- 1School of Biological Science, Guizhou Education University, Guiyang, China
- 2State Key Laboratory of Hybrid Rice, Laboratory of Plant Systematics and Evolutionary Biology, College of Life Sciences, Wuhan University, Wuhan, China
- 3School of Resources and Environmental Science, Hubei University, Wuhan, China
- 4School of Ecology and Environment, Tibet University, Lhasa, China
- 5Key Laboratory of Plant Resource Conservation and Germplasm Innovation in Mountainous Region (Ministry of Education), College of Life Sciences/Institute of Agro-bioengineering, Guizhou University, Guiyang, Guizhou, China
Introduction: The genus Rosa belongs to the family Rosaceae within the order Rosales, which is one of the more ancient plant lineages. At present, the complete mitochondrial genome of Rosa spp. is still rarely reported, and studies on the mitochondrial genome of Rosa spp. are limited.
Methods: In this study, the R. laevigata mitochondrial genome was sequenced using both Pacbio Sequel II and DNB-SEQ-T7 platforms. The second- and third-generation data for the other five Rosa species were downloaded from the NCBI database. Genome annotation was performed using Geneious, with structural visualization via CPGview. In-depth analyses were conducted, including assessments of non-synonymous/synonymous mutation ratios (Ka/Ks), codon usage bias, collinearity, and the identification of homologous fragments between chloroplast and mitochondrial genomes. Finally, we employed the maximum likelihood (ML) methods to analyze the phylogenetic relationships among R. laevigata and other Rosa species.
Results: The chloroplast genome sizes ranged from 156,342 bp (R. laevigata) to 157,214 bp (R. agrestis). The GC content varied from 37.2% to 37.3%, and the number of genes ranged from 129 to 131. The mitochondrial genomes were all circular, with lengths between 271,191 bp and 338,975 bp, containing 52 to 59 genes. Codon usage analysis indicated a preference for A/T-ending codons in both chloroplast and mitochondrial genes. Four highly differentiated regions (rps19, ndhF, ycf1, and psbM-trnD-GUC) in the plastomes of the 7 Rosa species were identified, which can serve as molecular markers for future species identification and studies of genetic diversity. Compared to PCGs of plastome, mitochondrial PCGs displayed a higher non-synonymous to synonymous ratio. We also observed extensive gene transfer between the mitochondria and chloroplasts, particularly with the rrn16 and rpl23 genes, which are commonly found in Rosa species. These gene transfer events likely occurred in the ancestor of Rosa around 4.46 Mya. Estimates of divergence events indicate that rapid differentiation among Rosa species took place around 4.46 Mya, potentially influenced by the uplift of the Qinghai-Tibet Plateau during the Late Miocene.
Discussion: This study enriches the genetic resources of the Rosa genus and lays the groundwork for the development of molecular markers, phylogenetic analyses, and research into the evolution of organelle genomes.
1 Introduction
The genus Rosa belongs to the family Rosaceae within the order Rosales, which is one of the more ancient plant lineages. Rosa species are semi-woody perennials that are cultivated globally as ornamental plants (Gahlaut et al., 2021). With a diverse array of species and colors, Rosa species are among the most diverse horticultural crops. They have extensive applications in landscaping, home gardening, perfumery, cosmetics, and pharmaceuticals, offering substantial economic, aesthetic, and medicinal value to both people’s lives and industries. Currently, approximately 200 species of the Rosa genus are recognized worldwide, distributed mainly in cold-temperate to subtropical regions, including Asia, Europe, North America, and North Africa. In China, there are 95 species, 65 of which are endemic, such as Rosa taronensis, R. omeiensis, and R. longicuspis (Wu and Chen, 1974; Lu et al., 2003; Joichi et al., 2005; Potter et al., 2007; Fougere-Danezan et al., 2015). The classification of the Rosa genus can be challenging due to blurred species boundaries and complex interspecific relationships, which are often further complicated by trait similarities resulting from artificial breeding. Consequently, Focke’s subgenus concept is currently applied in China to divide the Rosa genus into two subgenera: Subgen. Rosa and Subgen. Hulthemia, which includes 7 lineages and 9 groups (Wu and Chen, 1974; Lu et al., 2003; Joichi et al., 2005; Bruneau et al., 2007). Issues such as unclear species delimitation, low sequence variation, and incomplete lineage sorting (ILS) have posed significant challenges to the molecular taxonomy of Rosa species. Advances in sequencing technology have made integrated molecular taxonomic studies utilizing plastomes, ribosomal genes, and nuclear genome fragments increasingly mainstream in plant taxonomy (Zhu and Gao, 2015; Zhang et al., 2020). Although the understanding of the Rosa genus dates back quite some time, several factors present significant challenges to its classification. The Rosa genus is widely distributed, with many species capable of hybridizing, which has led to multiple instances of chromosomal duplication (Zong et al., 2024). This contributes to numerous phenotypic similarities among species, further complicated by human interventions such as hybrid breeding and transcontinental migrations. As a result, the genus includes similar traits, along with challenges like multiple synonyms for the same species and inconsistent phenotypic expressions across different regions. To date, several researchers have attempted to reconstruct the phylogenetic relationships within the Rosa genus. However, integrating molecular taxonomy, cytological taxonomy, and classical taxonomy has not yielded satisfactory results. Many of the traits used for characterization in the Rosa genus appear remarkably similar, yet significant differences emerge at the cellular and molecular levels, making it challenging to consolidate them into a cohesive phylogenetic framework. Thus, the phylogenetic relationships within the Rosa genus remain a pressing issue in plant taxonomy.
However, organelle genomes still hold significant application in these analysis mentioned above. These genomes play a crucial role in species identification and the reconstruction of evolutionary histories, which conserved genetic features and low levels of gene flow (Smith and Keeling, ,P, 2015; Wang et al., 2024b). Meanwhile, due to the limited availability of genomic data, organelle genomes remain the predominant method for phylogenetic studies of Rosa species. The plastome is an organelle with a semi-autonomous genetic system that exists independently of the nuclear genome. Since the first plastome from the genus Rosa was reported in 2014 (Yang et al., 2014), the NCBI has cataloged 51 complete plastomes from various Rosa species. Phylogenetic studies based on these plastomes indicate that the phylogenetic relationships among Rosa species are generally consistent with current taxonomic classifications (Engler System) (Niu et al., 2022; Shen et al., 2022). However, many Rosa species remain unrepresented, Highlighting the need for further sequencing of unreported species to enhance our understanding of phylogenetic relationships within the genus Rosa. The mitogenome also features an independent semi-autonomous genetic system separate from the nuclear genome. However, unlike plastomes, mitogenomes in higher plants display substantial size variation and structural complexity, ranging from a few kb to several Mb, and can be linear, circular, or multi-circular (Guo et al., 2023; Zhang et al., 2024). Although mitogenome sequencing has been completed for many plant species (Wu et al., 2020; Bi et al., 2023; Wang et al., 2023), research on the mitogenomes of Rosa species is quite limited. The mitogenome of R. chinensis was first reported by Raymond et al (Raymond et al., 2018), followed by the report of R. angusta’s mitogenome by Park et al. in 2020 (Park et al., 2020). In this study, we performed PacBio HiFi sequencing of R. laevigata, along with the assembly and annotation of their mitochondrial genomes in conjunction with 5 other Rosa species (R. agrestis, R. canina, R. roxburghii, R. spinosissima, and R. sterilis) for which third-generation sequencing data are currently available. Prior to this study, only 3 mitogenomes (R. rugosa, R. chinensis, R. hybrid) were available for the entire Rosa genus. Our findings substantially enrich this genomic resource by adding multiple new mitochondrial sequences.
Our study annotated and assembled the mitogenomes and plastomes of 6 previously unreported Rosa species, thereby enriching the Rosa organellar genome database. Additionally, we conducted intergeneric comparative analyses of the reported plastomes to investigate genomic characteristics, evolutionary relationships, and phylogenetic connections, providing a foundational basis for future research on Rosa organellar genomes. This study aims to assemble the plastomes and mitogenomes of previously unreported Rosa species. By combining these new sequences with existing plastomes data, we seek to reconstruct phylogenetic relationships within the genus, explore the connections and classification boundaries among various species groups, and identify regions of genetic variability. The findings from this research will provide valuable insights into the development of molecular markers for the Rosa species, as well as for phylogenetic studies and taxonomic revisions.
2 Results
2.1 Characterization of organelle genes
The complete plastomes of the 7 Rosa species ranged in length from 156,342 bp (R. laevigata) to 157,214 bp (R. agrestis), with a maximum difference of 872 bp and a minimum difference of 43 bp (Figure 1; Table 1). The GC content varied between 37.2% and 37.3%. Minor differences were observed in the number of genes across species, which ranged from 129 to 131, as shown in Table 1. The mitogenomes of all 7 Rosa species were circular in structure, with lengths ranging from 271,191 bp to 338,979 bp and GC contents between 45.0% and 45.6% (Figure 1; Table 2). Differences in the number of mitochondrial genes among species ranged from 52 to 59. Our results indicated that the mitogenomes exhibited greater fluctuations in both GC content and genome size. By contrast, the plastomes showed more conserved GC content and less variation in genome size throughout evolution.
