- 1College of Horticulture Science, Zhejiang A&F University, Hangzhou, Zhejiang, China
- 2Key Laboratory for Agricultural Soil and Water Engineering in Arid Area of Ministry of Education, Northwest A&F University, Yangling, Shaanxi, China
- 3Key Laboratory of Quality and safety Control for Subtropical Fruit and vegetable, Ministry of Agriculture and Rural Affairs, Hangzhou, Zhejiang, China
- 4State Key Laboratory of Soil Erosion and Dryland Farming on the Loess Plateau, Institute of Water and Soil Conservation, Northwest A&F University, Yangling, Shaanxi, China
Water shortages greatly challenge high-quality apple production in dryland agricultural regions. Bridging the gap between water use and apple crop water requirements, as well as clarifying water suitability levels, are essential steps to improve water use efficiency. This study innovatively introduced phenological models to accurately predict apples’ phenological stages, thus constructing a dynamic crop coefficient (Kc) curve. By skillfully integrating this curve with classic FAO 56 Penman-Monteith (P-M) ETo model, the water requirements (WR) and water suitability (S) were evaluated during apple flowering-fruit setting, fruit expansion, and coloring-maturity stages. The results showed that the average durations of the apple phenological stages were 22 days for flowering-fruit setting, 102 days for fruit expansion, and 39 days for fruit coloring-maturity. Unexpectedly, counterintuitive results emerged regarding water requirements and suitability across the phenophases. Despite the fruit expansion stage having the highest average water requirement (319 mm), multi-year data indicated ‘relatively suitable’ (S=0.8) conditions for most counties. In contrast, although the average water requirement during flowering-fruit setting was 120 mm, the suitability level was classified as ‘unsuitable’ (S=0.3), indicating a water shortage, particularly in Xinjiang, the northwest Loess Plateau, and northern Bohai Bay areas. The coloring-maturation stage, with an average water requirement of 113 mm, was classified as ‘very suitable’ (S=1.5), reflecting highly favorable conditions. As this stage progressed, over-humidity conditions began in the Southwestern Cool Highlands and spread to the southwestern Loess Plateau. These findings revealed that the relationship between water requirements and suitability was not linear and emphasized the critical need for focused water management during the flowering-fruit setting stage to ensure sustainable apple production.
1 Introduction
Apple trees, as perennial crops with high water requirements, consumed substantial amounts of water to produce high-quality fruit during various phenological stages (Wang and Liu, 2022). China was one of the world’s largest apple producers, with the highest output and an extensive production area. Most high-quality apples with commercial value were grown in dryland agricultural regions, such as the Yellow River Basin, Haihe River Basin, and Aksu River Basin (Sugiura et al., 2023; Vujadinović Mandić et al., 2023). Irrigation systems built near these river basins ensured the sustainable development of local fruit production (Wang et al., 2020a). However, the accelerating impacts of climate change increasingly highlighted the instability of irrigation water resources for orchards in these areas. This raised serious concerns for apple production and highlighted the need to optimize water resource management strategies to mitigate the potential crisis and ensure the stable growth of apple cultivation.
Recently, methods needed to improve management of actual water use by crops in irrigated agriculture have been developed providing useful insights on orchard water use patterns. Among them, soil-based methods, which use soil moisture sensors to monitor water conditions in the root zone and surrounding areas, and plant-based methods, which rely on plants as biosensors to integrate soil and climatic conditions along with physiological responses to water deficits, are widely used (Juillion et al., 2022; Wang et al., 2020b). Despite their usefulness, studies conducted using these methods in fields and small catchments have generally failed to provide comprehensive spatial water characteristics of soil and orchards at a regional scale, limiting their applicability for large-scale water management (Dzikiti et al., 2018). Moreover, most existing studies tend to focus only on a specific growth stage of the orchard (Jia et al., 2020; Wang et al., 2021a; Zanotelli et al., 2019), which leaves a significant gap in detailed, continuous, quantitative data regarding how water requirements fluctuate from flowering to fruit maturity.
In addition to these methods, the concept of water suitability has become increasingly important in agricultural water management and has proven effective in guiding water management planning across extensive crop areas. The level of water suitability measures how closely local effective rainfall aligns with the ideal water conditions required for crop growth. For instance, Elnashar et al. (2021) assessed the water suitability of pome fruits using the ALES-Arid, Ref-ET, and SEBAL models, while Fadl and Abuzaid (2017) calculated the water requirements of various crops, including peach, citrus, olive, and sunflower using the FAO-Cropwat model. These researches demonstrated that the concept of water suitability was widely applicable to various crops. Similarly, Gao et al. (2018) quantified apple water suitability in the Loess Plateau of China using the Penman-Monteith equation, revealing important trends in regional water availability for apple production. However, while water suitability index has been effectively applied, there remains a research gap in quantifying water suitability for fruit trees, particularly apples, across multiple phenological stages. Therefore, comprehensive studies are needed to better understand how water suitability evolves during the different phenological stages of apples, from flowering to fruit maturation.
Water requirements calculation was the core foundation of water suitability. The most widely used estimate of water requirements for a particular crop is obtained multiplying the reference evapotranspiration (ETo, the sum of evaporation and transpiration of homogeneously clipped and well-irrigated grass field (Allen et al., 1998; Monteith, 1965; Penman, 1948), calculated by the FAO 56 Penman-Monteith (P-M) ETo equation, by a crop coefficient (Kc), yielding ETc=ETo×Kc. ETo is determined based on meteorological data from reference stations. However, Kc represents an integration of the primary characteristics that differentiate the grass reference from the crop regarding energy balance (Allen et al., 1998). Therefore, the Kc value must be adjusted for specific crops, tree spacing, regional climates, and canopy coverage at different growth stages (Doorenbos et al., 1977; Panigrahi, 2023; Ye et al., 2020). In practice, there are two primary approaches to obtain Kc values: one relies on field observations, while the other predicts Kc values based on observed surface cover conditions and height ratios (Dang et al., 2020; Hublart et al., 2016). Both methods presented significant challenges for woody crops like fruit trees or crops with incomplete canopy cover. The unique growth habits and complex canopy structure of fruit trees made field observations difficult, while prediction methods based on ground cover could not capture the full growth details, leading to significant data discrepancies (Liu et al., 2023). To address these challenges, the application of phenological models became crucial. Phenological models could simulate the dynamic growth of apple trees across different phenological stages, helping to understand their changes over time (Fernandez et al., 2022; Yaacoubi et al., 2019). By using these models, more accurate Kc-time curves could be constructed, overcoming the limitations of traditional methods and providing an effective tool for optimizing water management strategies in apple orchards and improving the precision of Kc estimation.
The objectives of this study are to estimate the water requirement of apple trees at the county scale in China’s main apple-producing areas, and to assess water suitability levels during apple three phenological stages. Specifically, the study focused on: (1) Quantifying phenological stages: The time window of three key phenological stages of apple development—flowering-fruit setting, fruit expansion, and fruit coloring-maturity—were identified and quantified using an ensemble of multiple phenological models; (2) Estimating water requirements: the FAO 56 Penman-Monteith (P-M) ETo equations, combined with crop coefficients (Kc) specific to different phenological stages, were applied to estimate potential evapotranspiration (ETo) and subsequently calculate the water requirements for apple trees; (3) Introducing the index of water suitability level: The water suitability level for apples was quantified by considering the spatiotemporal evolution characteristics of effective precipitation during the three phenological stages. The study provided a scientific basis for the irrigation planning and management of apple orchards.
2 Materials and methods
2.1 Study area
China’s apple cultivation covers vast regions and can be divided into four main apple-producing areas (Figure 1): the Loess Plateau region, the Bohai Bay region, the Southwest Cool Highland region, and the Xinjiang region (Zhang et al., 2020). The sum of apple planting area in the Loess Plateau and Bohai Bay regions amounts to 1.63 million hectares, yielding a total apple production of 37.3 million tons, which constitutes 82.4% and 87.9% of China’s total apple orchard area and production, respectively Wang et al., 2019). The total apple production of Southwest Cool Highland approximately 2 million tons in 2021 (Lijia and Xuexi, 2014). “Fuji” is dominant apple variety, with planting area and total production exceeding 50% and 76.4%, respectively (Lu et al., 2022).
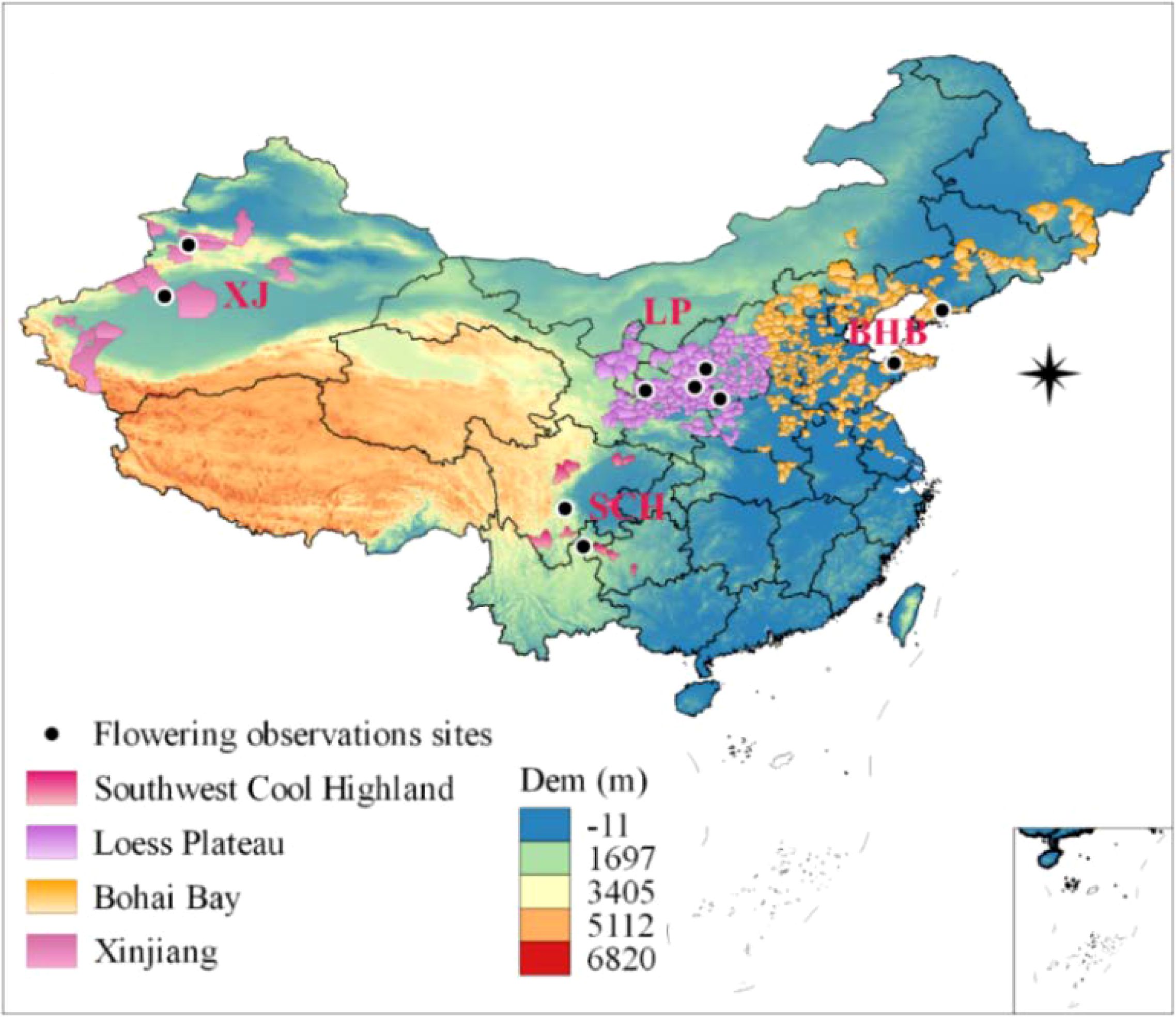
Figure 1. Map of four main apple cultivation regions in China’s: the Loess Plateau region (LP), the Bohai Bay region (BHB), the Southwest Cool Highland region (SCH), and the Xinjiang region (XJ). The black dots are the location information of the apple flowering dates observation sites.
