R. (1978). Floral UV-absorption patterns and anthochlor pigments in the Asteraceae. Southwestern Naturalist. 23, 371–374. doi: 10.2307/3670243
Seiler, G., Jan, C. C. (2010). “Basic information,” in Genetics, Genomics and Breeding of Sunflower. Eds. Hu, J., Seiler, G., Kole, C. (CRC Press, Boca Raton), 1–50.
Shakya, R., Bhatla, S. C. (2010). A comparative analysis of the distribution and composition of lipidic constituents and associated enzymes in pollen and stigma of sunflower. Sexual Plant Reproduction. 23, 163–172. doi: 10.1007/s00497-009-0125-0
Sharma, B. (2019). An analyses of flavonoids present in the inflorescence of sunflower. Braz. J. Botany. 42, 421–429. doi: 10.1007/s40415-019-00552-z
Shivanna, K. R. (2014). “Biotic pollination: how plants achieve conflicting demands of attraction and restriction of potential pollinators,” in Reproductive Biology of Plants. Eds. Ramawat, K. G., Mérillon, J. M., Shivanna, K. R. (CRC Press, Boca Raton).
Shomali, A., Das, S., Arif, N., Sarraf, M., Zahra, N., Yadav, V., et al. (2022). Diverse physiological roles of flavonoids in plant environmental stress responses and tolerance. Plants 11, 3158. doi: 10.3390/plants11223158
Silva, F. A., Guirgis, A., von Aderkas, P., Borchers, C. H., Thornburg, R. (2020). LC-MS/MS based comparative proteomics of floral nectars reveal different mechanisms involved in floral defense of Nicotiana spp., Petunia hybrida and Datura stramonium. J. Proteomics 213, 103618. doi: 10.1016/j.jprot.2019.103618
Skinner, J. A. (1987). Abundance and spatial distribution of bees visiting male-sterile and male-fertile sunflower cultivars in California. Environ. Entomology 16, 922–927. doi: 10.1093/ee/16.4.922
Slavković, F., Bendahmane, A. (2023). Floral phytochemistry: impact of volatile organic compounds and nectar secondary metabolites on pollinator behavior and health. Chem. Biodiversity 20, e202201139. doi: 10.1002/cbdv.202201139
Soto, V. C., Gatica, I. J. G., Galmarini, C. R. (2021). Onion (Allium cepa L.) hybrid seed production: Sugar content variation during the flowering period. Crop Sci. 61, 3529–3537. doi: 10.1002/csc2.20597
Soto, V. C., Maldonado, I. B., Gil, R. A., Peralta, I. E., Silva, M. F., Galmarini, C. R. (2013). Nectar and flower traits of different onion male sterile lines related to pollination efficiency and seed yield of F1 hybrids. J. Economic Entomology 106, 1386–1394. doi: 10.1603/ec13096
Soto, V. C., Maldonado, I. B., Jofré, V. P., Galmarini, C. R., Silva, M. F. (2015). Direct analysis of nectar and floral volatile organic compounds in hybrid onions by HS-SPME/GC–MS: Relationship with pollination and seed production. Microchemical J. 122, 110–118. doi: 10.1016/j.microc.2015.04.017
Stanciu, O., Marghitas, L., Dezmirean, D., Campos, M. (2011). A comparison between the mineral content of flower and honeybee collected pollen of selected plant origin (Helianthus annuus L. and Salix sp.). Romanian Biotechnol. Letters. 16, 6291–6296.