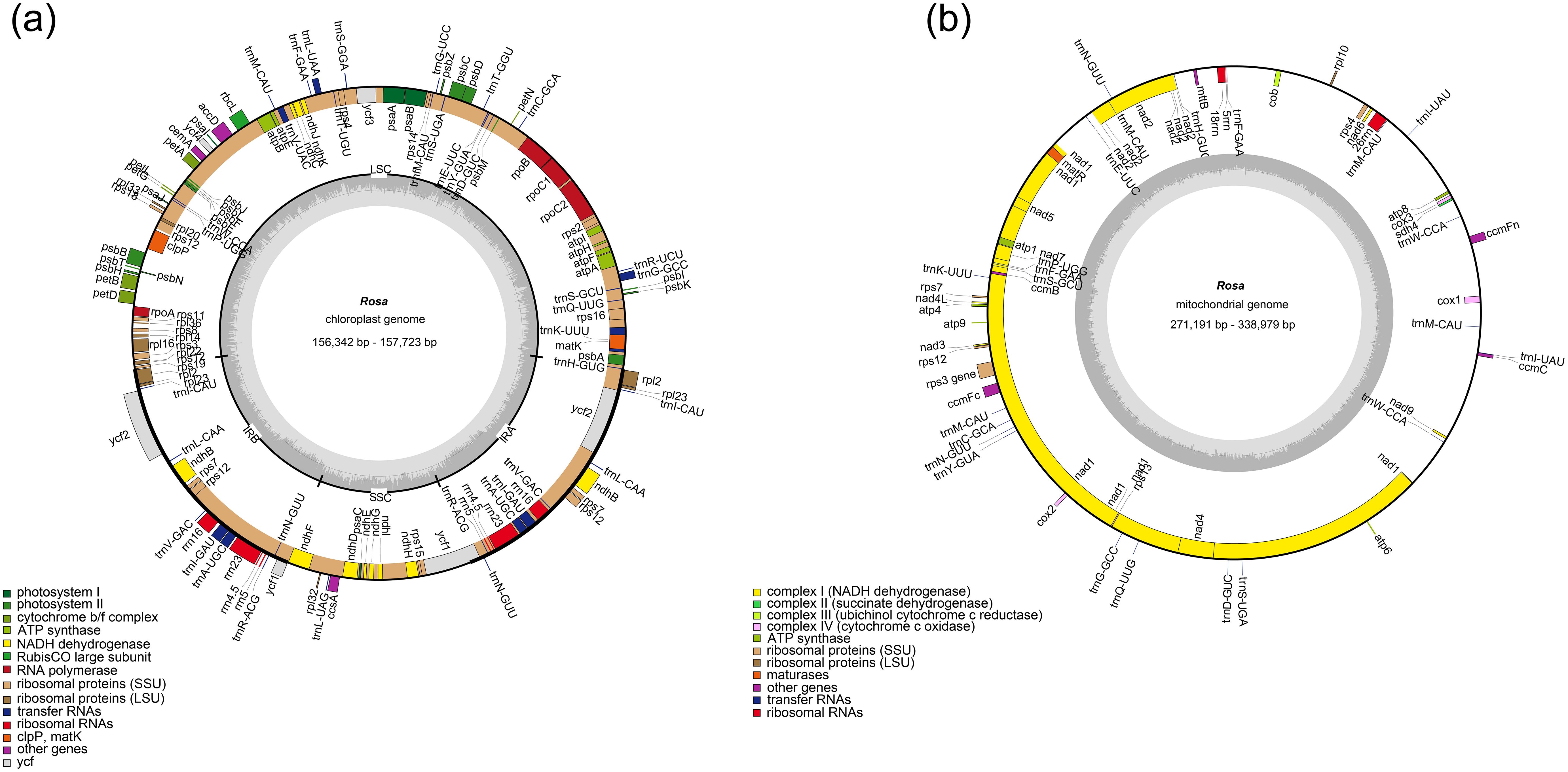
Figure 1. Genome map of (a) the plastome and (b) mitogenome of Rosa species. Genes belonging to functional groups are color-coded on the circle as transcribed clockwise (outside) and transcribed counterclockwise (inside). The darker grey in the inner circle represents the GC content, while the lighter grey represents the AT content.
2.2 Comparative analysis of plastomes
The overall sequence identity analysis of the plastomes of 7 species within the genus Rosa was conducted using mVISTA, with R. rugosa as the reference. The results indicate that the plastomes of these 7 Rosa species exhibit a high degree of sequence similarity, with the inverted repeat (IR) regions being more conserved than the single-copy regions (Supplementary Figure S1). Genetic variation is lower in the coding regions than in the non-coding regions. Three highly variable regions were identified in the coding sequences: rps19, ndhF, and ycf1. Additionally, a highly variable region was observed in the non-coding region (psbM-trnD-GUC) (Supplementary Figure S1). The IR regions, being the most conserved areas of the chloroplast genome, can undergo expansions and contractions at their boundaries, which can result in changes to the overall genome length (Zhang et al., 2024).
This study conducted a comparative analysis of the IR/SSC boundary regions among the plastomes of the 7 Rosa species (Figure 2). We found subtle differences at the IR/SSC boundaries across the 7 species. The IRb/LSC (JLB) junctionlies in the intergenic region between the rps19 and rpl2 genes, while the SSC/IRa (JSA) junction is situated on the ycf1 gene. The difference of regions primarily occur at IRb/SSC (JSB) and IRa/LSC (JLA), with R. rubiginosa, R. spinosissima, and R. sterilis showing IR contraction into the ycf1 gene at JSB. The JLA for R. rugosa and R. laevigata is located between the rpl2 and trnH genes, whereas for the other species, it is positioned between rpl2 and psbA.
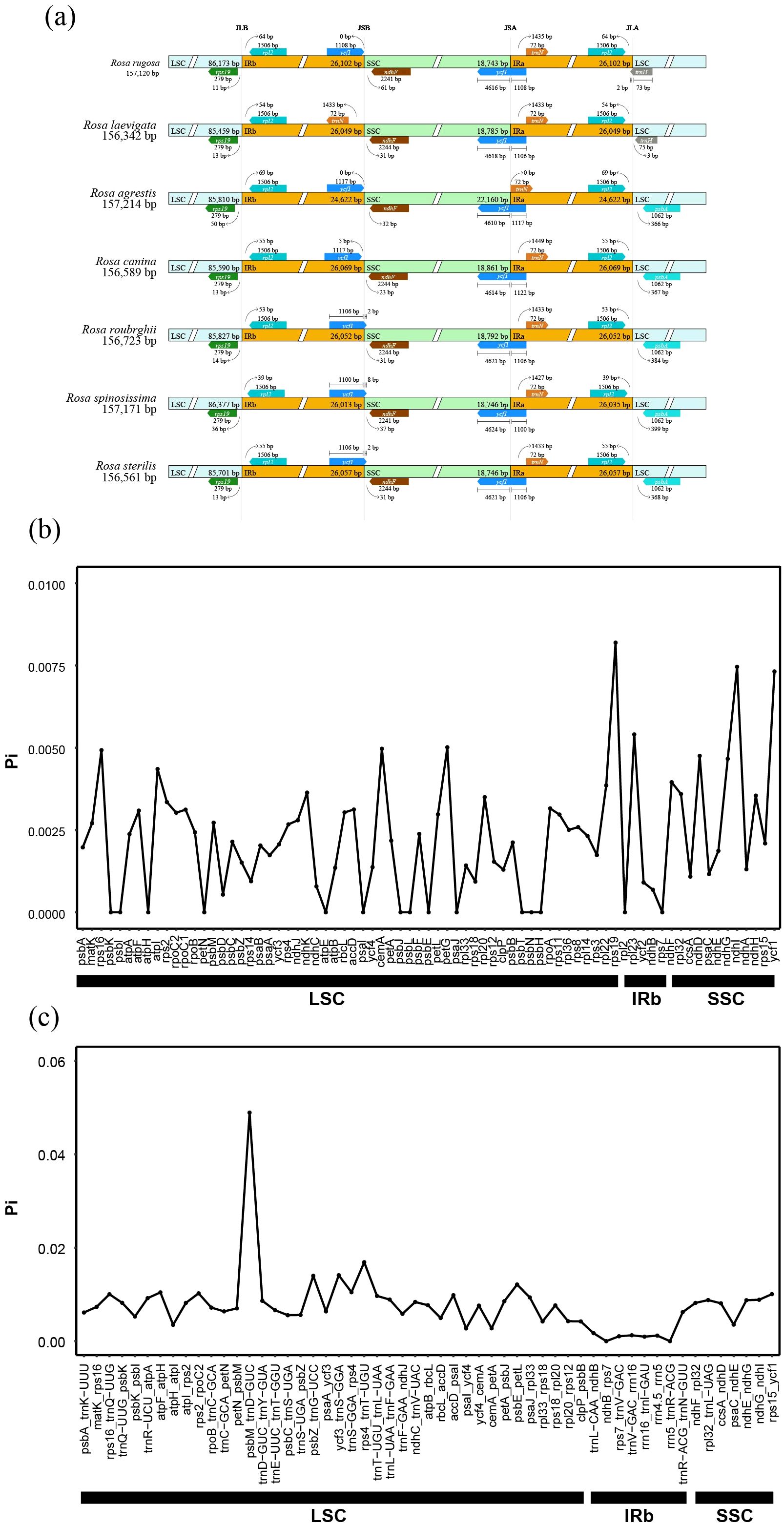
Figure 2. Comparative plastome analysis. (a) Comparison of borders between LSC, SSC and IRs regions across the plastomes of Rosa species. (b) Sliding-window analysis of gene coding regions in plastomes for 7 Rosa species. (c) Sliding-window analysis of non-coding regions in plastomes for 7 Rosa species. X-axis: position of the midpoint of a window; Y-axis: nucleotide diversity (Pi) of each window.
Furthermore, an analysis of nucleotide polymorphism (Pi) based on 78 plastome coding and non-coding regions across the 7 Rosa species revealed notable patterns. Among the 78 PCGs, rps19 exhibited the highest Pi value (0.009182524), followed by ndhI (0.007458405) and ycf1(0.007316403) (Figure 2). In the non-coding regions, the highest Pi value was observed in psbM_trnD-GUC (0.048908538), followed by rps4_trnT-UGU and ycf3_trnS-GGA, with Pi values of 0.016897081 and 0.014111365, respectively (Figure 2). These findings suggested that the Pi values are significantly higher in the non-coding regions compared to coding regions. Additionally, the elevated Pi values in the PCGs, particularly in ndhI and ycf1, indicated a relatively high level of nucleotide polymorphism and highlighted their potential as genetic markers for phylogenetic studies.
2.3 Codon usage and ENc-GC3s analysis
The codon usage of protein-coding genes in the chloroplasts and mitochondria of 7 species of the Rosa genus is illustrated in Figure 3. Phenylalanine (Phe) is the most frequently used amino acid among these organelles, accounting for 3.89% in chloroplast and 6.63% in mitochondria, respectively (Supplementary Table 3). Following Phe, isoleucine (Ile) ranks next, comprising 3.84% and 2.96%. This appears to be a common phenomenon, as similar findings have been observed in the organellar genomes of Aconitum (Xia et al., 2022; Zhang et al., 2024). Additionally, we performed a statistical analysis of codon usage in the PCGs of the plastomes and mitogenomes across the 7 species. In the plastome, there are 31 codons with RSCU values greater than 1, 31 less than 1, and 2 equal to 1. Among these, AGA has the highest RSCU value (1.8114), while CGC has the lowest (0.4725). Of the codons with RSCU values greater than 1, 13 end with A, 15 with U, and 1 with G; none end with C. A similar pattern is observed in the mitogenome, where 27 codons have RSCU values greater than 1, 35 less than 1, and 2 equal to 1. Again, AGA has the highest RSCU value (1.5553) and CGC has the lowest (0.5963). Among these, 11 end with A, 13 with U, 2 with C, and none with G. These findings suggested a general trend, as reported in Aconitum species (Meng et al., 2018; Zhang et al., 2024).