2.2 Data sources
2.2.1 Meteorological data
The meteorological data, which comprise two weather datasets, were collected from China Meteorological Data Sharing Network (http://data.cma.cn). This first dataset includes daily measured Rs and other accessible weather variable measurements from 80 solar radiation observation stations in China (Supplementary Table S1). The second dataset contains both benchmark and basic meteorological stations across 367 primary apple-growing counties from 1960 to 2020. The accessible weather variables in the two datasets include maximum temperature (Tmax, °C), minimum temperature (Tmin, °C), precipitation (P, mm), wind speed (U, m s − 1), relative humidity (RH, %), and sunshine hours (n, h). In addition, the high costs of establishment and maintenance of solar radiation observation equipment have resulted in a limited number of solar radiation monitoring stations in China, restricting the application of the Penman-Monteith model. To address this limitation, the previous research performed quality control on meteorological data and utilized machine learning combined with the Ångstrom-Prescott equation to estimate solar radiation at 839 meteorological stations based on the solar radiation observation (Chen et al., 2022; He et al., 2020).
2.2.2 Phenological data
The observation data of apple flower dates were obtained from the Shaanxi Meteorological Bureau (http://sn.cma.gov.cn/) and the Nanjing Shuxi Intelligent Technology Co., Ltd. (http://www.shuxitech.com/). The dataset for flowering dates spanned from 1972 to 2020, encompassing a total of 10 observation counties distributed across the four major apple-producing areas in China. Detailed latitude and longitude information of these flowering observations for each site is provided in Figure 1 (represented by black dots) and Table 1.
2.3 Identifying and modeling apple phenology
2.3.1 Identifying and dividing phenology stage of apples
The critical phenological stages that determined yield and fruit quality in apple trees occurred between flowering and maturity (Han et al., 2023; Yang et al., 2021). We divided this period into three phenological windows: the flowering-fruit setting stage, the fruit expansion stage, and the fruit coloring-maturity stage. Descriptive diagrams of these specific phenological stages of apples and the typical observed occurrence times of phenology are presented in Table 2. The three phenological stages of apples mapped to the FAO56 growth stages (initial, mid-season, and late-season). We accurately simulate site-specific flowering dates and subsequently determined the duration from the initial occurrence to the end of these three phenological stages. For instance, we simulated that the flowering date of a particular site is April 3 (93 DOY), and the fruit-setting date is May 3 (123 DOY), indicating a 30-day flowering-fruit setting stage. This practice provided an appropriate framework to construct Kc curves on a daily scale.
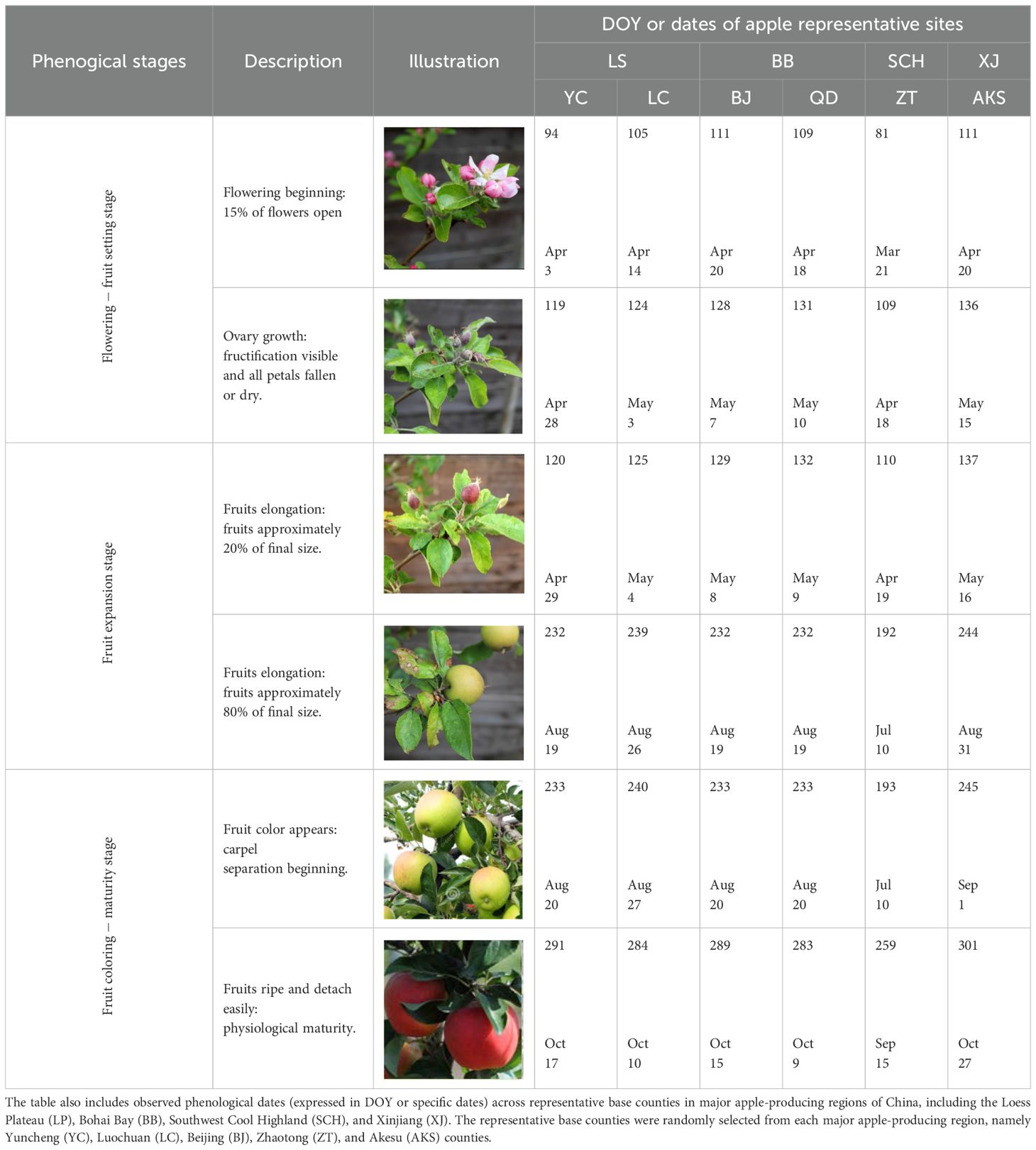
Table 2. Description and illustration of the three time windows of apple growth stages—flowering-fruit setting (Initial Stage in FAO56), fruit expansion (Mid-Season Stage in FAO56), and fruit coloring-maturity (Late-Season Stage in FAO56).
2.3.2 Apple crop coefficient (Kc)
The crop coefficient (Kc) was a dynamic parameter that reflected variations in crop water demand across different phenological stages. Long-term site monitoring in major apple-producing regions of China, including Yan’an (Shaanxi) (Meng, 2011), Linfen (Shanxi) (Dang et al., 2020; Wang et al., 2021a), and the hilly areas of Shandong (Chen, 2011; Liu et al., 2024), revealed characteristic changes in Kc for apple trees. Specifically, Kc increased from 0.33 to 0.45 during the early phenological stage, rose further to 0.95 during the flowering and fruiting stage, remained stable during the peak stage of fruit expansion, gradually decreased during the coloring-maturity stage, and eventually fell back to 0.33 during the leaf fall and dormancy stage (Figure 2). This dynamic pattern corresponded closely to the water requirements of each phenological stage.
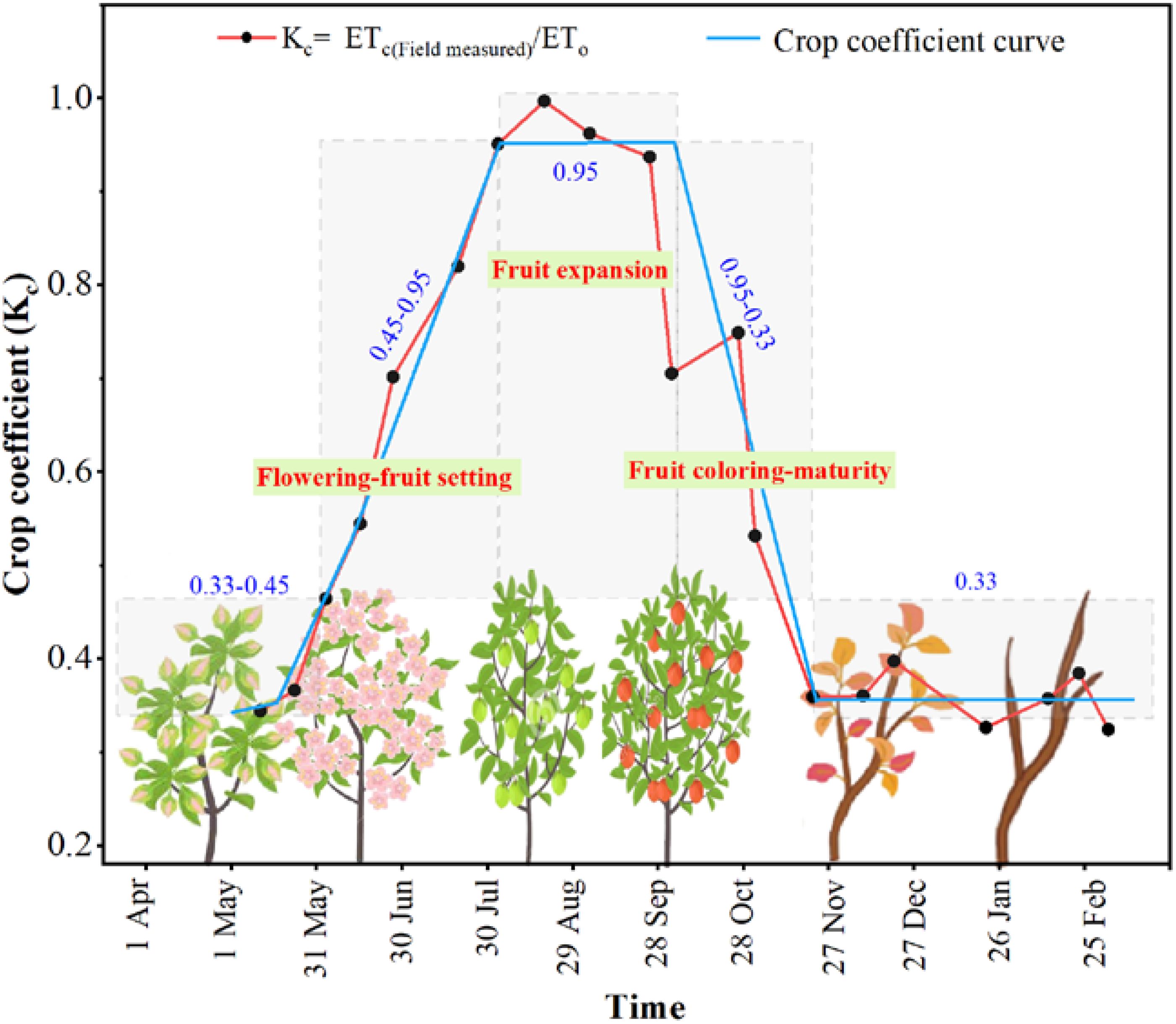
Figure 2. Corrected crop coefficient (Kc) curves during different phenological stages in the representative apple orchard of China.
In this study, key phenological stages of apple trees—flowering-fruit setting, fruit expansion, and fruit coloring-maturity—were simulated and integrated with the dynamic Kc curve. The FAO-56 Penman-Monteith formula was then employed to estimate crop evapotranspiration (ETc) for each stage. For instance, Kc started at 0.75 during the flowering-fruit setting stage, increased gradually to 0.95 during the fruit expansion stage, remained at 0.95 throughout fruit coloring-maturity stage. These Kc values, derived from field measurements under local climatic conditions and apple-specific growth patterns, were well-suited for estimating water requirements.
2.3.3 Apple phenology model
The apple phenological model is based on the temperature response theory of ‘heat forcing’ and ‘chilling requirement’ (Chuine et al., 1999; Ettinger et al., 2020). ‘Heat forcing’ describes the accumulation of heat that stimulates apple trees after dormancy. Once enough heat is accumulated, the apple trees begin flowering. Common forcing response functions include linear, sigmoidal (S-shaped), and exponential functions (Harrington and Gould, 2015). ‘Chilling requirement’ is another important factor in the dormancy release of apple trees. Moderate sub-zero temperatures induce dormancy, which is typically broken after prolonged exposure to chilling but non-freezing temperatures between 2 and 7°C (Basler, 2016; Hänninen, 1987; Luedeling et al., 2009). In current models, the response to chilling temperatures is implemented either as a triangular function of temperature or bell shaped curve (Wang et al., 2020b).