Stejskalová, M., Konradyová, V., Suchanová, M., Kazda, J. (2018). Is pollinator visitation of Helianthus annuus (sunflower) influenced by cultivar or pesticide treatment? Crop Protection. 114, 83–89. doi: 10.1016/j.cropro.2018.08.018
Stephen, K. W., Chau, K. D., Rehan, S. M. (2024). Dietary foundations for pollinators: nutritional profiling of plants for bee health. Front. Sustain. Food Systems. 8. doi: 10.3389/fsufs.2024.1411410
Stpiczyńska, M., Płachno, B. J., Davies, K. L. (2018). Nectar and oleiferous trichomes as floral attractants in Bulbophyllum saltatorium Lindl. (Orchidaceae). Protoplasma 255, 565–574. doi: 10.1007/s00709-017-1170-4
Taha, E.−K.A. (2015). Chemical composition and amounts of mineral elements in honeybee-collected pollen in relation to botanical origin. J. Apicultural Sci. 59, 75–81. doi: 10.1515/jas-2015-0008
Taha, E.−K.A., Al-Kahtani, S., Taha, R. (2019). Protein content and amino acids composition of bee-pollens from major floral sources in Al-Ahsa, eastern Saudi Arabia. Saudi J. Biol. Sci. 26, 232–237. doi: 10.1016/j.sjbs.2017.06.003
Takatsuto, S., Omote, K. (1989). Phytosterol composition in the pollen of sunflower, Helianthus annuus L. Agric. Biol. Chem. 53, 3363–3364. doi: 10.1080/00021369.1989.10869818
Tantidanai-Sungayuth, N., Leewatchararongjaroen, J., Ritthiruangdej, P. (2012). Classification of thai honey origins by their mineral contents and color parameters. J. Agric. Sci. Technol. B. 2, 678–690.
Tasei, J.−N., Aupinel, P. (2008). Nutritive value of 15 single pollens and pollen mixes tested on larvae produced by bumblebee workers (Bombus terrestris, Hymenoptera: Apidae). Apidologie 39, 397–409. doi: 10.1051/apido:2008017
Tepedino, V. J., Parker, F. D. (1982). Interspecific differences in the relative importance of pollen and nectar to bee species foraging on sunflowers. Environ. Entomol. 11, 246–250. doi: 10.1093/ee/11.1.246
Terzić, S., Miklič, V., Čanak, P. (2017). Review of 40 years of research carried out in Serbia on sunflower pollination. OCL 24, D608. doi: 10.1051/ocl/2017049
Thakur, S. S., Rathore, R., Kanaura, S. (2005). Honey bee visit and nectar-sugar secretion pattern in sunflower Helianthus annuus L. as influenced by day hours, temperature and relative humidity. Indian J. Entomol. 67, 178–180.
Thiery, D., Bluet, J. M., Pham-Delègue, M.−H., Etiévant, P., Masson, C. (1990). Sunflower aroma detection by the honeybee. J. Chem. Ecol. 16, 701–711. doi: 10.1007/BF01016481
Thrasyvoulou, A., Tananaki, C., Goras, G., Karazafiris, E., Dimou, M., Liolios, V., et al. (2018). Legislation of honey criteria and standards. J. Apicultural Res. 57, 88–96. doi: 10.1080/00218839.2017.1411181
Todesco, M., Bercovich, N., Kim, A., Imerovski, I., Owens, G. L., Dorado Ruiz, Ó., et al. (2022). Genetic basis and dual adaptive role of floral pigmentation in sunflowers. eLife 11, e72072. doi: 10.7554/eLife.72072
Tomás-Barberán, F. A., Martos, I., Ferreres, F., Radovic, B. S., Anklam, E. (2001). HPLC flavonoid profiles as markers for the botanical origin of European unifloral honeys. J. Sci. Food Agric. 81, 485–496. doi: 10.1002/jsfa.836
Tourbez, C., Semay, I., Michel, A., Michez, D., Gerbaux, P., Gekière, A., et al. (2023). Heather pollen is not necessarily a healthy diet for bumble bees. Belgian J. Zoology 153, 105–124. doi: 10.26496/bjz.2023.111
Treanore, E. D., Vaudo, A. D., Grozinger, C. M., Fleischer, S. J. (2019). Examining the nutritional value and effects of different floral resources in pumpkin agroecosystems on Bombus impatiens worker physiology. Apidologie 50, 542–552. doi: 10.1007/s13592-019-00668-x