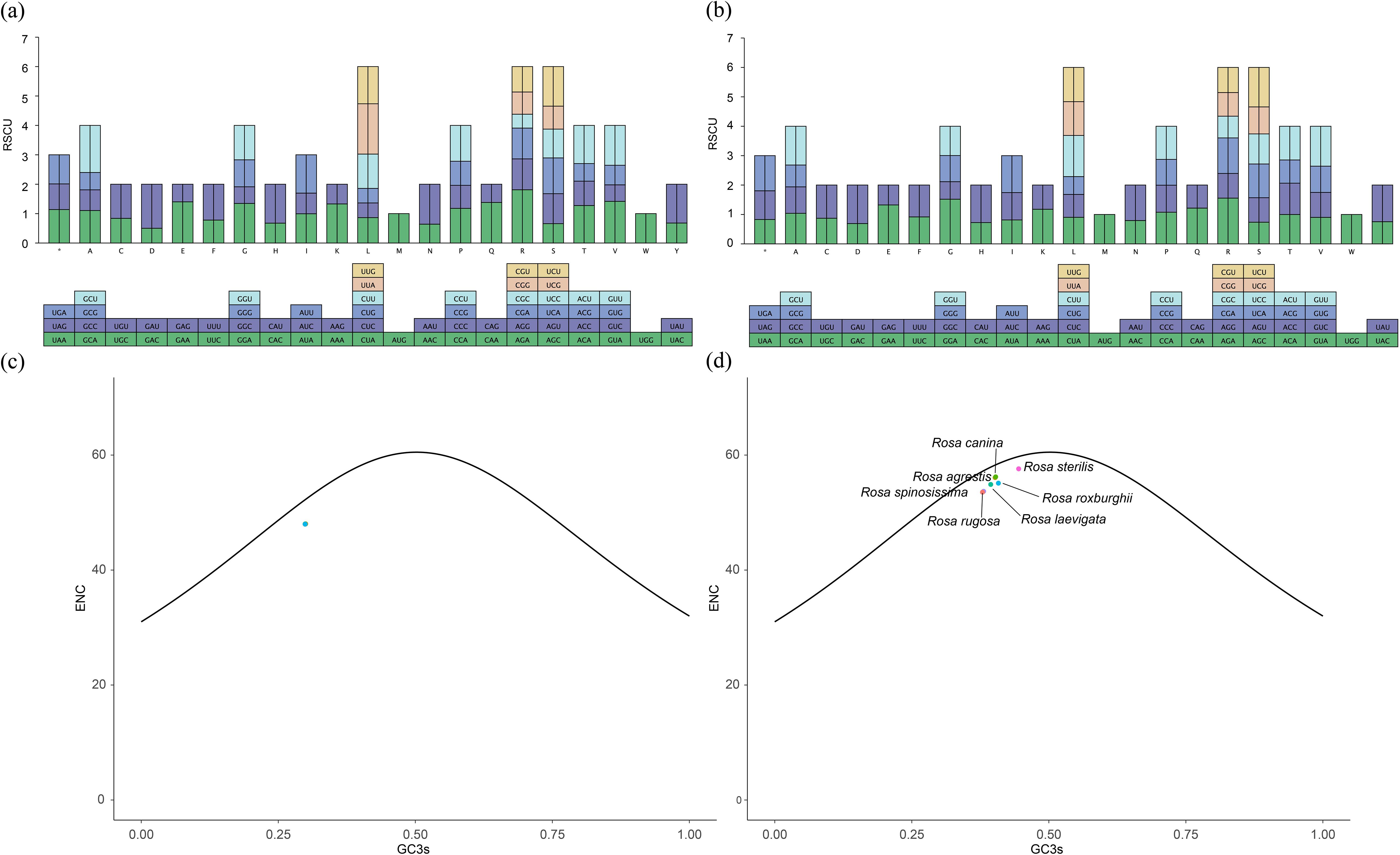
Figure 3. Relative synonymous codon usage (RSCU) and ENC plotted against GC3s based on PCGs of 7 Rosa organelle genes. (a) the plastome genes. (b) the mitogenome gene. (c) the plastome PCGs. (d) the mitogenome PCGs. The solid line indicates the expected curve of positions of genes when the codon usage is merely determined by the GC3s composition.
To explore the relationship between nucleotide composition and codon usage, we analyzed the GC content and codon usage of PCGs in the plastomes and mitogenomes of the 7 Rosa species. The results show that the ENC values for the plastomes range from 47.98 to 48.03, while those for the mitochondrial range from 53.54 to 55.61 (Figure 3; Supplementary Table 4). The plotted data indicate that the ENC values fall below the standard curve rather than aligning with it, suggesting that the PCGs of these species exhibit low codon preference. This implies that the selective pressure on codon usage in the plastomes of Rosa species is greater than the effects of mutation. The smaller variation in codon usage among the plastome PCGs compared to the mitogenome PCGs, indicating that plastomes of Rosa more likely to use similar codons than the mitogenome.
2.4 Mitochondrial structure analysis and gene transfer
Co-linearity block analysis is a common method for identifying evolutionary relationships among closely related species at the genomic level. In this study, we conducted a co-linearity block analysis of 7 Rosa species to investigate structural differences within the mitogenomes of the genus. Our results indicated that, compared to plastomes, the mitogenomes of the 7 species exhibit a more complex co-linear structure (Figure 4c). By examining the positions of mitochondrial homologous genes among the 7 Rosa species, we found that structural rearrangements within the mitogenomes disrupted gene clusters (Figure 4d). Nevertheless, some gene clusters remain conserved across the Rosa species, including 6 larger gene clusters (Figure 4d). The composition of these clusters is as follows: Cluster 1 consists of trnW-CCA-nad9-sdh4-cox3-atp8; Cluster 2 includes nad7-trnP-UGG-trnF-GAA-trnS-GCU-ccmB; Cluster 3 comprises trnS-UGA-trnD-GUC-nad4-trnQ-UUG-trnG-GCC-rps13-cox2; Cluster 4 contains trnY-GUA-trnN-GUU-trnC-GCA-trnM-CAU-ccmFc-rps12-nad3; Cluster 5 is made up of rps4-nad6-rrn26-trnM-CAU; and Cluster 6 consists of trnF-GAA-rrn5-rrn18-mttB-trnH-GUG.
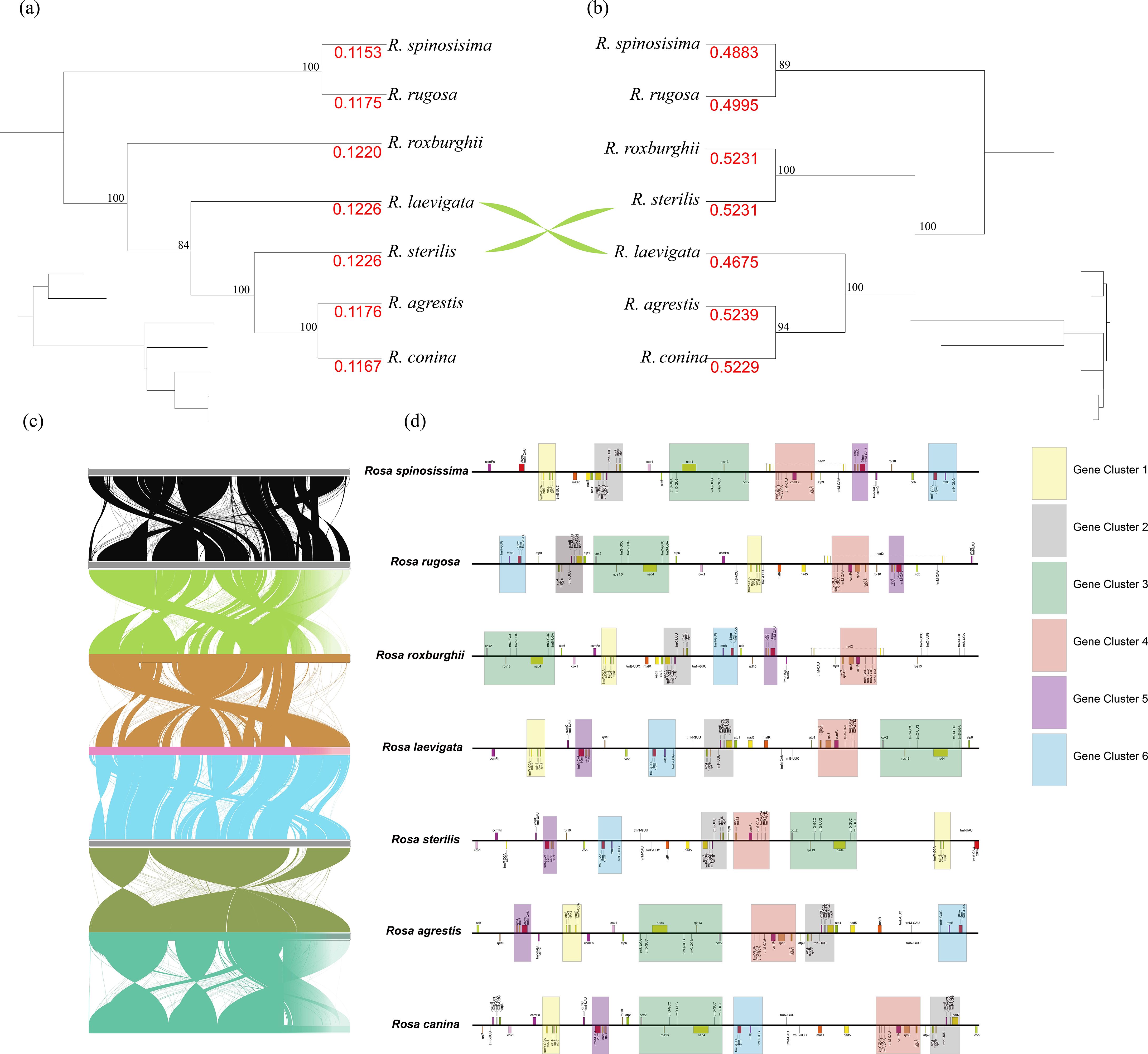
Figure 4. Comparative analysis of organelle genomes. (a) The phylogenetic tree based on 78 pt-PCGs. (b) The phylogenetic tree based on 31 mt-PCGs. (c) Collinearity regions of mitogenomes among 7 Rosa species, and (d) conserved gene blocks in the mitogenomes of 7 Rosa species.
Previous studies have reported the presence of plastome gene remnants in the mitogenome, indicating significant sequence transfer between these two organelles. To investigate this, we performed a homology comparison between the plastomes and mitogenomes of the 7 Rosa species, illustrated in Figure 5. Our analysis detected numerous fragments ranging from 22 to 36 in number, with total lengths spanning from 7,971 bp to 12,132 bp (Supplementary Table 5). Notably, R. spinosissima exhibited the longest fragments, while R. canina had the shortest. Among these fragments, we functionally annotated a total of 14 intact-plastid genes, including 11 PCGs (accD, psbA, psbC, psbD, petD, rpl2, rpl23, rpoA, rpoC1, ycf2, ycf4), 2 tRNA genes (trnI-CAU, trnS-UGA), and 1 rRNA gene (rrn16) (Figure 5). Importantly, we detected that the rrn16 and rpl23 genes are part of the intact plastid genes across all 7 species’ plastomes and mitogenomes.