This study used a weighted ensemble model of four phenological models to simulate the flowering date of apple. These four models are the Photothermal-time model (M1) (Blümel and Chmielewski, 2012), the Uniforc model (Chuine, 2000), the Alternating model (Benmoussa et al., 2018; Chuine, 2000), and the Unichill model (Table 3) (Caffarra et al., 2011). The first two models solely account for ‘heat forcing’, whereas the latter two models integrate sub-models of ‘chilling requirements’ and ‘heat forcing’ in different manners (Harrington and Gould, 2015; Ru et al., 2023a). Different assumptions about the functional form of the temperature effects when the model was originally built resulted in diverse models. For more information and detailed descriptions of the above phenological model, please refer to Section 2.3.3 in the Supporting Materials.
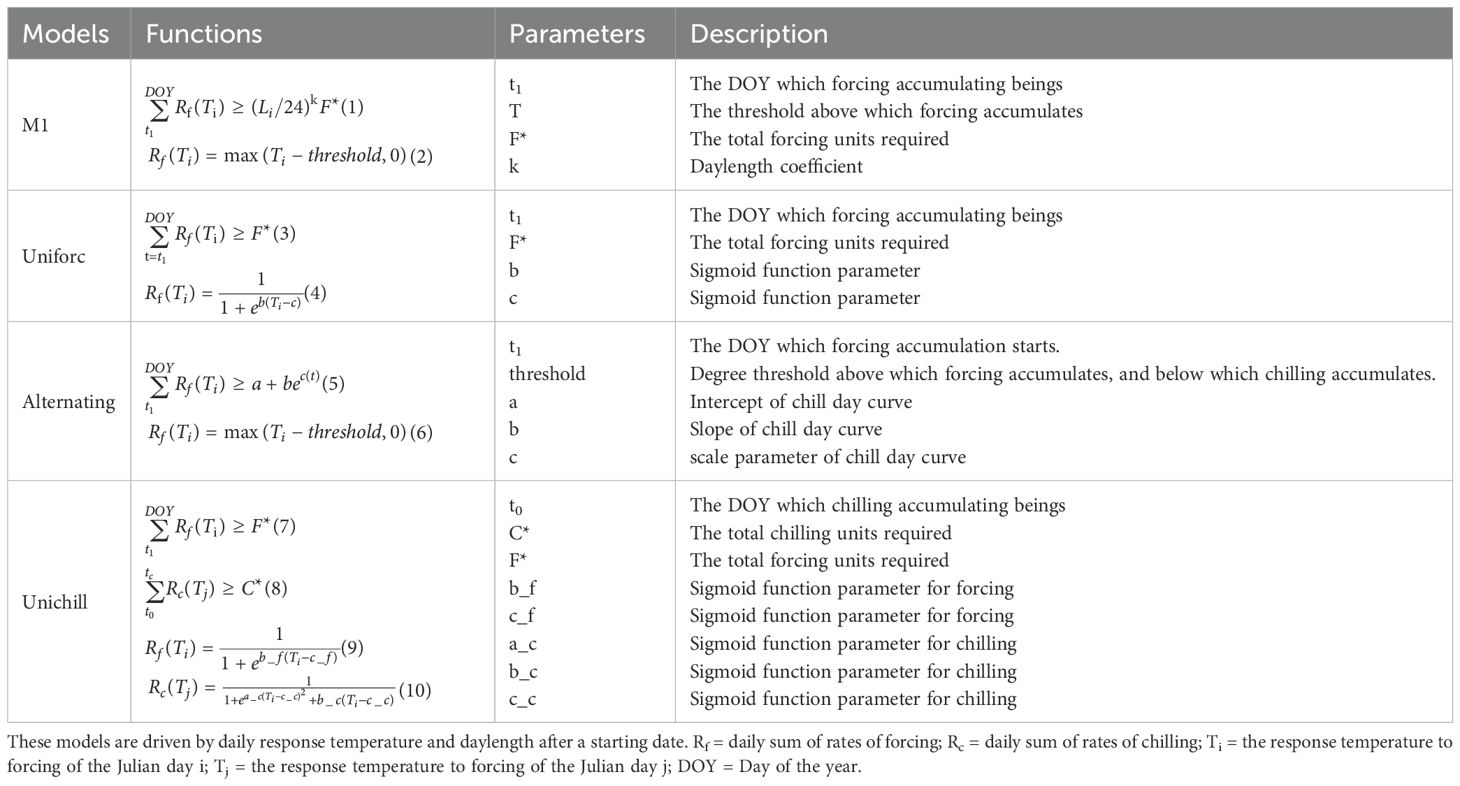
Table 3. Temperature response functions and structures of chilling/forcing-based apple phenology models used in this study.
The core weighted model was an overall model that combined the predictions of several phenological models. The variance of the output value of this weighted model is lower and better than any member of the model. The weight (wm) of this core model is determined by the Root Mean Square Error (RMSE) values for each participating models (m) in model validation. If mRMSE is the RMSE in the phenological model validation of each participating model, . Therefore, the final simulated apple flowering dates were actually weighted ensembles of four different models.
2.3.4 Model calibration and performance evaluation
The phenological model was subjected to rigorous calibration and validation procedures before application. Initially, the basin hopping algorithm was employed to estimate the parameter values of the phenology model (Wales and Doye, 1997). This calibration process minimized the RMSE (Equations 11) between each model’s simulated and observed apple phenology dates. To achieve the most accurate model parameters, the basin hopping algorithm was configured to run for 1000 iterations, accompanied by a random perturbation value of 0.5. The calibration process was iterated until parameters yielding the final selected minimum RMSE value were obtained (Wang et al., 2021b). Subsequently, the remaining 30% of phenological observations were used to evaluate the robustness of the apple phenology simulation model during the testing process. Additionally, we computed the overall adjusted R square (R2, Equations 12) to evaluate the goodness-of-fit and prediction error measures during both the calibration and validation datasets.
where Oi represented observed apple phenology dates (DOY); represented the average observed phenology dates (DOY); Pi represented simulated phenology dates at site i; n was the number of observations.
2.4 Quantifying apple water suitability level using the FAO 56 Penman-Monteith ETo equation
2.4.1 Estimating water requirements for apples
Apple water requirement (WR) refers to the total amount of water required to compensate for evapotranspiration losses from farmland under well-managed conditions, such as the absence of water, fertilizer, or insect stress (Doorenbos et al., 1977; Villalobos et al., 2016). The specific water requirement of apples is calculated by multiplying the specific coefficient parameters of apple crops at different growth stages and the reference evapotranspiration (Equation 13). The formula is as follows:
Where WR is apple water requirement (mm); j is the key phenological stage of apple (flowering and fruit setting period, fruit enlargement period and coloring and maturity period); n is the number of days included in the jth growth period of apple, and i is the day ordinal number. Kc is the crop coefficient in the jth growth period, which represents the comprehensive influence coefficient of various impedances (such as surface impedance, stomatal impedance, and diffusion impedance). ETo is the reference crop evapotranspiration (mm/d) (Equation 14). The formula based on the FAO 56 Penman-Monteith (P-M) ETo (Allen et al., 1998; Villalobos et al., 2016) estimation formula is as follows:
Where ETo represents the reference crop evapotranspiration (mm/day); Rn denotes the net radiation input within the canopy [MJ/(m2 day)]; G stands for the soil heat flux [MJ/(m2 day)]; T represents the daily average temperature at a high altitude of 2 meters (°C); u2 signifies the wind speed at a height of 2 meters (m/s), derived from the wind speed value u10 measured at 10.5 meters by the weather station, following the unified standard, where u2 = 0.743 u10; es represents the saturated water vapor pressure (kPa); ea represents the actual water vapor pressure (kPa); Δ denotes the slope of the curve representing the relationship between the difference in saturated water vapor pressure and temperature at a specific point (kPa/°C); γ symbolizes the constant relating to wet and dry thermometers (kPa/°C). For clarity, all the above parameters, parameter symbols, and calculation methods involved in the ETo formula are presented in Table 4.
2.4.2 Determining irrigation water requirements for apples
The apple irrigation water requirement (IWR) refers to the volume of water essential to satisfy the physiological water requirement (WR) of apple trees, in addition to the precipitation received (Equation 15). The calculation formula is outlined below:
Where IWR represents the irrigation water requirement of apples (mm), WR denotes the water requirement of apples (mm), Peff signifies the effective precipitation (mm), and j corresponds to the critical phenological stage of apples (including flowering-fruit setting, fruit expansion, and coloring-maturity stage).
Given that not all precipitation (P) received in the field is available for crop utilization, this study incorporates the concept of effective precipitation (Peff). Effective precipitation refers to the portion of precipitation that actively contributes to soil conservation and plant growth under conditions conducive to meeting the water demands of plants. In the realms of irrigation and agriculture, understanding effective precipitation is pivotal for accurately computing irrigation water needs and judiciously managing water resources in agricultural land. Peff represents the fraction of total precipitation (P) that can directly benefit crops without loss. The variability in Peff is influenced by several factors, including precipitation characteristics, soil parameters, crop evapotranspiration rates, and irrigation practices. In this study, the United States Department of Agriculture (USDA) soil conservation method was employed (Bal et al., 2022), and Peff was estimated using the following formula (Equation 16). Detailed analytical data and results on Peff in the study area are provided in the Supporting Material (Supplementary Figure S2).
2.4.3 Assessing levels of water suitability for apples
The water suitability level (S) for apples is quantified by the extent to which the cumulative effective precipitation during the apple’s growth period fulfills its physiological water needs (Equation 17). This relationship is captured by the formula:
Where Sj represents the water suitability level of apples in the jth growth period, Peff represents the cumulative effective precipitation during apple corresponding phenological phases (mm), and WRj represents the physiological water demand of apples during the corresponding phenological phases (mm);
This study classified six water suitability levels for apples (Equation 18) according to the studies on water suitability level indicators (Oiu et al., 2018). Each level corresponds to a specific range of water suitability, indicating how well the apple trees’ water requirements are being met.
3 Results
3.1 Performance of models and phenology time derived from simulation
The performances of four phenological models in predicting apple initial flowering dates were evaluated using the R2 and RMSE values in the model validation process (or RMSEv), as depicted in Figure 3. Among the predicted apple flowering dates of the other three models, the Unichill model exhibited the largest simulation error (R2 = 0.33, RMSEv = 9.71 d), while the Alternating model demonstrated the best simulation accuracy (R2 = 0.63, RMSEv = 5.21 d). The M1 model yielded a slightly larger RMSEv value of 6.97 d (R2 = 0.59), and the Uniforc model had an RMSEv value of 6.88 d (R2 = 0.58). Based on these values obtained from the model validation process, it can be concluded that the performances of the four phenological models in predicting apple flowering dates were generally acceptable for simulation studies.