Truzzi, C., Illuminati, S., Annibaldi, A., Finale, C., Rossetti, M., Scarponi, G. (2014). Physicochemical properties of honey from marche, central Italy: classification of unifloral and multifloral honeys by multivariate analysis. Natural Product Commun. 9, 1595–1602. doi: 10.1177/1934578X1400901117
Tsuruda, J. M., Chakrabarti, P., Sagili, R. R. (2021). Honey bee nutrition. Veterinary Clinics North A. Food Anim. Practice. 37, 505–519. doi: 10.1016/j.cvfa.2021.06.006
Vanderplanck, M., Gilles, H., Nonclercq, D., Duez, P., Gerbaux, P. (2020). Asteraceae paradox: Chemical and mechanical protection of Taraxacum pollen. Insects 11, 304. doi: 10.3390/insects11050304
Vanderplanck, M., Marin, L., Michez, D., Gekière, A. (2023). Pollen as bee medicine: is prevention better than cure? Biology 12, 497–519. doi: 10.3390/biology12040497
Vanderplanck, M., Roger, N., Moerman, R., Ghisbain, G., Gérard, M., Popowski, D., et al. (2019). Bumble bee parasite prevalence but not genetic diversity impacted by the invasive plant Impatiens glandulifera. Ecosphere 10, e02804. doi: 10.1002/ecs2.2804
Vannette, R. L. (2020). The floral microbiome: Plant, Pollinator, and Microbial Perspectives. Annu. Rev. Ecology Evolution Systematics. 51, 363–386. doi: 10.1146/annurev-ecolsys-011720-013401
Vaudo, A. D., Dyer, L. A., Leonard, A. S. (2024). Pollen nutrition structures bee and plant community interactions. Proc. Natl. Acad. Sci. U. States A. 121, e2317228120. doi: 10.1073/pnas.2317228120
Vaudo, A. D., Patch, H. M., Mortensen, D. A., Tooker, J. F., Grozinger, C. M. (2016). Macronutrient ratios in pollen shape bumble bee (Bombus impatiens) foraging strategies and floral preferences. Proc. Natl. Acad. Sci. U. States A. 113, e4035–e4042. doi: 10.1073/pnas.1606101113
Vaudo, A. D., Tooker, J. F., Patch, H. M., Biddinger, D. J., Coccia, M., Crone, M. K., et al. (2020). Pollen protein: lipid macronutrient ratios may guide broad patterns of bee species floral preferences. Insects 11, 132–146. doi: 10.3390/insects11020132
Vear, F., Pham-Delègue, M., Tourvieille de Labrouhe, D. D., Marilleau, R., Loublier, Y., le Métayer, M., et al. (1990). Genetical studies of nectar and pollen production in sunflower. Agronomie 10, 219–231. doi: 10.1051/agro:19900305
Vîjan, L. E., Mazilu, I. C., Enache, C., Enache, S., Topală, C. M. (2023). Botanical origin influence on some honey physicochemical characteristics and antioxidant properties. Foods 12, 2134. doi: 10.3390/foods12112134
Villagómez, G. N., Brachvogel, R. C., Kárpáti, Z., Leonhardt, S. D., Schmitt, T., Ruedenauer, F. A. (2023). A common protocol for reliable comparison of pollen fatty acid profiles: highlighting pitfalls and proposing a methodology for ecological research. Front. Ecol. Evolution. 11. doi: 10.3389/fevo.2023.1141832
Westphal, C., Steffan-Dewenter, I., Tscharntke, T. (2003). Mass flowering crops enhance pollinator densities at a landscape scale. Ecol. Lett. 6, 961–965. doi: 10.1046/j.1461-0248.2003.00523.x
Westreich, L. R., Tobin, P. C. (2021). Comparison of pollen grain treatments without mechanical fracturation prior to protein quantification. J. Insect Sci. 21, 3. doi: 10.1093/jisesa/ieab043
White, J. W., Jr, Subers, M. H., Schepartz, A. I. (1963). The identification of inhibine, the antibacterial factor in honey, as hydrogen peroxide and its origin in a honey glucose-oxidase system. Biochim. Biophys. Acta 73, 57–70. doi: 10.1016/0926-6569(63)90108-1
Wright, G. A., Baker, D. D., Palmer, M. J., Stabler, D., Mustard, J. A., Power, E. F., et al. (2013). Caffeine in floral nectar enhances a pollinator’s memory of reward. Science 339, 1202–1204. doi: 10.1126/science.1228806