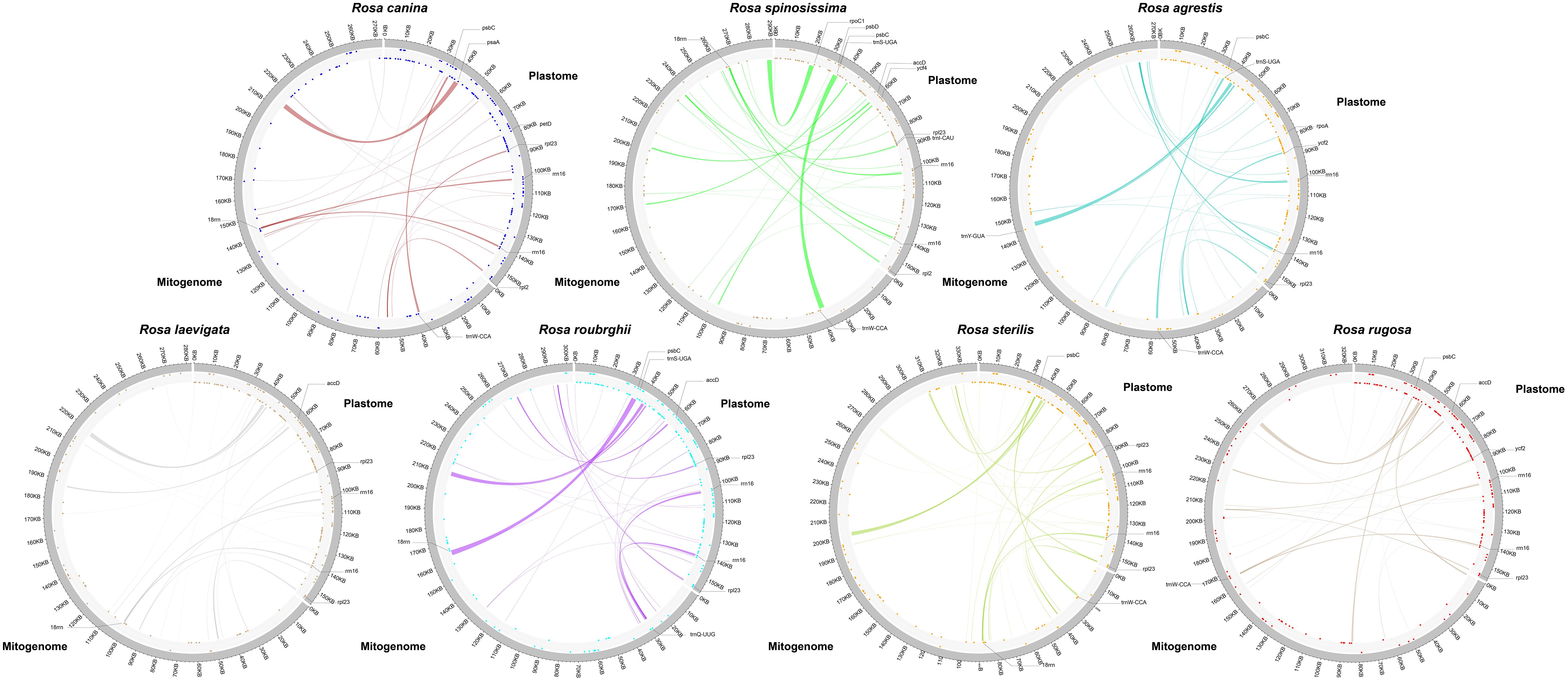
Figure 5. Homologous sequences between the plastome and the mitogenome. In the circle diagram, dots in the inner circle represent genes in the counterclockwise direction and dots in the outer circle represent genes in the clockwise direction. Shaded links represent identified homologous sequences. In homologous sequences complete PCGs are marked with a dash.
2.5 The substitution rates of pt- and mt-PCGs
We assessed the Ka/Ks values of the plastomes and mitogenomes in species of the genus Rosa. Our findings revealed significant differences in the Ka and Ks ratios between the two organelles, with average values for mt-PCG ranging from 0.4675 to 0.5231, while for pt-PCG ranging from 0.1115 to 0.1226 (Figure 6; Supplementary Table 6). Most of the PCGs showed Ka/Ks ratios below 1, indicating that purifying selection has been the dominant evolutionary force acting on these genes. Notably, genes such as nad4L and sdh4 from the mitogenomes exhibited Ka/Ks ratios exceeding 1, suggesting they may have undergone positive selection. The results of the PAML software also supported that these two genes also suffered positive selection in the Rosa species (Supplementary Table 7). This pattern of differential selective pressure across organelles provides valuable insights into the evolutionary dynamics of plastome and mitogenome in Rosa species.
2.6 Phylogenetic analysis and divergence time estimation
Using 78 PCGs of plastome from 53 Rosa species and one outgroup, we constructed a phylogenetic tree with the optimal amino acid substitution model being GTR+F+G4. The phylogenetic results showed strong support, with most nodes having a bootstrap support of 100 (Figure 7a). However, the topologies of the phylogenetic trees based on mt-PCGs and pt-PCGs exhibited slight differences (Figures 4a, b). The mitochondrial tree strongly supported that R. sterilis is sister to R. roxburghii (BS = 100; Figure 4b). In contrast, the plastid analysis indicated a closer relationship between R. sterilis, R. laevigata, R. agrestis, and R. conina (BS = 100; Figure 4a).
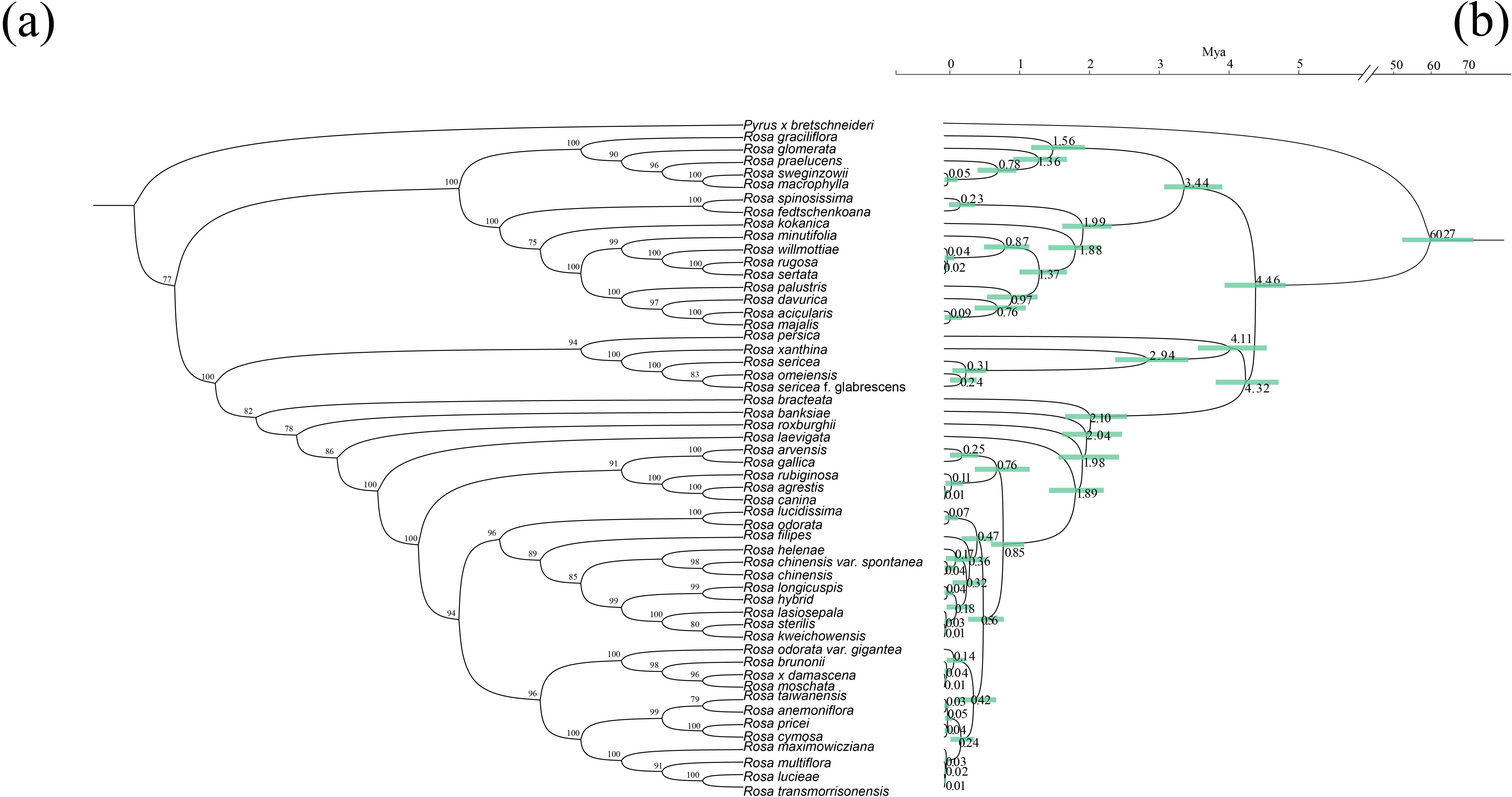
Figure 7. Phylogenetic tree of 7 Rosa species. (a) The phylogenetic tree based on 78 pt-PCGs. (b) Estimates of divergence time of Rosa species inferred from an analysis of 78 complete plastomes using Bayesian MCMCTree with the independent-rates relaxed-clock model. Values are shown next to nodes with mean estimates and 95% confidence intervals.
We estimated divergence times using the mcmctree program based on the plastome maximum likelihood tree, with Pyrus bretschneideri as the root species, resulting in a divergence time of ‘>49.2 Mya <77.1 Mya’. This suggested that species in the Rosa genus diverged from Pyrus around 60.77 Mya. The first divergence within Rosa occurred approximately 4.46 Mya. R. spinosissima and R. fedtschenkoana diverged around 0.23 Mya, while R. roxburghii diverged from other Rosa species approximately 1.98 Mya. R. laevigata diverged from other Rosa species around 1.89 Mya, and R. agrestis and R. canina diverged approximately 0.01 Mya. Finally, R. sterilis diverged from R. kweichowensis around 0.01 Mya (Figure 7b).