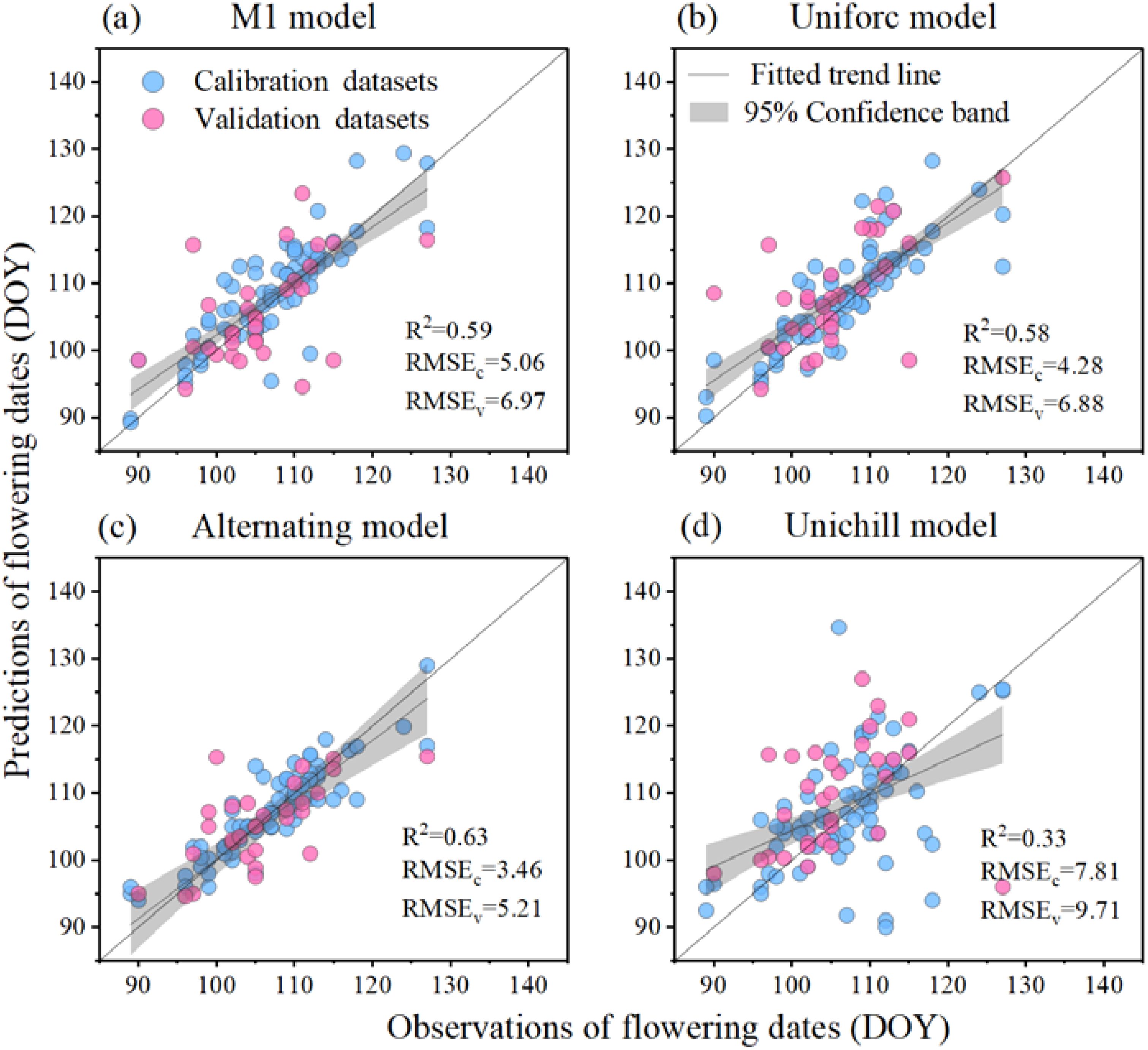
Figure 3. Root-mean-square error (RMSE) and R2 between apple flowering observations and predictions in model calibration (RMSEc) and validation (RMSEv). The blue circles represented the calibration dataset, the pink circles represented the validation dataset, the gray line indicated the linear trend line, and the gray shaded area denoted the 95% confidence band.
After modeling the flowering dates of various regions, we computed the time window from initial flowering to fruit set, initial fruit expansion to final fruit expansion, and fruit coloring to maturity stages, respectively (Figure 4). It is important to note that our study encompassed over 300 apple-producing counties across China. To present the quantitative findings effectively, we randomly selected the results from 100 sites for display. The average length of apple flowering-fruit setting, fruit expansion, and fruit coloring-maturity stages was 22, 102, and 39 days, respectively. The three phenological stages of apples differ across different regions, which displayed the advantage of individually calculating different phenological stages of apples based on modeling methods. This approach highlighted the importance of using phenological models to simulate the flowering dates when considering the external climate conditions.
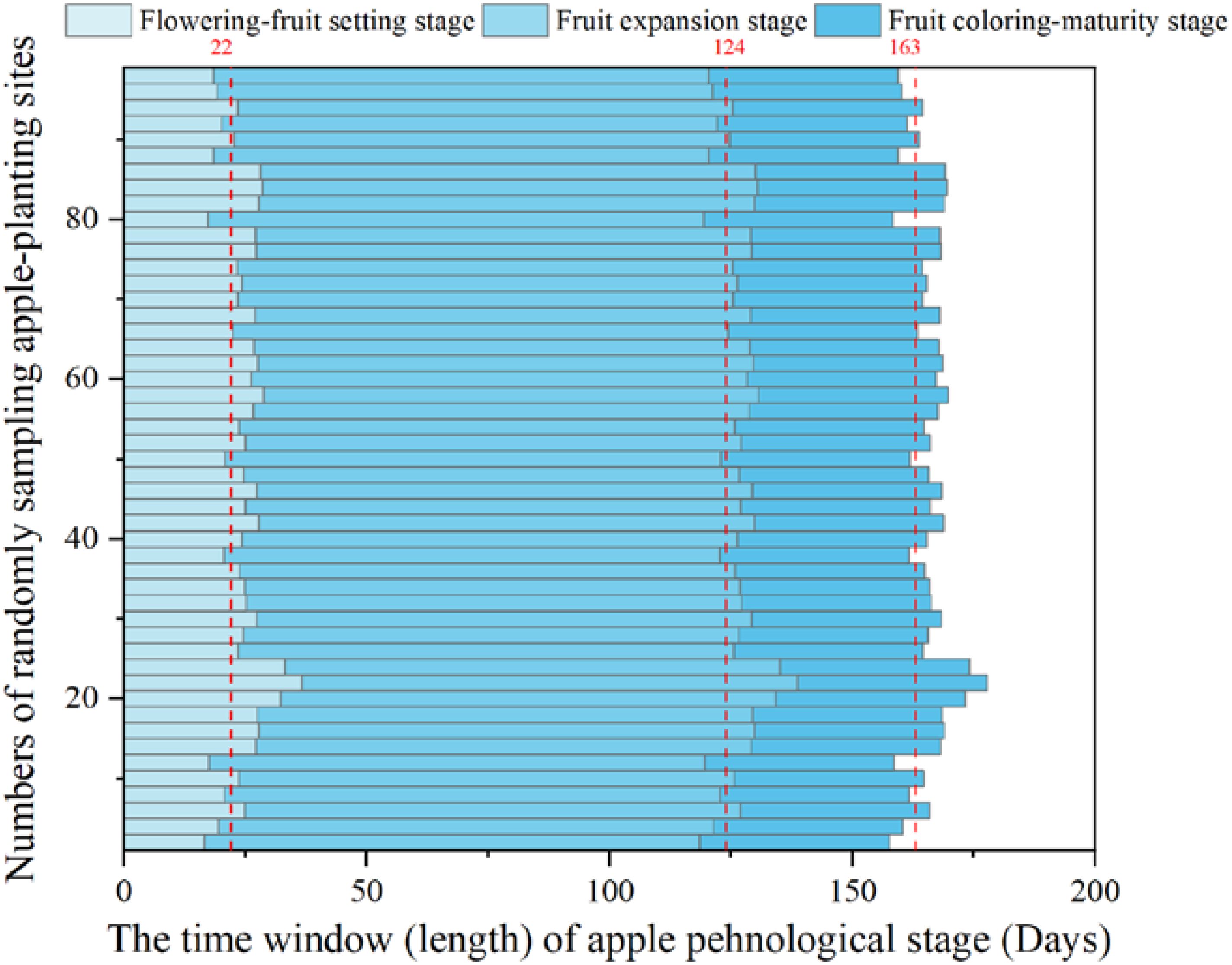
Figure 4. The time window for the apple flowering-fruit setting, fruit expansion, and fruit coloring-maturity stages. These phenological time was quantified based on the simulated apple flowering dates obtained from the calibrated phenological model mentioned above. The red dotted line is the average line.
3.2 Spatiotemporal changing trends of apple water requirements
Assessing the spatial and temporal variation in water requirements for apples is crucial for effective water management and sustainable apple production practices. The multi-year average water requirements (WR) of apples were 120 mm, 319 mm, and 113 mm during the flowering-fruit setting stage, fruit expansion stage, and coloring-maturity stage, respectively (Figure 5). The results showed that the WR was highest during the fruit expansion stage, followed by the flowering-fruit setting stage and the coloring-maturity stage. This trend suggested that more water was needed during the stages of active growth and fruit development, which aligned with the physiological demands of the apple tree. The minimum and maximum WR were approximately 67 mm and 160 mm during the flowering-fruit setting stage, respectively. During the fruit expansion stage, the minimum and maximum WR were approximately 191 mm and 559 mm, respectively, and approximately 62 mm and 167 mm during apple coloring-maturation stage. The variability in the range of WR values for each stage indicated the varying water needs within each apple phenological stage, which highlighted the importance of effectively understanding and managing water consumption throughout the apple growing season.
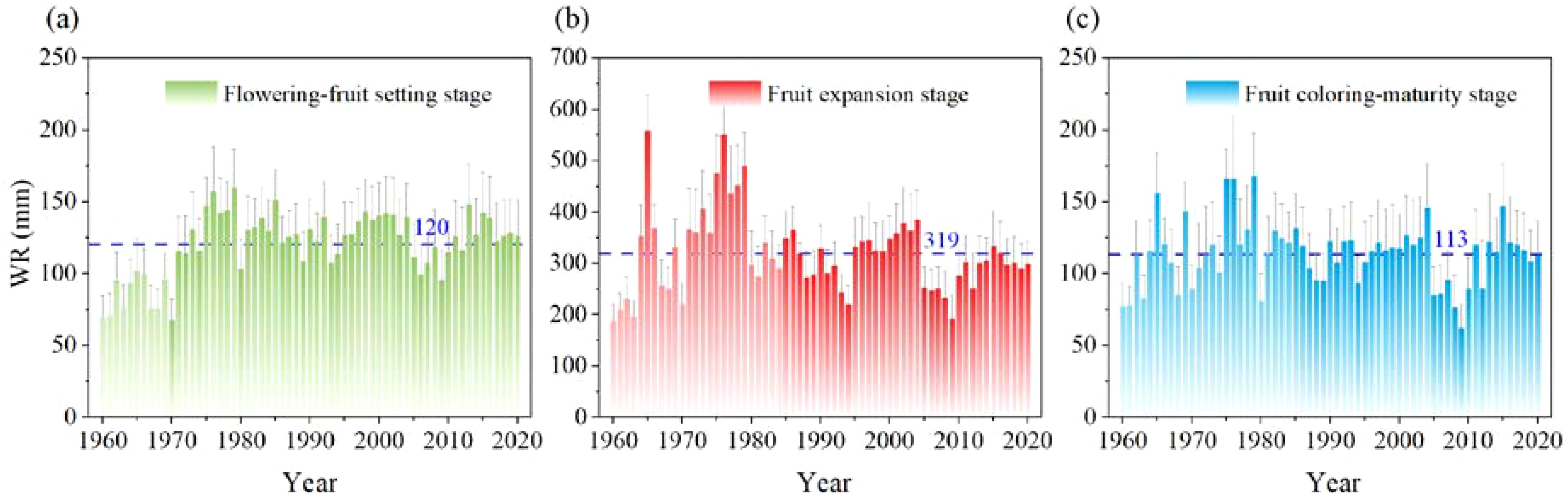
Figure 5. Temporal variation of water requirement (WR) in apple flowering-fruit setting stage (a), fruit expansion stage (b), and fruit coloring-maturity stage (c) from 1960 to 2020 in the whole apple-producing areas of China.
The changes of WR were analyzed through the spatial distribution map and the frequency distribution histogram during apple flowering-fruit-setting, fruit expansion and coloring-maturity stage (Figure 6). The average WR of apples were 114 mm, 290 mm, and 109 mm during the flowering-fruit setting stage, fruit expansion stage, and coloring-maturity stage across all apple production counties, respectively. Some apple production areas exhibited relatively high water requirements (WR > 136 mm) during the flowering-fruit setting stage. These areas included the northwestern parts of the Loess Plateau (e.g., Ningxia, Shaanxi, and northern Shanxi province), the central Bohai Bay areas, and the southern parts of Xinjiang. Conversely, areas with relatively low water requirements (WR< 83 mm) were found in eastern parts of Gansu, Henan, and Heilongjiang province during apples flowering-fruit setting stage. The frequency diagram displayed the normal distribution trend of apple WR during the three phenological stages. The WR of 101 to 120 mm was at the maximum frequency during the flowering-fruit setting stage (Figure 6a). During the fruit expansion stage, the WR of the apple notably surpassed that of the flowering-fruit setting stage. Areas with higher water requirements were identified in Xinjiang, Ningxia, Shaanxi, and Shanxi provinces (Figure 6b). The WR in the range of 250 to 301 mm was at the maximum frequency during apple fruit expansion stage. In contrast, the WR was slightly lower during the coloring-maturation stage, with the range of 101 to 136 mm of WR showing the maximum frequency compared to the fruit expansion stage (Figure 6c).