Wright, G. A., Schiestl, F. P. (2009). The evolution of floral scent: the influence of olfactory learning by insect pollinators on the honest signalling of floral rewards. Funct. Ecol. 23, 841–851. doi: 10.1111/j.1365-2435.2009.01627.x
Wright, E. K., Timberlake, T. P., Baude, M., Vaughan, I. P., Memmott, J. (2024). Quantifying the production of plant pollen at the farm scale. New Phytologist. 242, 2888–2899. doi: 10.1111/nph.19763
Yang, K., Wu, D., Ye, X., Liu, D., Chen, J., Sun, P. (2013). Characterization of chemical composition of bee pollen in China. J. Agric. Food Chem. 61, 708–718. doi: 10.1021/jf304056b
Yokota, S. C., Broeckling, C., H S Seshadri, A. (2024). Pollen foraging preferences in honey bees and the nutrient profiles of the pollen. Sci. Rep. 14, 15028. doi: 10.1038/s41598-024-65569-1
Yost, R. T., Fowler, A. E., Adler, L. S. (2023). Gut transplants from bees fed an antipathogenic pollen diet do not confer pathogen resistance to recipients. Microbial Ecol. 86, 3133–3137. doi: 10.1007/s00248-023-02309-4
Zajácz, E., Zaják, Á., Enikõ, S. M., Tamás, S. (2006). Nectar production of some sunflower hybrids. J. Apicultural Sci. 50, 109–113.
Zhang, X., Yu, M., Zhu, X., Liu, R., Lu, Q. (2022). Metabolomics reveals that phenolamides are the main chemical components contributing to the anti-tyrosinase activity of bee pollen. Food Chem. 389, 133071. doi: 10.1016/j.foodchem.2022.133071
Zhang, J., Wang, Z., Wen, P., Qu, Y., Tan, K., Nieh, J. C. (2018). The reluctant visitor: An alkaloid in toxic nectar can reduce olfactory learning and memory in Asian honey bees. J. Exp. Biol. 221 (17). doi: 10.1242/jeb.191478
Zhelyazkova, I., Lazarov, S. (2017). Comparative study of rapeseed, monofloral types and multifloral honey by some physicochemical parameters. Agric. Sci. Technol. 9, 277–281. doi: 10.15547/ast.2017.04.052
Zhou, J., Chen, L.−L., Zhu, Y.−T., Siemann, E., Wan, N.−F. (2023). Volatiles of the trap crop sunflowers: Effects on the behaviour of adult female Conogethes punctiferalis moths. J. Appl. Entomol. 147, 924–930. doi: 10.1111/jen.13185
Zhou, Z., Zhang, H., Mashilingi, S. K., Jie, C., Guo, B., Guo, Y., et al. (2024). Bombus terrestris prefer mixed-pollen diets for a better colony performance: A laboratory study. Insects 15, 285–297. doi: 10.3390/insects15040285
Keywords: floral rewards, Helianthus annuus, nectar, plant-pollinator interactions, pollen, pollination syndromes, pollinator health, pollinator nutrition
Citation: Husband S, Cankar K, Catrice O, Chabert S and Erler S (2025) A guide to sunflowers: floral resource nutrition for bee health and key pollination syndromes. Front. Plant Sci. 16:1552335. doi: 10.3389/fpls.2025.1552335
Received: 27 December 2024; Accepted: 26 March 2025;
Published: 01 May 2025.
Edited by:
Ujjwal Layek, Rampurhat College, IndiaReviewed by:
Domancar Orona-Tamayo, Centro de Innovación Aplicada en Tecnologías Competitivas (CIATEC), MexicoArijit Kundu, Vidyasagar University, India
Copyright © 2025 Husband, Cankar, Catrice, Chabert and Erler. This is an open-access article distributed under the terms of the Creative Commons Attribution License (CC BY). The use, distribution or reproduction in other forums is permitted, provided the original author(s) and the copyright owner(s) are credited and that the original publication in this journal is cited, in accordance with accepted academic practice. No use, distribution or reproduction is permitted which does not comply with these terms.
*Correspondence: Salena Husband, aHVzYmFuZC5zYWxlbmFAZ21haWwuY29t