3 Discussion
In this study, we assembled and annotated the mitogenomes and plastomes of 6 Rosa species. Consistent with the typical organellar genome structure of Rosa (Jeon and Kim, 2019; Lin et al., 2019; Sun et al., 2020), the plastomes of the 7 Rosa species are all circular, ranging in size from 156,342 bp to 157,214 bp. There are slight variations in gene counts among the species, which range from 129 to 131. The mitogenomes are also circular, but they exhibit greater size variation, ranging from 271,191 bp to 338,975 bp, with gene counts varying from 52 to 59. All 7 species possess three rRNA genes in their mitogenomes, aligning with the observation that most land plants have three rRNA genes (Archibald, 2011; Zhang et al., 2024). Notably, the size of mitogenomes in eukaryotes can vary dramatically, ranging from 6 kb to several tens of Mb (Hikosaka et al., 2010; Sloan et al., 2012). Our results indicate that the mitogenome sizes of the 7 Rosa species range from 271,191 bp to 338,875 bp, which is comparable to the size of R. chinensis (313,448 bp) (Raymond et al., 2018). Therefore, we hypothesize that a mitogenome size around 300 kb is a common characteristic among Rosa species. There was a significant difference between the plastome and mitogenome sizes in Rosa species, a phenomenon that is consistent with previous findings (Smith and Keeling, ,P, 2015; Wang et al., 2024a). Frequent recombination events in plant mitochondrial genomes may have integrated a large amount of exogenous DNA during evolution, possibly leading to large differences in plant mitochondrial genome size. Furthermore, GC content significantly influences amino acid composition and the structural integrity of genomes during evolution (Wang et al., 2023; Wang et al., 2024). The GC content is typically stable at 43%-46% in higher plants (Herbert et al., 2016a). Our analysis of GC content in the plastomes and mitogenomes of the 7 Rosa species reveals that the plastomes exhibit more stable GC content across different species (Table 1), whereas the GC content in mitogenomes shows greater variability (Table 2). The GC content of the mitogenome of Rosa species is in the range of 45.0%-45.6%, which is similar to that of other angiosperm mitogenomes (Chen et al., 2019a; He et al., 2024). This result supported the conclusion that GC content is highly conserved in higher plants (Chen et al., 2019a).
The rearrangements and horizontal gene transfers (HGT) within organelle genomes have been widely demonstrated to play a crucial role in plant evolution, contributing to gene gain and loss, as well as changes in genome size (Notsu et al., 2002; Cheng et al., 2021; Han et al., 2022; Xu et al., 2023). Genes transferred from the plastome to the mitogenome, known as mitochondrial plastid DNA (mitoplastid DNA, MTPT), often manifest as pseudogenes or non-functional rRNA sequences (Bi et al., 2022). In this study, we identified 22 to 36 fragments across 7 Rosa species, with the MTPT of R. spinosissima being the longest at 12,132 bp, significantly surpassing that of other Rosa species. Among these fragments, we detected 14 genes, including 11 PCGs, 2 tRNA genes, and 1 rRNA gene. Notably, we found that rpl23 and rrn16 genes belong to the shared MTPTs across the 7 Rosa species, suggesting that the transfer of the rrn16 and rpl23 genes likely occurred in their common ancestor. Most lost mitochondrial tRNA genes are usually compensated for by corresponding chloroplast-derived tRNA genes, such as trnH-GUG, trnN-GUU, trnS-GGA, and trnW-CCA (Notsu et al., 2002; Sun et al., 2024; Wang et al., 2024a; Wang et al., 2025; Zhang et al., 2025a). Consistent with previous research, we identified two complete tRNA genes in the MTPT, further supporting the notion of frequent tRNA gene transfer from plastome to mitogenome (Han et al., 2022; Xu et al., 2023). HGT is a mechanism of genetic material transfer that has been recognized as a significant driving force in biological evolution (Soucy et al., 2015; Wang et al., 2024). The presence of several chloroplast-derived genes in Rosa may enhance the species’ adaptability to environmental changes. For instance, rpl23 is a gene encoding ribosomal proteins, and studies have shown that seedlings with high expression of RPL23A showed significant increases in fresh weight, root length, proline content, and chlorophyll levels under simulated drought and salt stress (Moin et al., 2017). In summary, previous studies have indicated that tRNA genes are transferred to mitogenome in angiosperms via horizontal gene transfer to maintain their function (Bi et al., 2019). Moreover, Yue et al (Yue et al., 2012). found that annotated homologous pt-genes in the genome play key roles in environmental adaptation. Thus, our findings of intracellular gene transfer between organelle genomes provide deeper insights into the adaptive strategies of Rosa species during their evolutionary history.
Due to maternal inheritance, haploid characteristics, and ease of assembly, plant plastomes have been widely used to resolve evolutionary relationships among different lineages (Mwanzia et al., 2020; Wang et al., 2023). In contrast, the complex structure of mitogenomes presents greater challenges in obtaining complete sequences, which limits their application in phylogenetic studies. In this study, the phylogenetic relationships of the major lineages in the plastome are consistent with the APG IV system and previous research (Fougere-Danezan et al., 2015). Notably, the evolutionary trees of mitogenomes and plastomes for the 7 Rosa species in this study exhibit conflicts. This discrepancy may be attributed to significant differences in the Ka/Ks values of mitochondrial and plastid PCGs (mt-PCGs and pt-PCGs) among the Rosa species (Figure 6; p < 0.01). As shown in Figure 4, the Ka/Ks values of the pt-PCGs for R. laevigata and R. sterilis are more similar, while those for the mt-PCGs of R. roxburghii and R. sterilis are more closely aligned. This may contribute to the observed conflicts between plastid and mitochondrial lineages. Meanwhile, previous studies have found that frequent multiple-nucleotide substitutions in Fragaria mitogenomes caused by microinversions misled the phylogenetic trouble (Fan et al., 2022). we examined the results of the comparison of mitochondrial PCGs in all Rosa species, especially the sdh4 and nad4L genes that showed positive selection, and found that there was a mechanism for mitochondrial PCGs in the Rosa species similar to that of Fragaria (Supplementary Figure S2). Mann-Whitney U tests indicated that the Ka/Ks values of mt-PCGs were significantly higher than those of pt-PCGs (Figure 6). Therefore, we hypothesized that this mechanism leads to significantly lower Ka/Ks values in the pt-PCGs than in the mt-PCGs of Rosa species.
We observed that most Ka/Ks values of PCG are below 1, suggesting strong purifying selection has acted on these genes throughout evolutionary history. Notably, certain genes, including nad4L and sdh4 from the mitogenomes, exhibit Ka/Ks ratios exceeding 1, indicating they may have undergone positive selection. Evidence of positive selection on the sdh4 gene has also been reported in Scheuchzeria palustris (Wang et al., 2023). As a part of the succinate dehydrogenase (SDH) complex, SDH plays a critical role in the tricarboxylic acid cycle and contributes to energy metabolism, stress responses, and signal transduction (Lemos et al., 2002). Additionally, research indicates a positive correlation between SDH activity and male reproductive cell function, underscoring the importance of assessing SDH activity to evaluate mitochondrial function in male gametes (Rao and Sharma, 2001; Ruiz-Pesini et al., 2001). In various crops, including radish (Su et al., 1996), cotton (Huang, 2003), and non-heading cabbage (Yuan, 2005), enzymatic cytochemical studies have revealed differences in SDH activity between sterile and maintainable lines, implying that low SDH activity may be linked to pollen sterility. Therefore, the positive selection of the sdh4 gene could be critical for the activity of male gametes in these species. However, due to the limited number of Rosa mitogenomes analyzed (only 7 species), we advise caution in drawing definitive conclusions until a broader dataset of Rosa mitogenomes become available.
Estimates of divergence times suggest that speciation within the Rosa genus occurred at approximately 4.46 Mya, which aligns with earlier findings regarding Aconitum (Zhang et al., 2024). Divergence within the Aconitum genus is estimated to have taken place around 7.96 Mya, while divergence within Subg. Aconitum taking place about 4.36 Mya. Recent research based on reconstructions of global sea surface temperatures during the late Miocene indicates that a major global cooling event, known as the late Miocene cooling, was triggered by a decrease in CO2 levels by approximately 7 Mya (Tzanova et al., 2015; Herbert et al., 2016b). Concurrently, studies by Chen et al (Chen et al., 2019b). demonstrate that the Tibetan Plateau and the Xining Basin experienced substantial uplift during the late Miocene, accelerating aridification across the Asian interior. Since Rosa and Aconitum are predominantly distributed in southwestern and southeastern China (Supplementary Figure S3), we propose that the combined effects of declining CO2 levels and the uplift of the Tibetan Plateau may have driven rapid species divergence in the region around the late Miocene.
4 Materials and methods
4.1 Plant samples and mitochondrial genome sequencing
Wild-growing R. laevigata plant specimens were collected from Gaopo Town, Huaxi County, Guiyang City, Guizhou Province, China (106°37′ N 26°17′ E), and the samples were identified by Prof. Yuemei Zhao from Guizhou Education University. Fresh leaves were sampled and immediately frozen in liquid nitrogen for DNA extraction. Subsequently, the pressed voucher specimens were numbered (collection number: Rla_00978) and deposited at the herbarium of Guizhou Education University, China. Total genomic DNA was isolated from fresh leaves using the CTAB method (Doyle and Doyle, 1987). The extracted genomic DNA was used to construct DNA libraries, which were sequenced separately on the Illumina and PacBio platforms at GrandOmics (Wuhan). Second-generation sequencing was performed using the DNB-SEQ-T7 platform, while third-generation sequencing was conducted on the PacBio Sequel II platform. The second- and third-generation data for the other five Rosa species were downloaded to the NCBI database and were used for the subsequent assembly of chloroplast and mitochondrial genomes, with specific information provided in Supplementary Table 1.
4.2 Assembly and annotation of organelle genomes
For the plastome, raw reads were processed to remove adapter sequences and filter out low-quality reads using BBTools (https://sourceforge.net/projects/bbmap/). The filtered data were then assembled into contigs using the GetOrganelle software (Jin et al., 2020). Subsequently, Pilon software (Walker et al., 2014) was employed to polish the assembly with second-generation sequencing data, correcting single nucleotide errors. The resulting genome was annotated using CPGview software (Liu et al., 2023), and manual corrections to the annotations were performed using Geneious software (Kearse et al., 2012). For the mitogenome, we assembled contigs from third-generation sequencing data using PMAT software (Bi et al., 2024). Similarly, Pilon software (Walker et al., 2014) was used to polish the mitogenome assembly, addressing single nucleotide errors. The results were annotated using Geneious software (Kearse et al., 2012), with R. rugosa (NC_065237) as the reference. Finally, the plastomes and mitogenomes were then visualized using OGDraw software (Greiner et al., 2019).