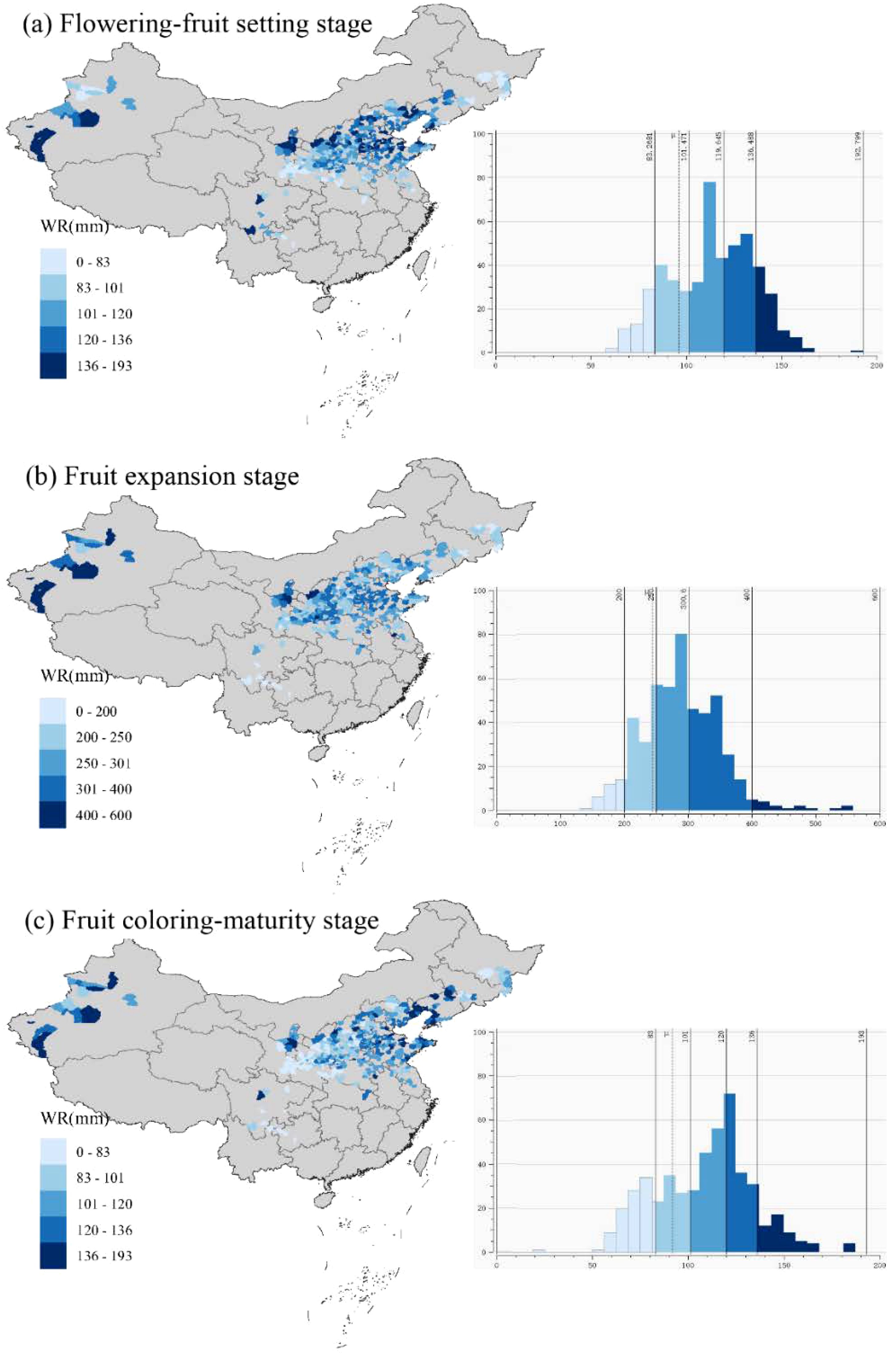
Figure 6. Spatial distribution map and frequency histogram of water requirement (WR) during apple flowering to fruit-setting stage (a), fruit expansion stage (b), and fruit coloring maturity stage (c) in the whole apple-producing areas of China.
3.3 Spatiotemporal changing trends of apple irrigation water requirements
The irrigation water requirements (IWR) were accessed during three phenological stages across apple-producing counties in China (Figure 7). The average IWR of apples can be ranked as follows: fruit expansion stage (145 mm) > flowering-fruit setting stage (91 mm) > coloring-maturity stage (58 mm). The IWR during the flowering–fruit setting stage ranged from 16 mm to 148 mm, with a standard deviation of approximately ±34 mm. This indicated moderate interannual variability and suggested that a fixed irrigation amount could generally meet the needs during this stage. In contrast, the IWR during the fruit expansion stage showed the greatest variability, ranging from 4 mm to 524 mm, with a standard deviation of ±41mm. This reflected a high sensitivity to climatic fluctuations and emphasized the importance of dynamic irrigation scheduling. During the coloring–maturity stage, the IWR ranged from 3 mm to 196 mm, with relatively lower overall water demand but still observable interannual variation.
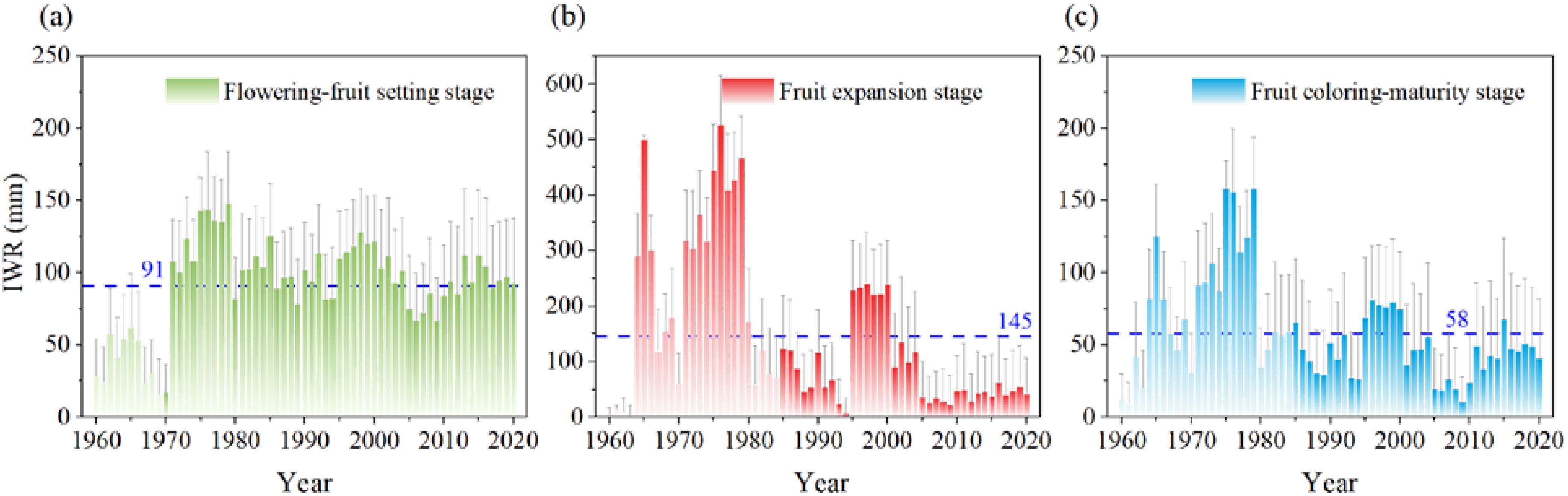
Figure 7. Temporal variation of irrigation water requirement (IWR) in apple flowering-fruit setting stage (a), fruit expansion stage (b), and fruit coloring-maturity stage (c) from 1960 to 2020 in the whole apple-producing areas of China (The blue dotted line is the average IWR line).
The spatial distribution map and the frequency distribution histogram of apples’ IWR were shown during three phenological stages (Figure 8). As latitude increases, the IWR also increases during the flowering-fruit-setting stage. In the northern high latitudes (e.g., Xinjiang production areas), the IWR was the highest. This phenomenon could be attributed to China’s precipitation pattern, where it rains more in the southeast and less in the northwest. Regionally, the Bohai Bay apple production area exhibited high average IWR during the apple flowering-fruit setting stages, while Xinjiang and the Loess Plateau (e.g., Gansu, Ningxia, and Shaanxi) production areas showed high IWR during the fruit expansion stages and the coloring-maturation stage. Additionally, the frequency histogram revealed that the IWR was most concentrated between 60 and 81 mm during the flowering-fruit setting in apple-producing counties across the country, 0 and 24 mm during the fruit expansion stage, and 14 and 38 mm during the coloring-maturity stage.
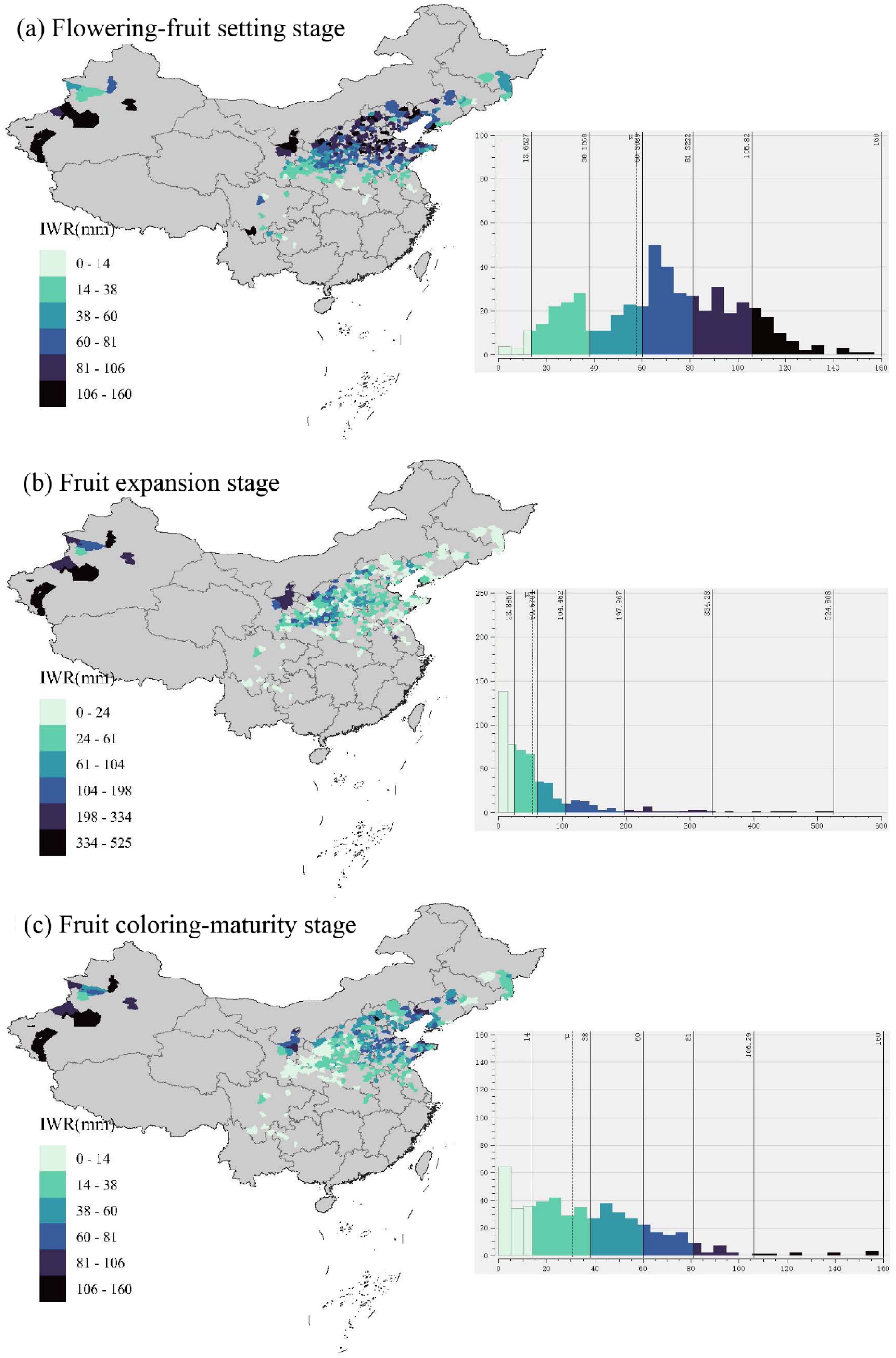
Figure 8. Spatial distribution map and frequency histogram of irrigation water requirement (IWR) during apple flowering-fruit setting stage (a), fruit expansion stage (b), and fruit coloring-maturity stage (c) in the whole apple-producing areas of China.
3.4 Spatiotemporal changing trends of apple water suitability level
The level of water suitability (S) of apples was assessed over time and space in apple production areas of China based on the concepts of effective precipitation and apple water requirements (Figure 9). The annual average water suitability of apples was 0.3 (unsuitable), 0.8 (fairly suitable), and 1.5 (extremely suitable) during the flowering-fruit setting stage, fruit expansion stage, and coloring-maturity stage, respectively. Specifically, during the flowering-fruit setting stage, the water suitability for apples reached an extremely suitable level in 1968, with a level of 1.18. However, from 1970 to 2020, the water suitability of apples during this stage remained consistently low. These findings underscored the prevalence of water scarcity during the apple flowering and fruit-setting stage in China over numerous years. During the fruit expansion stage, the highest water suitability occurred in 1962, with the highest value of 2.19, which was an excessive moisture level. We also found the water suitability was in the suitable and extremely suitable range (light pink area in Figure 9) during this stage of apples in most years. Moreover, the inter-annual fluctuations of water suitability exhibited considerable variability during apples during the coloring-maturity stage. These analyses showed more attention should be paid to the water suitability during the flowering-fruit setting stage.
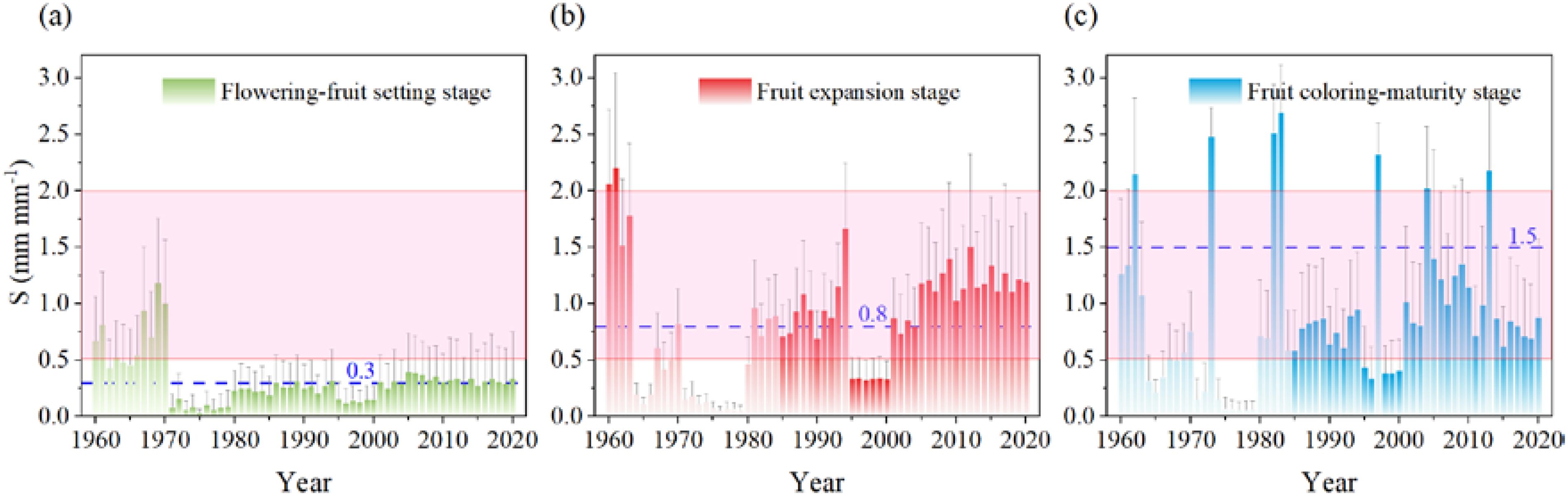
Figure 9. Temporal variation of water suitability (S) in apple flowering-fruit setting stage (a), fruit expansion stage (b), and fruit coloring-maturity stage (c) from 1960 to 2020 in the whole apple-producing areas of China (The blue dotted line denotes the average line, while the light pink area represents the range from the suitable level to the extremely suitable level of apple water requirements.).
The spatial distribution map and histogram showed that the water suitability of most apple-producing counties in China was less than 0.4 (Figure 10a) during the flowering-fruit-setting stages, which was an extremely unsuitable level. These areas were mainly concentrated in Xinjiang apple production areas, the northwest parts of Loess Plateau production areas and the northern parts of Bohai Bay production areas. Conversely, during the fruit expansion stage, the water suitability level in most areas was extremely suitable, ranging from 1.0 to 2.0 (Figure 10b). The apple production areas of the Southwestern Cool Highlands exhibited excessively high water suitability levels (>2.0), and the few areas (e.g. Xinjiang and Ningxia province) experienced water shortages. During the apple coloring-maturation stage, the areas with excessive humidity not only included the Southwestern Cool Highlands but also extended to some counties in the southwestern Loess Plateau (Figure 10c).
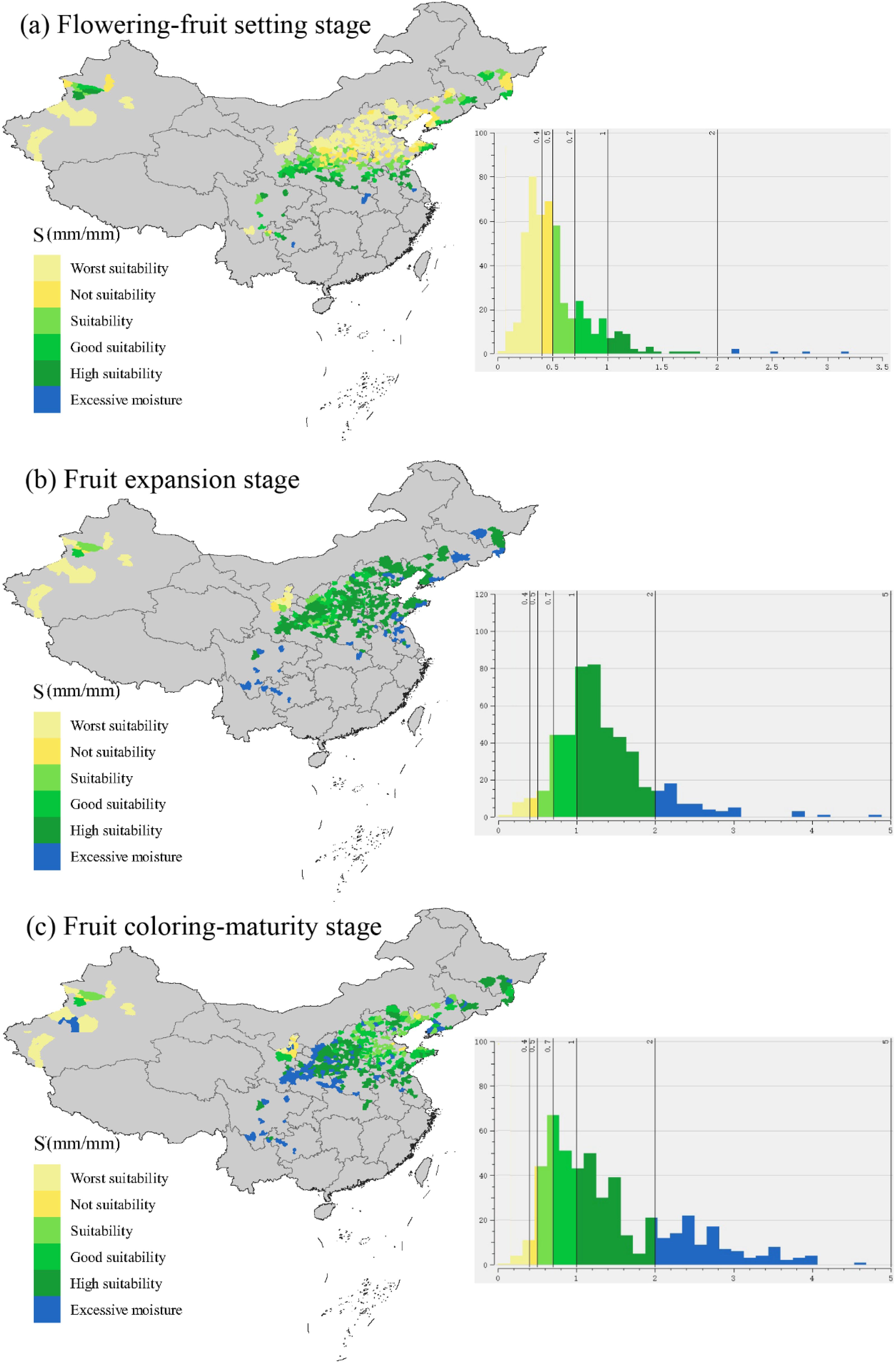
Figure 10. Spatial distribution map and frequency histogram of water suitability (S) during apple flowering-fruit setting stage (a), fruit expansion stage (b), and fruit coloring-maturity stage (c) in the whole apple-producing areas of China.
4 Discussion
4.1 Enhanced precision in apple water requirement estimation through dynamic phenological modeling
This study assessed apple water requirements by integrating accurate phenological simulation. The phenology modeling analysis showed that the Alternating model exhibited the highest accuracy, while the Unichill model showed the largest simulation error. The model ensemble method addressed the issue of low accuracy caused by arbitrary model selection (Dai et al., 2022; Ru et al., 2023b). Then, the time window of the flowering-fruit setting, fruit expansion, and fruit coloring-maturity stages was quantified. Previous studies used the Penman-Monteith equation to assess crop water requirements, it was common practice to vaguely define flowering and fruit-setting stages of all study areas within a broad time range, such as considering the period from March 1 to April 30 as the flowering and fruit-setting stage (Liu et al., 2024; Nyolei et al., 2019). In contrast, our study offered a more targeted and location-specific phenology dates (Figure 4). The dynamic phenological data simulated by the phenological model enabled the calculated apple irrigation water requirement to be adjusted according to the length of the phenological dates. For instance, when the temperature is high in a certain year, flower-fruiting is likely to occur earlier, and the water requirement may also be earlier. The data simulated by the phenological model could dynamically match the temporal distribution of apple water requirements.
4.2 Water requirement dynamics in different phenophases and regions
An in-depth analysis of the details of water requirements throughout the various stages of apple growth formed the foundation of sustainable apple cultivation practices. According to our results, the stages of apple flowering-fruit setting, fruit expansion, and coloring-maturity showed distinctly different water requirements (Table 5). At the beginning of the flowering-fruit setting stage, the apple tree began its reproductive process, a modest water requirement of about 120 mm was essential for maintaining the delicate water balance in apples. This finding is corroborated by the conclusions of a field-scale fruit study conducted in the United States (Dombrowski et al., 2022). As the apple tree progressed into the fruit expansion stage, characterized by vigorous growth and cellular proliferation, the water requirement increased dramatically. With an average requirement of 319 mm, this stage became the peak of water consumption, reflecting the heightened metabolic activity involved in fruit development (Moriondo et al., 2015). Subsequently, as the apple approached the coloring-maturity stage, there was a shift from vegetative to reproductive functions, accompanied by a corresponding decrease in water requirements. Despite this reduction, an average demand of 113 mm persisted, highlighting the continued importance of water management throughout the growing season. Importantly, the observed trend of increasing water requirements during stages of active growth and development aligned well with the physiological demands of the apple tree as previously reported (Dang et al., 2020; Wang et al., 2021a).
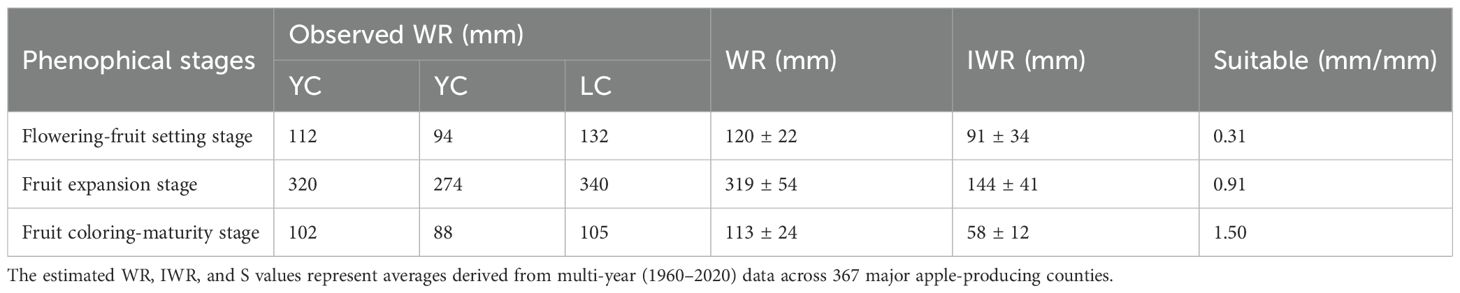
Table 5. Observed Water Requirements (WR) of 3 representative apple producing counties (Yuncheng [YC], Luochuan [LC], and Beijing [BJ]) and the indicator of WR, Irrigation Water Requirements (IWR), and Suitability (S) estimated by FAO 56 Penman-Monteith (P-M) ETo equation.