4.3 Comparative analysis of plastomes
We conducted a comparative analysis of the complete plastomes of 7 species within the genus Rosa. The IRscope online tool was utilized to analyze the junctions between the inverted repeat (IR), small single-copy (SSC), and large single-copy (LSC) regions. Sequence variability among the plastomes of the 7 species was assessed using mVISTA with the LAGAN model (Frazer et al., 2004), using R. rugosa as the reference, to illustrate both inter- and intraspecific variations. We extracted the protein-coding genes (PCGs) and intergenic spacers (IGSs) using Geneious software (Kearse et al., 2012), and alignments were performed with MAFFT using default parameters (Katoh and Standley, 2013). The nucleotide diversity of the PCGs and IGSs was analyzed using DnaSP (Librado and Rozas, 2009), employing a sliding window approach with a step size of 200 bp and a window length of 600 bp. CodonW software (Peden, 2000) was used to estimate the codon usage patterns of the PCGs across the plastomes of the 7 Rosa species. The codon usage bias for each species was quantified through relative synonymous codon usage (RSCU) values and effective number of codons (ENC). For visualization of the relationship between ENC and GC3s, refer to Zhang et al (Zhang et al., 2024; Zhang et al., 2025a; Zhang et al., 2025b).
4.4 Calculation of nucleic acid substitution rates
To compare the Ka/Ks values between the plastomes and mitogenomes of 7 Rosa species, we used Malus domestica (plastome: MK434916; mitogenome: NC_018554) as a reference for calculating the Ka/Ks values of PCGs. We extracted the coding sequences (CDS) of 77 chloroplast PCGs and 27 mitochondrial PCGs using Phylosuite software (Zhang et al., 2020). The nucleotide sequences of each gene pair were compared using KaKs_calculator software (Zhang et al., 2006) to compute the paired Ka/Ks ratios for the 77 plastid and 27 mitochondrial PCGs. We also calculated Ka/Ks values for all organelle protein-coding genes in rosa species using the branch model (based on phylogenetic relationships) in PAML software (Yang, 2007). Additionally, to compare differences in evolutionary rates between chloroplast and mitochondrial genes, the Ka/Ks values for individual chloroplast protein-coding genes from the 7 mitogenomes were compared and evaluated using a Mann-Whitney U test in R.
4.5 Comparative mitogenome analysis and gene transfer
For a comparative analysis of the mitogenome structure of 7 Rosa species, regions of covariance between the mitogenomes were identified using Geneious software (Kearse et al., 2012), with ≥80% matches and E-values ≤1e-5. These covariance regions were visualized using the RIdeogram R package (Hao et al., 2020) with default parameters. To identify potential horizontal gene transfers between mitochondria and chloroplasts in 7 Rosa species, we used BLASTN software to identify homologous sequences between the organelles (parameters: identity > 80%; e-value=1e-5), and the shinyCircos package was utilized to visualize intracellular gene transfers (IGT) (Chen et al., 2015; Yu et al., 2018).
4.6 Phylogenomic analysis
A total of 53 species from the genus Rosa and one outgroup species were used for phylogenetic analysis of their plastomes, along with the mitogenomes of 7 Rosa species (Supplementary Table 2). First, we manually corrected apparent annotation errors in the plastomes of the 54 species and the mitogenomes of the 7 species using Geneious software (Kearse et al., 2012). Subsequently, we extracted the coding sequences (CDS) of 78 PCGs from the plastomes and 31 from the mitogenomes using Phylosuite software (Zhang et al., 2020). The CDS sequences were then aligned using MAFFT software (Katoh and Standley, 2013), and gaps as well as highly variable sites were removed with TrimAl software (Capella-Gutiérrez et al., 2009). We then concatenated the CDS sequences of the 78 chloroplast genes and the 31 mitochondrial genes into a supermatrix (CDS_dataset) using FASconCAT-G software (Kück and Longo, 2014), resulting in a supermatrix with 66,764 chloroplast (cp) and 28,492 mitochondrial (mt) sites for subsequent analyses. The species tree was inferred using the maximum likelihood (ML) method in IQ-TREE software (Nguyen et al., 2015), with 1,000 bootstrap replicates. The optimal nucleotide substitution model was selected using ModelFinder (Kalyaanamoorthy et al., 2017). Meanwhile, in order to explore the phylogeny constructed on the basis of chloroplast and mitochondrial data, phylogenetic analyses were performed based on 7 Rosa species for which plastome and mitogenome currently exist. The chloroplast data contained 78 PCGs, the mitochondria contained 31 PCGs, and the models were the optimal alternative models automatically tested and selected by IQ-TREE software (Nguyen et al., 2015). Finally, we visualized the ML tree using ChiPlot (Xie et al., 2023).
The divergence times of Rosa species were estimated using the MCMCTree software package from PAML v4.9j (Yang, 2007). Fossil calibration points were obtained from the Timetree5 website (http://www.timetree.org/) and two individual fossil calibration points were selected: divergence times for the genera Pyrus and Rosa (23.20 to 29.61 Mya), and divergence times for R. pricei and R. taiwanensis (2.78 Mya). Finally, the results were visualized using the ChiPlot online website (Xie et al., 2023).
5 Conclusions
In this study, we assembled and annotated the mitochondrial genomes of six species from the genus Rosa and the plastomes of two species. The chloroplast genome sizes ranged from 156,342 bp (R. laevigata) to 157,214 bp (R. agrestis), with a maximum difference of 872 bp and a minimum difference of 43 bp. The GC content varied from 37.2% to 37.3%, and the number of genes ranged from 129 to 131. The mitochondrial genomes were all circular, with lengths between 271,191 bp and 338,975 bp, containing 52 to 59 genes. Codon usage analysis indicated a preference for A/T-ending codons in both chloroplast and mitochondrial genes. The ENC-map analysis revealed that the protein-coding genes in both chloroplast and mitochondrial genomes fell below the expected ENC curve, suggesting that mutation plays a minor role in shaping codon preferences. The expansion and contraction analysis of the inverted repeat (IR) regions showed higher conservation in the IR regions compared to the large single copy (LSC) and small single copy (SSC) regions. Additionally, we identified four highly differentiated regions (rps19, ndhF, ycf1, and psbM-trnD-GUC) in the plastomes of the 7 Rosa species, which can serve as molecular markers for future species identification and studies of genetic diversity.
In contrast to the chloroplasts, the mitochondrial genomes exhibited more complex collinearity relationships, with structural rearrangements disrupting gene clusters. However, some gene clusters remained conserved within the Rosa species. Furthermore, compared to PCGs of plastome, mitochondrial PCGs displayed a higher non-synonymous to synonymous ratio. This phenomenon may be due to frequent polynucleotide mutations mediated by microinversions mechanism. We also observed extensive gene transfer between the mitochondria and chloroplasts, particularly with the rrn16 and rpl23 genes, which are commonly found in Rosa species. These gene transfer events likely occurred in the ancestor of Rosa around 4.46 Mya. Estimates of divergence events indicate that rapid differentiation among Rosa species took place around 4.46 Mya, potentially influenced by the uplift of the Qinghai-Tibet Plateau during the Late Miocene. This study enriches the genetic resources of the Rosa genus and lays the groundwork for the development of molecular markers, phylogenetic analyses, and research into the evolution of organelle genomes.
Data availability statement
The datasets presented in this study can be found in online repositories. The names of the repository/repositories and accession number(s) can be found in the article/Supplementary Material.
Author contributions
RZ: Formal analysis, Writing – original draft. SL: Data curation, Formal Analysis, Writing – review & editing. YL: Writing – review & editing. PW: Writing – review & editing. NX: Software, Writing – review & editing. YZ: Data curation, Writing – review & editing. XG: Investigation, Writing – review & editing. YY: Investigation, Writing – review & editing. LQ: Supervision, Writing – review & editing. TY: Formal Analysis, Writing – original draft, Writing – review & editing.
Funding
The author(s) declare that financial support was received for the research and/or publication of this article. Thanks to the funded by the Rolling support Project for the provincial University scientific research platform team of the Guizhou Provincial Department of Education [(2022)031].
Acknowledgments
We appreciate Ms. Jiao Chen for her support in life.
Conflict of interest
The authors declare that the research was conducted in the absence of any commercial or financial relationships that could be construed as a potential conflict of interest.
Generative AI statement
The author(s) declare that no Generative AI was used in the creation of this manuscript.
Publisher’s note
All claims expressed in this article are solely those of the authors and do not necessarily represent those of their affiliated organizations, or those of the publisher, the editors and the reviewers. Any product that may be evaluated in this article, or claim that may be made by its manufacturer, is not guaranteed or endorsed by the publisher.
Supplementary material
The Supplementary Material for this article can be found online at: https://www.frontiersin.org/articles/10.3389/fpls.2025.1584289/full#supplementary-material
Supplementary Figure 1 | Comparison of 7 plastomes using R. rugosa annotation as a reference. The vertical scale indicates the percentage of identity, ranging from 50 to 100%. The horizontal axis indicates the coordinates within the plastome. Genome regions are color-coded as exons, introns and conserved non-coding sequences (CNS).
Supplementary Figure 2 | Multinucleotide substitutions and mutation spectra. (a) Examples of multinucleotide mutations (MNMs) in Fragaria species (Fan et al., 2022). (b) MNMs in sdh4 gene of Rosa species. (c) MNMs in nadL4 gene of Rosa species.
Supplementary Figure 3 | Global distribution of the two Rosaspecies in this study.
Supplementary Table 1 | Source of SRA data used in this study.
Supplementary Table 2 | Source of organelle genome files downloaded to the NCBI database in this study.
Supplementary Table 3 | Statistics of RSCU values for PCGs in organelle genome from 7 Rosa species.
Supplementary Table 4 | Summary of GC3s and ENC results for the organelle genomes of 7 Rosa species.
Supplementary Table 5 | Results of a homology comparison between plastome and mitogenome from 7 Rosaspecies.
Supplementary Table 6 | Ka/Ks for the protein-coding genes of the 7 Rosa organelle genomes.
Supplementary Table 7 | Ka/Ks for the protein-coding genes of the 7 Rosa organelle genomes based PAML software.