The combination of spatial analysis and frequency distribution histograms provided additional insights from an inter-regional perspective on dynamic water requirements. During the flowering-fruit setting stage, regions such as the northwestern areas of the Loess Plateau and the central Bohai Bay areas emerged as areas with elevated water needs. Conversely, there were areas with diminished water requirements along the eastern edges of Gansu, Henan, and Heilongjiang provinces. The accompanying frequency distribution histogram revealed the peak frequency of the normal distribution within the 101 to 120 mm range of water requirement. These statistical findings not only confirmed the spatial differences observed but also reinforced the prevalence of moderate water requirements during this stage of apple development. Transitioning into the fruit expansion stage, regions spanning Xinjiang, Ningxia, Shaanxi, and Shanxi provinces emerged as focal points of heightened water requirements. The frequency distribution histogram echoed this narrative, showing a notable shift towards higher ranges of water requirement, with the 250 to 301 mm range being the central point of frequency distribution. As the apple tree progressed towards the coloring-maturity stage, there was a subtle adjustment in water requirements, reflecting the transition from vegetative to reproductive functions (Juillion et al., 2022; Ye et al., 2020). The frequency distribution histogram demonstrated this trend, with the 101 to 136 mm range of water requirement dominating the frequency distribution landscape, representing the nuanced adjustments in water needs during apple maturation.
4.3 Variations of irrigation water requirements in different phenophases and regions
For irrigation water requirements (IWR) of apples, the order was as follows: fruit expansion > flowering-fruit setting > coloring-maturity. The differential IWR inherent in each phenophical phase aligned with the WR pattern of apples. The large difference between the average stability and interannual variation (standard deviation) of IWR at each stage reflected the dynamic impact of climatic factors on apple irrigation water requirements in the three phenological stages of apple (Gao et al., 2021; Gush et al., 2019). Spatially, the results unveiled a compelling correlation between latitude and IWR, with higher latitudes corresponding to elevated water demands during the flowering-fruit setting stage (Gao et al., 2018). This spatial gradient mirrored China’s precipitation patterns, with regions such as Xinjiang exhibiting the highest IWR due to diminished rainfall. Regionally, the Bohai Bay and Loess Plateau regions emerged as focal points of heightened water demand during distinct phenological stages, indicative of localized climatic influences shaping orchard water requirements. Complementing our spatial analysis, frequency distribution histograms showed the prevalence of specific IWR ranges across apple-producing counties. Notably, the concentration of IWR between 60 and 81 mm during the flowering-fruit setting stage underscored the prevalence of moderate water demands during this critical phase of apple development. In essence, the amalgamation of spatial analysis and temporal variability offered a comprehensive understanding of the complex nexus between climatic factors, geographical gradients, and agronomic demands shaping irrigation water requirements within apple-producing regions of China. However, this study did not perform separate analyses of specific biotic and abiotic stressors—such as diseases, pests, soil salinization, or nutrient deficiencies—which are known to significantly influence apple water use efficiency and suitability. Future research could benefit from incorporating crop–environment–stress coupling models, such as integrating disease indices into water suitability assessments or employing crop growth models to simulate water demand dynamics under varying stress conditions.
4.4 Water suitability dynamics in different phenophases and regions
The assessment of water suitability (S) in apple production counties of China offered valuable insights into the dynamic interplay between effective precipitation, apple water requirements, and regional climatic conditions. During the flowering-fruit setting stage, while a notable exception occurred in 1968, when water suitability reached an extremely suitable level, subsequent years witnessed consistently low water suitability levels, indicating recurrent water stress during this pivotal phase of apple development. During the fruit expansion stage, a contrasting picture emerged, characterized by a predominance of suitable to extremely suitable water suitability levels in most years. However, a notable anomaly in 1962, when excessively high water suitability levels were observed, highlighted the potential risks associated with excessive moisture during this developmental phase. Despite this anomaly, the overall trend underscored the favorable water conditions for fruit growth, development during the fruit expansion stage. The coloring-maturity stage unveiled a mosaic of spatial variability in water suitability, with regions experiencing both excessive humidity and water shortages. While areas such as the Southwestern Cool Highlands exhibited excessively high water suitability levels, extending beyond the optimal range, counties in the southwestern Loess Plateau faced challenges associated with excessive humidity.
Spatial analysis further clarified the disparities in water suitability across apple-producing counties, with concentrations of extremely unsuitable levels during the flowering-fruit setting stage in Xinjiang, the northwest parts of the Loess Plateau, and the northern parts of the Bohai Bay production areas. Conversely, during the fruit expansion stage, most regions exhibited favorable water suitability levels, with exceptions noted in Xinjiang and Ningxia province, indicating localized water scarcity challenges. The coloring-maturation stage presented a nuanced spatial distribution, with areas of excessive humidity extending from the Southwestern Cool Highlands to select counties in the southwestern Loess Plateau. These spatial nuances showed the localized climatic influences shaping water availability during apple coloring-maturation stages. This emphasized the need for specific irrigation management plans to address risks related to water suitability challenges. Compared to previous research (Wang et al., 2020b; Ye et al., 2020), this study offers a more detailed understanding of spatial and temporal dynamics, enabling stakeholders to implement targeted interventions for improved water management practices.
5 Conclusion
This study provides a comprehensive macroscopic evaluation of apple water requirements and suitability levels at the county scale in China by integrating phenological modeling-derived phenophases with the FAO 56 Penman-Monteith (P-M) ETo approach. The results reveal significant variation in water needs and suitability across different phenological stages and regions, highlighting the critical importance of tailored water management strategies. The highest average water requirements were observed during the fruit expansion stage, while the flowering-fruit setting stage exhibited the lowest water suitability, particularly in regions such as Xinjiang, the northwest Loess Plateau, and northern Bohai Bay. Conversely, excessive moisture was noted in the Southwestern Cool Highlands during the fruit expansion and coloring-maturity stages. These findings underscore the necessity for region-specific water management practices to enhance apple quality and sustainability. By identifying phenological windows with varying water suitability, this study offers valuable insights for optimizing irrigation practices and supports the sustainable development of the apple industry in China.
Data availability statement
The original contributions presented in the study are included in the article/Supplementary Material. Further inquiries can be directed to the corresponding author/s.
Author contributions
XR: Conceptualization, Data curation, Formal analysis, Validation, Visualization, Writing – original draft, Writing – review & editing. TH: Formal analysis, Investigation, Writing – review & editing. GY: Methodology, Writing – review & editing. YH: Formal analysis, Writing – review & editing. ZZ: Supervision, Writing – original draft. QY: Supervision, Writing – original draft. JH: Funding acquisition, Software, Supervision, Writing – review & editing.
Funding
The author(s) declare that financial support was received for the research and/or publication of this article. This research was supported by the Zhejiang Provincial Natural Science Foundation of China (No. ZCLQN25C1502), the National Key Research and Development Program of China (No. YFD1900700), the National Natural Science Foundation of China (No. 52079115), the start-up fund from the Zhejiang Agricultural & Forestry University (No. 2024LFR084), the Natural Science Foundation of Shaanxi Province of China (No. JC-YB-279), and the “111 Project” (No. B12007) of China.
Conflict of interest
The authors declare that the research was conducted in the absence of any commercial or financial relationships that could be construed as a potential conflict of interest.
Generative AI statement
The author(s) declare that no Generative AI was used in the creation of this manuscript.
Publisher’s note
All claims expressed in this article are solely those of the authors and do not necessarily represent those of their affiliated organizations, or those of the publisher, the editors and the reviewers. Any product that may be evaluated in this article, or claim that may be made by its manufacturer, is not guaranteed or endorsed by the publisher.
Supplementary material
The Supplementary Material for this article can be found online at: https://www.frontiersin.org/articles/10.3389/fpls.2025.1572647/full#supplementary-material
References
Allen, R., Pereira, L., Raes, D., Smith, M. (1998). Crop evapotranspiration: guidelines for computing crop water requirements. Irrigation and drainage paper 56 Vol. 300 (Rome, Italy: Food and Agriculture Organization (FAO).
Bal, S. K., Chandran, M. A. S., Madhavan, S. V., Rao, A. V. M. S., Manikandan, N., Praveen Kumar, R., et al. (2022). Water demand in maize is projected to decrease under changing climate in India. Sustainability 14, 1419. doi: 10.3390/su14031419
Basler, D. (2016). Evaluating phenological models for the prediction of leaf-out dates in six temperate tree species across central Europe. Agr. Forest. Meteorol. 217, 10–21. doi: 10.1016/j.agrformet.2015.11.007
Benmoussa, H., Ben Mimoun, M., Ghrab, M., Luedeling, E. (2018). Climate change threatens central Tunisian nut orchards. Int. J. Biometeorol 62, 2245–2255. doi: 10.1007/s00484-018-1628-x
Blümel, K., Chmielewski, F. M. (2012). Shortcomings of classical phenological forcing models and a way to overcome them. Agr. Forest. Meteorol. 164, 10–19. doi: 10.1016/j.agrformet.2012.05.001
Caffarra, A., Donnelly, A., Chuine, I. (2011). Modelling the timing of Betula pubescens budburst. II. Integrating complex effects of photoperiod into process-based models. Clim. Res. 46, 159–170. doi: 10.3354/cr00983
Chen, K. (2011). Apple tree transpiration rule and apple orchard evapotranspiration features in hilly area of Shandong province. Tai’an, Shandong, China: Shandong Agricultural University.
Chen, S., He, C., Huang, Z., Xu, X., Jiang, T., He, Z., et al. (2022). Using support vector machine to deal with the missing of solar radiation data in daily reference evapotranspiration estimation in China. Agr. Forest. Meteorol. 316, 108864. doi: 10.1016/j.agrformet.2022.108864
Chuine, I. (2000). A unified model for budburst of trees. J. Theor. Biol. 207, 337–347. doi: 10.1006/jtbi.2000.2178
Chuine, I., Cour, P., Rousseau, D. D. (1999). Selecting models to predict the timing of flowering of temperate trees: implications for tree phenology modelling. Plant Cell Environ. 22, 1–13. doi: 10.1046/j.1365-3040.1999.00395.x
Dai, W., Jin, H., Liu, T., Jin, G., Zhang, Y., Zhou, Z. (2022). Applying ensemble learning in ecophysiological models to predict spring phenology. For. Ecol. Manage. 505, 119911. doi: 10.1016/j.foreco.2021.119911
Dang, H., Feng, J., Wang, M., Chen, S., Que, X. E. (2020). A study on water demand characteristics of apple trees in different growing stages in the Loess Plateau Area. Int. J. Fruit Sci. 37, 659–667. doi: 10.13925/j.cnki.gsxb.20190394
Dombrowski, O., Brogi, C., Hendricks, H. J., Zanotelli, D., Bogena, H. (2022). CLM5-FruitTree: a new sub-model for deciduous fruit trees in the Community Land Model (CLM5). Geosci. Model Dev. 15, 5167–5193. doi: 10.5194/gmd-15-5167-2022
Doorenbos, J., Pruitt, W. O., Aboukhaled, A., Damagnez, J., Dastane, N. G., Van Den Berg, C., et al. (1977). Crop water requirements. Available online at: https://openknowledge.fao.org/handle/20.500.14283/S8376E (Accessed February 3, 2025).