References
Archibald, J. M. (2011). Origin of eukaryotic cells: 40 years on. Symbiosis 54, 69–86. doi: 10.1007/s13199-011-0129-z
Bi, C., Paterson, A. H., Wang, X., Xu, Y., Wu, D., Qu, Y., et al. (2019). Corrigendum to analysis of the complete mitochondrial genome sequence of the diploid cotton Gossypium raimondii by comparative genomics approaches. BioMed. Res. Int. 2016 (1), 9691253. doi: 10.1155/2019/9691253
Bi, C., Qu, Y., Hou, J., Wu, K., Ye, N., Yin, T. (2022). eciphering the multi-chromosomal mitochondrial genome of Populus simonii D. Front. Plant Sci. 13, 914635. doi: 10.3389/fpls.2022.914635
Bi, C., Shen, F., Han, F. H., Qu, Y. S., Hou, J., Xu, K. W., et al. (2024). PMAT: an efficient plant mitogenome assembly toolkit using low-coverage HiFi sequencing data. Horticulture Res. 11, uhae023. doi: 10.1093/hr/uhae023
Bi, C., Sun, N., Han, F., Xu, K., Yang, Y., Ferguson, D. K.. (2023). The first mitogenome of Lauraceae (Cinnamomum chekiangense)[J. Plant Diversity 46, 144. doi: 10.1016/j.pld.2023.11.001
Bruneau, A., Starr, J. R., Joly, S. (2007). Phylogenetic relationships in the genus Rosa: new evidence from chloroplast DNA sequences and an appraisal of current knowledge. Syst. Bot. 32, 366–378. doi: 10.1600/036364407781179653
Capella-Gutiérrez, S., Silla-Martínez, J. M., Gabaldón, T. (2009). trimAl: a tool for automated alignment trimming in large-scale phylogenetic analyses. Bioinformatics 25, 1972–1973. doi: 10.1093/bioinformatics/btp348
Chen, C., Bai, Y., Fang, X., Guo, H., Meng, Q., Zhang, W., et al. (2019a). A late miocene terrestrial temperature history for the Northeastern Tibetan plateau’s period of tectonic expansion. Geophysical Res. Lett. 46, 8375–8386. doi: 10.1029/2019GL082805
Chen, C., Bai, Y., Fang, X., Guo, H., Meng, Q., Zhang, W., et al. (2019b). A late miocene terrestrial temperature history for the Northeastern Tibetan plateau’s period of tectonic expansion. Geophysical Res. Lett. 46, 8375–8386. doi: 10.1029/2019GL082805
Chen, Y., Ye, W. C., Zhang, Y. D., Xu, Y. (2015). High speed BLASTN: an accelerated MegaBLAST search tool. Nucleic Acids Res. 43, 7762–7768. doi: 10.1093/nar/gkv784
Cheng, Y., He, X.X., Priyadarshani, S. V. G. N., Wang, Y., Ye, L., Shi, C., et al. (2021). Assembly and comparative analysis of the complete mitochondrial genome of. Suaeda glauca. BMC Genomics 22, 167. doi: 10.1186/s12864-021-07490-9
Doyle, J. J., Doyle, J. L. (1987). A rapid DNA isolation procedure for small quantities of fresh leaf tissue. Phytochemical Bull. 19. doi: 10.1016/0031-9422(80)85004-7
Fan, W., Liu, F., Jia, Q., Du, H., Chen, W., Ruan, J., et al. (2022). Fragaria mitogenomes evolve rapidly in structure but slowly in sequence and incur frequent multinucleotide mutations mediated by microinversions. New Phytol. 236, 745–759. doi: 10.1111/nph.v236.2
Fougere-Danezan, M., Joly, S., Bruneau, A., Gao, X. F., Zhang, L. B. (2015). Phylogeny and biogeography of wild roses with specific attention to polyploids. Ann. Bot. 115, 275–291. doi: 10.1093/aob/mcu245
Frazer, K. A., Pachter, L., Poliakov, A., Rubin, E. M., Dubchak, I. (2004). VISTA: computational tools for comparative genomics. Nucleic Acids Res. 32, W273–W279. doi: 10.1093/nar/gkh458
Gahlaut, V., Kumari, P., Jaiswal, V., Kumar, S. (2021). Genetics, genomics and breeding in Rosaspecies. J. Hortic. Sci. Biotechnol. 96, 545–559. doi: 10.1080/14620316.2021.1894078
Greiner, S., Lehwark, P., Bock, R. (2019). OrganellarGenomeDRAW (OGDRAW) version 1.3.1: expanded toolkit for the graphical visualization of organellar genomes. Nucleic Acids Res. 47, W59–W64. doi: 10.1093/nar/gkz238
Guo, X., Wang, F., Fang, D., Lin, Q., Sahu, S. K., Luo, L., et al. (2023). The genome ofAcorus deciphers insights into early monocot evolution. Nat. Commun. 14, 3662. doi: 10.1038/s41467-023-38836-4
Han, F., Qu, Y., Chen, Y., Xu, L., Bi, C. (2022). Assembly and comparative analysis of the complete mitochondrial genome of Salix wilsonii using PacBio HiFi sequencing. Front. Plant Sci. 13, 1031769. doi: 10.3389/fpls.2022.1031769
Hao, Z., Lv, D., Ge, Y., Shi, J., Weijers, D., Yu, G., et al. (2020). RIdeogram: drawing SVG graphics to visualize and map genome-wide data on the idiograms. PeerJ Comput. Sci. 6, e251. doi: 10.7717/peerj-cs.251
He, X., Qian, Z., Gichira, A. W., Chen, J., Li, Z. (2024). Assembly and comparative analysis of the first complete mitochondrial genome of the invasive water hyacinth. Eichhornia crassipes. Gene 914, 148416. doi: 10.1016/j.gene.2024.148416
Herbert, T. D., Lawrence, K. T., Tzanova, A., Peterson, L. C., Caballero-Gill, R., Kelly, C. S. (2016a). Late Miocene global cooling and the rise of modern ecosystems. Nat. Geosci. 9, 843–847. doi: 10.1038/ngeo2813
Herbert, T. D., Lawrence, K. T., Tzanova, A., Peterson, L. C., Caballero-Gill, R., Kelly, C. S. (2016b). Late Miocene global cooling and the rise of modern ecosystems. Nat. Geosci. 9, 843–847. doi: 10.1038/ngeo2813
Hikosaka, K., Watanabe, Y.-i., Tsuji, N., Kita, K., Kishine, H., Arisue, N., et al. (2010). Divergence of the mitochondrial genome structure in the Apicomplexan Parasites, Babesia and Theileria. Mol. Biol. Evol. 27, 1107–1116. doi: 10.1093/molbev/msp320
Huang, J. L. (2003). Genetic study on Jin a cytoplasmic male sterile linein cotton (Gossypium). Shanxi Agric. Univ. Available online at: https://kns.cnki.net/kcms2/article/abstract?v=EKYfHJ8l29h0qPv5qqMKXnN4EMYSGnb43L1OWcnY53UQRfc8CwuCL3rLcjR4gB-0sM_0SdY8f8FLcz1s9kUHVMeOFaIoZgafIGPdIiZqOz1_BOQw5FgKD_JXeM_SXptG147z36bnfbStTMaIT4N7F2Bvl65ddh-6VBIdHxPH6CdqNHBjyVzLhg==&uniplatform=NZKPT&language=CHS.
Jeon, J. H., Kim, S. C. (2019). Comparative a nalysis of the complete chloroplast genome sequences of three closely related East-Asian wild Roses (Rosasect. Synstylae; Rosaceae). Genes 10 (1), 23. doi: 10.3390/genes10010023
Jin, J. J., Yu, W. B., Yang, J. B., Song, Y., dePamphilis, C. W., Yi, T.-S., et al. (2020). GetOrganelle: a fast and versatile toolkit for accurate de novo assembly of organelle genomes. Genome Biol. 21, 241. doi: 10.1186/s13059-020-02154-5
Joichi, A., Yomogida, K., Awano, K., Ueda, Y. (2005). Volatile components of tea-scented modern roses and ancient Chinese roses. Flavour Fragrance J. 20, 152–157. doi: 10.1002/ffj.1388
Kalyaanamoorthy, S., Minh, B. Q., Wong, T. K. F., von Haeseler, A., Jermiin, L. S. (2017). ModelFinder: fast model selection for accurate phylogenetic estimates. Nat. Methods 14, 587–589. doi: 10.1038/nmeth.4285
Katoh, K., Standley, D. M. (2013). MAFFT multiple sequence alignment software version 7: improvements in performance and usability. Mol. Biol. Evol. 30, 772–780. doi: 10.1093/molbev/mst010
Kearse, M., Moir, R., Wilson, A., Stones-Havas, S., Cheung, M., Sturrock, S., et al. (2012). Geneious Basic: An integrated and extendable desktop software platform for the organization and analysis of sequence data. Bioinformatics 28, 1647–1649. doi: 10.1093/bioinformatics/bts199
Kück, P., Longo, G. C. (2014). FASconCAT-G: extensive functions for multiple sequence alignment preparations concerning phylogenetic studies. Front. Zoology 11, 81. doi: 10.1186/s12983-014-0081-x
Lemos, R. S., Fernandes, A. S., Pereira, M. M., Gomes, C. M., Teixeira, M. (2002). Quinol:fumarate oxidoreductases and succinate:quinone oxidoreductases: phylogenetic relationships, metal centres and membrane attachment. Biochim. Biophys. Acta (BBA) - Bioenergetics 1553, 158–170. doi: 10.1016/S0005-2728(01)00239-0
Librado, P., Rozas, J. (2009). DnaSP v5: a software for comprehensive analysis of DNA polymorphism data. Bioinformatics 25, 1451–1452. doi: 10.1093/bioinformatics/btp187
Lin, W., Huang, J., Xue, M., Wang, X., Wang, C. (2019). Characterization of the complete chloroplast genome of Chinese roseRosachinensis (Rosaceae: rosa). Mitochondrial DNA Part B 4, 2984–2985. doi: 10.1080/23802359.2019.1664350
Liu, S. Y., Ni, Y., Li, J. L., Zhang, X. Y., Yang, H. Y., Chen, H. M., et al. (2023). CPGView: A package for visualizing detailed chloroplast genome structures. Mol. Ecol. Resour. 23, 694–704. doi: 10.1111/1755-0998.13729
Meng, J., Li, X., Li, H., Yang, J., Wang, H., He, J. (2018). Comparative analysis of the complete chloroplast genomes of four. Aconitum medicinal species. Molecules 23. doi: 10.3390/molecules23051015
Moin, M., Bakshi, A., Madhav, M. S., Kirti, P. B. (2017). Expression profiling of ribosomal protein gene family in dehydration stress responses and characterization of transgenic Rice plants overexpressing RPL23A for water-use efficiency and tolerance to drought and salt stresses. Front. Chem. 5, 97. doi: 10.3389/fchem.2017.00097
Mwanzia, V. M., He, D. X., Gichira, A. W., Li, Y., Ngarega, B. K., Karichu, M. J., et al. (2020). The complete plastome sequences of five Aponogeton species (Aponogetonaceae): Insights into the structural organization and mutational hotspots. Plant Diversity 42, 334–342. doi: 10.1016/j.pld.2020.02.002
Nguyen, L.-T., Schmidt, H. A., von Haeseler, A., Minh, B. Q. (2015). IQ-TREE: a fast and effective stochastic algorithm for estimating Maximum-Likelihood phylogenies. Mol. Biol. Evol. 32, 268–274. doi: 10.1093/molbev/msu300