Dzikiti, S., Volschenk, T., Midgley, S. J. E., Lötze, E., Taylor, N. J., Gush, M. B., et al. (2018). Estimating the water requirements of high yielding and young apple orchards in the winter rainfall areas of South Africa using a dual source evapotranspiration model. Agric. Water Manage. 208, 152–162. doi: 10.1016/j.agwat.2018.06.017
Elnashar, A., Abbas, M., Sobhy, H., Shahba, M. (2021). Crop water requirements and suitability assessment in arid environments: A new approach. Agronomy 11, 260–271. doi: 10.3390/agronomy11020260
Ettinger, A. K., Chamberlain, C. J., Morales-Castilla, I., Buonaiuto, D. M., Flynn, D. F. B., Savas, T., et al. (2020). Winter temperatures predominate in spring phenological responses to warming. Nat. Clim. Change 10, 1137–1142. doi: 10.1038/s41558-020-00917-3
Fadl, M., Abuzaid, A. (2017). Assessment of land suitability and water requirements for different crops in dakhla oasis, western desert, Egypt. Int. J. Plant Sci. 16, 1–16. doi: 10.9734/IJPSS/2017/33835
Fernandez, E., Schiffers, K., Urbach, C., Luedeling, E. (2022). Unusually warm winter seasons may compromise the performance of current phenology models – Predicting bloom dates in young apple trees with PhenoFlex. Agric. For. Meteorol. 322, 109020. doi: 10.1016/j.agrformet.2022.109020
Gao, X., Li, H., Zhao, X., Ma, W., Wu, P. (2018). Identifying a suitable revegetation technique for soil restoration on water-limited and degraded land: Considering both deep soil moisture deficit and soil organic carbon sequestration. Geoderma 319, 61–69. doi: 10.1016/j.geoderma.2018.01.003
Gao, X., Zhao, X., Wu, P., Yang, M., Ye, M., Tian, L., et al. (2021). The economic–environmental trade-off of growing apple trees in the drylands of China: A conceptual framework for sustainable intensification. J. Clean Prod. 296, 126497. doi: 10.1016/j.jclepro.2021.126497
Gush, M., Dzikiti, S., van der Laan, M., Steyn, M., Manamathela, S., Pienaar, H. (2019). Field quantification of the water footprint of an apple orchard, and extrapolation to watershed scale within a winter rainfall Mediterranean climate zone. Agr. Forest. Meteorol. 271, 135–147. doi: 10.1016/j.agrformet.2019.02.042
Han, X., Chang, L., Wang, N., Kong, W., Wang, C. (2023). Effects of meteorological factors on apple yield based on multilinear regression analysis: A case study of yantai area, China. Atmosphere 14, 183. doi: 10.3390/atmos14010183
Hänninen, H. (1987). Effects of temperature on dormancy release in woody plants. Silva Fennica 21, 279–299. doi: 10.14214/sf.a15476
Harrington, C. A., Gould, P. J. (2015). Tradeoffs between chilling and forcing in satisfying dormancy requirements for Pacific Northwest tree species. Front. Plant Sci. 6. doi: 10.3389/fpls.2015.00120
He, C., Liu, J., Xu, F., Zhang, T., Chen, S., Sun, Z., et al. (2020). Improving solar radiation estimation in China based on regional optimal combination of meteorological factors with machine learning methods. Energy Conv. Manage. 220, 113111. doi: 10.1016/j.enconman.2020.113111
Hublart, P., Ruelland, D., García de Cortázar-Atauri, I., Gascoin, S., Lhermitte, S., Ibacache, A. (2016). Reliability of lumped hydrological modeling in a semi-arid mountainous catchment facing water-use changes. Hydrol. Earth. Syst. Sc. 20, 3691–3717. doi: 10.5194/hess-20-3691-2016
Jia, G., Yang, L., Li, C., An, D., Wang, R., Gao, X. (2020). Characteristics and water suitability of apple at the eastern foot of Taihang Mountains in Central-south Region of Hebei Province. J. Meteor. Environ. 43, 109–114. doi: 10.16765/j.cnki.1673-7148.2020.02.015
Juillion, P., Lopez, G., Fumey, D., Lesniak, V., Génard, M., Vercambre, G. (2022). Shading apple trees with an agrivoltaic system: Impact on water relations, leaf morphophysiological characteristics and yield determinants. Sci. Hortic. 306, 111434. doi: 10.1016/j.scienta.2022.111434
Lijia, W., Xuexi, H. (2014). Transaction costs comparison between cooperatives and conventional apple producers: a case study of northwestern China. Ann. Public. Coop Econ. 85, 233–255. doi: 10.1111/apce.12039
Liu, M., Gao, M., Zheng, E., Wang, J., Chen, M., Feng, S., et al. (2024). Study on soil moisture dyamic characteristics of apple orchard in the Loess plateau of northern Shaanxi. J. Yanan Univ. 25, 1–9. doi: 10.13876/J.cnki.ydnse.230074
Liu, N., Shi, Y., Ding, Y., Liu, L., Peng, S., Xie, Y. (2023). Temporal effects of climatic factors on vegetation phenology on the Loess Plateau, China. J. Plant Ecol. 16, 63–71. doi: 10.1093/jpe/rtac063
Lu, Z., Liu, X., Wang, T., Zhang, P., Wang, Z., Zhang, Y., et al. (2022). Malice at the Gates of Eden: current and future distribution of Agrilus Mali threatening wild and domestic apples. Bull. Entomol. Res. 112, 145–757. doi: 10.1017/S000748532200013X
Luedeling, E., Zhang, M., Luedeling, V., Girvetz, E. H. (2009). Sensitivity of winter chill models for fruit and nut trees to climatic changes expected in California’s Central Valley. Agr. Ecosyst. Environ. 133, 23–31. doi: 10.1016/j.agee.2009.04.016
Meng, Q. Q. (2011). Soil moisture consumption pattern and growth response of hilly apple orchard in the loess plateau. Yangling, Shaanxi, China: Northwest A & F University.
Monteith, J. L. (1965). “Evaporation and environment,” in Symposium on the state and movement of water in living organisms (Cambridge, UK: Cambridge University Press), 205–234.
Moriondo, M., Ferrise, R., Trombi, G., Brilli, L., Dibari, C., Bindi, M. (2015). Modelling olive trees and grapevines in a changing climate. Environ. Modell. Software 72, 387–401. doi: 10.1016/j.envsoft.2014.12.016
Nyolei, D., Nsaali, M., Minaya, V., van Griensven, A., Mbilinyi, B., Diels, J., et al. (2019). High resolution mapping of agricultural water productivity using SEBAL in a cultivated African catchment, Tanzania. Phys. Chem. Earth. 112, 36–49. doi: 10.1016/j.pce.2019.03.009
Oiu, M., Guo, C., Wang, D., Yuan, F., Wang, M., Mu, J., et al. (2018). Analysis of characteristic on drought in different developmental times of maize based on crop water deficit index in Jilin Province. J. Catastrophology 33, 89–98. doi: 10.3969/j.issn.1000-811X.2018.02.017
Panigrahi, P. (2023). Scheduling irrigation for improving water productivity and fruit quality in citrus (cv. Nagpur mandarin) under a dry tropical climate. Irrigation Sci. 41, 511–520. doi: 10.1007/s00271-023-00847-w
Penman, H. L. (1948). Natural evaporation from open water, bare soil, and grass. Proc. R. Soc. London. Ser. A Math. Phys. Sci. 1032, 120–145. doi: 10.1098/rspa.1948.0037
Ru, X., Jiang, Y., Luo, Q., Wang, R., Feng, X., Wang, J., et al. (2023a). Evaluating late spring frost risks of apple in the Loess Plateau of China under future climate change with phenological modeling approach. Sci. Hortic. 308, 111604. doi: 10.1016/j.scienta.2022.111604
Ru, X., Zhou, J., Gong, K., He, Z., Dai, Z., Li, M., et al. (2023b). Climate warming may accelerate apple phenology but lead to divergent dynamics in late-spring frost and poor pollination risks in main apple production regions of China. Eur. J. Agron. 150, 126945. doi: 10.1016/j.eja.2023.126945
Sugiura, T., Fukuda, N., Tsuchida, T., Sakurai, M., Sugiura, H. (2023). Modeling the relationship between apple quality indices and air temperature. Hortic. J. 92, 424–430. doi: 10.2503/hortj.QH-076
Villalobos, F. J., Testi, L., Fereres, E. (2016). “Calculation of Evapotranspiration and Crop Water Requirements,” in Principles of Agronomy for Sustainable Agriculture. Eds. Villalobos, F. J., Fereres, E. (Springer International Publishing, Cham), 119–137. doi: 10.1007/978-3-319-46116-8_10
Vujadinović Mandić, M., Vuković Vimić, A., Fotirić Akšić, M., Meland, M. (2023). Climate potential for apple growing in Norway—Part 2: assessment of suitability of heat conditions under future climate change. Atmosphere 14, 937. doi: 10.3390/atmos14060937
Wales, D. J., Doye, J. P. (1997). Global optimization by basin-hopping and the lowest energy structures of Lennard-Jones clusters containing up to 110 atoms. J. Phys. Chem. A 101, 5111–5116. doi: 10.1021/jp970984n
Wang, S., An, J., Zhao, X., Gao, X., Wu, P., Huo, G., et al. (2020b). Age- and climate- related water use patterns of apple trees on China’s Loess Plateau. J. Hydrol. 582, 124462. doi: 10.1016/j.jhydrol.2019.124462
Wang, J., Liu, T. (2022). Spatiotemporal evolution and suitability of apple production in China from climate change and land use transfer perspectives. Food Energy Secur. 11, e386. doi: 10.1002/fes3.386
Wang, X. A., Tang, J., Whitty, M. (2021b). DeepPhenology: Estimation of apple flower phenology distributions based on deep learning. Comput. Electron. Agr. 185, 106123. doi: 10.1016/j.compag.2021.106123
Wang, H., Wu, C., Ciais, P., Penuelas, J., Dai, J., Fu, Y., et al. (2020a). Overestimation of the effect of climatic warming on spring phenology due to misrepresentation of chilling. Nat. Commun. 11, 4945. doi: 10.1038/s41467-020-18743-8
Wang, X., Zhao, X., Gao, X., Wei, W., Wang, F., Yu, L., et al. (2021a). Simulation on soil moisture and water productivity of apple orchard on the Loess Plateau, Northwest China. J. Appl. Ecol. 32, 201–210. doi: 10.13287/j.1001-9332.202101.012
Yaacoubi, A. E., Oukabli, A., Hafidi, M., Farrera, I., Ainane, T., Cherkaoui, S. I., et al. (2019). Validated model for apple flowering prediction in the Mediterranean area in response to temperature variation. Sci. Hortic. 249, 59–64. doi: 10.1016/j.scienta.2019.01.036
Yang, J., Huo, Z., Wang, P., Wu, D., Ma, Y. (2021). Indicator-based spatiotemporal characteristics of apple drought in North China. Nat. Hazards 108, 2123–2142. doi: 10.1007/s11069-021-04771-0
Ye, M., Zhao, X., Biswas, A., Huo, G., Yang, B., Zou, Y., et al. (2020). Measurements and modeling of hydrological responses to summer pruning in dryland apple orchards. J. Hydrol. 594, 125651. doi: 10.1016/j.jhydrol.2020.125651
Zanotelli, D., Montagnani, L., Andreotti, C., Tagliavini, M. (2019). Evapotranspiration and crop coefficient patterns of an apple orchard in a sub-humid environment. Agric. Water Manage. 226, 105756. doi: 10.1016/j.agwat.2019.105756
Keywords: apple, phenology model, irrigation water requirements, evapotranspiration, water suitability
Citation: Ru X, He T, Yan G, He Y, Zhu Z, Yu Q and He J (2025) Assessing water requirements and suitability for apple growth at county scale in China: a phenological modeling approach during key growth stages. Front. Plant Sci. 16:1572647. doi: 10.3389/fpls.2025.1572647
Received: 07 February 2025; Accepted: 14 April 2025;
Published: 12 May 2025.
Edited by:
Ingo Dreyer, University of Talca, ChileReviewed by:
Bijayalaxmi Mohanty, National University of Singapore, SingaporeGeza Bujdoso, Hungarian University of Agricultural and Life Sciences, Hungary
Copyright © 2025 Ru, He, Yan, He, Zhu, Yu and He. This is an open-access article distributed under the terms of the Creative Commons Attribution License (CC BY). The use, distribution or reproduction in other forums is permitted, provided the original author(s) and the copyright owner(s) are credited and that the original publication in this journal is cited, in accordance with accepted academic practice. No use, distribution or reproduction is permitted which does not comply with these terms.
*Correspondence: Xiaoya Ru, eGlhb3lhQHphZnUuZWR1LmNu; Jianqiang He, amlhbnFpYW5nLWhlQG53c3VhZi5lZHUuY24=