Niu, Y., Luo, Y., Wang, C., Xu, Q., Liao, W. (2022). The chloroplast genome of Rosarugosa × Rosasertata (Rosaceae): genome structure and comparative analysis. Genet. Mol. Biol. 45 (3), e20210319. doi: 10.1590/1678-4685-GMB-2021-0319
Notsu, Y., Masood, S., Nishikawa, T., Kubo, N., Akiduki, G., Nakazono, M., et al. (2002). The complete sequence of the rice (Oryza sativa L.) mitochondrial genome: frequent DNA sequence acquisition and loss during the evolution of flowering plants. Mol. Genet. Genomics 268, 434–445. doi: 10.1007/s00438-002-0767-1
Park, J., Xi, H., Kim, Y., Nam, S., Heo, K.-I. (2020). The complete mitochondrial genome of new species candidate of Rosarugosa (Rosaceae). Mitochondrial DNA Part B 5, 3435–3437. doi: 10.1080/23802359.2020.1821820
Potter, D., Eriksson, T., Evans, R. C., Oh, S., Smedmark, J. E. E., Morgan, D. R., et al. (2007). Phylogeny and classification of. Rosaceae. Plant Systematics Evol. 266, 5–43. doi: 10.1007/s00606-007-0539-9
Rao, M. V., Sharma, P. S. N. (2001). Protective effect of vitamin E against mercuric chloride reproductive toxicity in male mice. Reprod. Toxicol. 15, 705–712. doi: 10.1016/S0890-6238(01)00183-6
Raymond, O., Gouzy, J., Just, J., Badouin, H., Verdenaud, M., Lemainque, A., et al. (2018). The Rosagenome provides new insights into the domestication of modern roses. Nat. Genet. 50, 772–777. doi: 10.1038/s41588-018-0110-3
Ruiz-Pesini, E., Alvarez, E., Enríquez, J. A., López-Pérez, M. J. (2001). Association between seminal plasma carnitine and sperm mitochondrial enzymatic activities. Int. J. Andrology 24, 335–340. doi: 10.1111/j.1365-2605.2001.00311.x
Shen, W.X., Dong, Z.H., Zhao, W.Z., Ma, L.Y., Wang, F., Li, W.Y., et al. (2022). Complete chloroplast genome sequence of Rosalucieae and its characteristics. Horticulturae 8 (9), 788. doi: 10.3390/horticulturae8090788
Sloan, D. B., Alverson, A. J., Chuckalovcak, J. P., Wu, M., McCauley, D. E., Palmer, J. D., et al. (2012). Rapid evolution of enormous, multichromosomal genomes in flowering plant mitochondria with exceptionally high mutation rates. PloS Biol. 10, e1001241. doi: 10.1371/journal.pbio.1001241
Smith, D. R., Keeling, P. (2015). Mitochondrial and plastid genome architecture: Reoccurring themes, but significant differences at the extremes. Proc. Natl. Acad. Sci. United States America 112, 10177–10184. doi: 10.1073/pnas.1422049112
Soucy, S. M., Huang, J., Gogarten, J. P. (2015). Horizontal gene transfer: building the web of life. Nat. Rev. Genet. 16, 472–482. doi: 10.1038/nrg3962
Su, Y.W., Zhao, S.Y., Zhang, Y.J., Zhou, T.D. (1996). Enzyme cytochemistry in microsporogenesis of male sterile lines of Luo Bu (Raphanus sativus L. J. Shandong Univ. (Natural Sci. Edition) 3, 341–346. Available online at: https://kns.cnki.net/kcms2/article/abstract?v=EKYfHJ8l29ilTrco9LZSvWvgrKWO839DN6rs6zT-2R3XsjAoY1hcVUNgEqJrhXSgjcNh8vCiqbVgfgkFHOTwG-KisiCAp5NzqZk8Y4H_nhDYtPvpwPLfjiHnZx5ihNZQslqseiigic7t-HHmxd9JwcmfSuqDLeFpUDgSsl08fOs=&uniplatform=NZKPT&language=CHS.
Sun, N., Han, F., Wang, S., Shen, F., Liu, W., Fan, W., et al. (2024). Comprehensive analysis of the Lycopodium japonicum mitogenome reveals abundant tRNA genes and cis-spliced introns in Lycopodiaceae species. Front. Plant Sci. 15. doi: 10.3389/fpls.2024
Sun, Z., Shi, S., Pang, X., Chen, J., Wu, Y. (2020). Characterization of the complete chloroplast genome of Rosalaevigata var. leiocarpus in China and phylogenetic relationships. Mitochondrial DNA Part B 5, 1996–1997. doi: 10.1080/23802359.2020.1757526
Tzanova, A., Herbert, T. D., Peterson, L. (2015). Cooling Mediterranean Sea surface temperatures during the Late Miocene provide a climate context for evolutionary transitions in Africa and Eurasia. Earth Planetary Sci. Lett. 419, 71–80. doi: 10.1016/j.epsl.2015.03.016
Walker, B. J., Abeel, T., Shea, T., Priest, M., Abouelliel, A., Sakthikumar, S., et al. (2014). Pilon: an integrated tool for comprehensive microbial variant detection and genome assembly improvement. PloS One 9, e112963. doi: 10.1371/journal.pone.0112963
Wang, J., He, W. C., Xiang, K. L., Wu, Z. Q., Gu, C. H. (2023). Advances in plant phylogeny in the genome era. J. Zhejiang Agric. Forestry Univ. 40, 227–236.
Wang, J., Kan, S., Liao, X., Zhou, J., Tembrock, L. R., Daniell, H., et al. (2024a). Plant organellar genomes: much done, much more to do. Trends Plant Sci. 29, 754–769. doi: 10.1016/j.tplants.2023.12.014
Wang, L., Liu, X., Xu, Y., Zhang, Z., Wei, Y., Hu, Y., et al. (2024). Assembly and comparative analysis of the first complete mitochondrial genome of a traditional Chinese medicine Angelica biserrata (Shan et Yuan) Yuan et Shan. Int. J. Biol. Macromolecules 257, 128571. doi: 10.1016/j.ijbiomac.2023.128571
Wang, S., Qiu, J., Sun, N., Han, F., Wang, Z. (2025). Characterization and comparative analysis of the first mitochondrial genome of Michelia (Magnoliaceae). Genomics Commun. 2, e001. doi: 10.48130/gcomm-0025-0001
Wang, Y., Sun, N., Shi, W., Ma, Q., Sun, L., Hao, M., et al. (2023). Assembly and comparative analysis of the complete mitochondrial genome of ilex macrocarpa. Forests 14, 2372. doi: 10.3390/f14122372
Wang, J., Zou, Y., Mower, J. P., Reeve, W., Wu, Z. (2024b). Rethinking the mutation hypotheses of plant organellar DNA. Genomics Commun. 1, e003. doi: 10.48130/gcomm-0024-0003
Wu, Z., Waneka, G., Sloan, D. B. (2020). The tempo and mode of angiosperm mitochondrial genome divergence inferred from intraspecific variation in Arabidopsis thaliana. G3 Genes|Genomes|Genetics 10, 1077–1086. doi: 10.1534/g3.119.401023
Xia, C., Wang, M., Guan, Y., Li, J. (2022). Comparative analysis of the chloroplast genome for Aconitum species: genome structure and phylogenetic relationships. Front Genet. 13, 878182. doi: 10.3389/fgene.2022.878182
Xie, J., Chen, Y., Cai, G., Cai, R., Hu, Z., Wang, H. (2023). Tree Visualization By One Table (tvBOT): a web application for visualizing, modifying and annotating phylogenetic trees. Nucleic Acids Res. 51, W587–W592. doi: 10.1093/nar/gkad359
Xu, S.J., Teng, K., Zhang, H., Wu, J.Y., Duan, L.S., Zhang, H.Y., et al. (2023). The first complete mitochondrial genome of Carex (C. breviculmis): a significantly expanded genome with highly structural variations. Planta 258, 43. doi: 10.1007/s00425-023-04169-1
Yang, Z. (2007). PAML 4: phylogenetic analysis by maximum likelihood. Mol. Biol. Evol. 24, 1586–1591. doi: 10.1093/molbev/msm088
Yang, J. B., Li, D. Z., Li, H. T. (2014). Highly effective sequencing whole chloroplast genomes of angiosperms by nine novel universal primer pairs. Mol. Ecol. Resour. 14, 1024–1031. doi: 10.1111/1755-0998.12251
Yu, Y. M., Ouyang, Y. D., Yao, W. (2018). shinyCircos: an R/Shiny application for interactive creation of Circos plot. Bioinformatics 34, 1229–1231. doi: 10.1093/bioinformatics/btx763
Yuan, J. Y. (2005). Study on cytomorphology and characteristics of physiology and biochemistry in the new CMS Line of non-heading Chinese cabbage (Nanjing Agricultural University).
Yue, J.P., Hu, X.Y., Sun, H., Yang, Y.P., Huang, J.L. (2012). Widespread impact of horizontal gene transfer on plant colonization of land. Nat. Commun. 3, 1152. doi: 10.1038/ncomms2148
Zhang, D., Gao, F., Jakovlić, I., Zou, H., Zhang, J., Li, W. X., et al. (2020). PhyloSuite: An integrated and scalable desktop platform for streamlined molecular sequence data management and evolutionary phylogenetics studies. Mol. Ecol. Resour. 20, 348–355. doi: 10.1111/1755-0998.13096
Zhang, Z., Li, J., Zhao, X. Q., Wang, J., Wong, G. K., Yu, J. (2006). KaKs_Calculator: calculating Ka and Ks through model selection and model averaging. Genomics Proteomics Bioinf. 4, 259–263. doi: 10.1016/S1672-0229(07)60007-2
Zhang, R., Liu, Y., Liu, S., Zhao, Y., Xiang, N., Gao, X., et al. (2025a). Comparative organelle genomics in Daphniphyllaceae reveal phylogenetic position and organelle structure evolution. BMC Genomics 26, 40. doi: 10.1186/s12864-025-11213-9
Zhang, R., Xiang, N., Gao, X., Zhang, G., Lu, T., Yuan, T. (2025b). Molecular phylogenetic relationships based on mitogenomes of spider: insights into evolution and adaptation to extreme environments. Ecol. Evolution. 15, e70774. doi: 10.1002/ece3.70774
Zhang, R., Xiang, N., Qian, C., Liu, S., Zhao, Y., Zhang, G., et al. (2024). Comparative analysis of the organelle genomes of Aconitum carmichaelii revealed structural and sequence differences and phylogenetic relationships. BMC Genomics 25, 260. doi: 10.1186/s12864-024-10136-1
Zhu, Z.M., Gao, X.F. (2015). Molecular evidence for the hybrid origin of Rosalichiangensis (Rosaceae). Phytotaxa 222, 221–228. doi: 10.11646/phytotaxa.222.3.5
Keywords: Rosa, organelle, phylogeny, structural, adaptation
Citation: Zhang R, Liu S, Liu Y, Wei P, Xiang N, Zhao Y, Gao X, Yin Y, Qin L and Yuan T (2025) Comparative analysis of the organelle genomes of seven Rosa species (Rosaceae): insights into structural variation and phylogenetic position. Front. Plant Sci. 16:1584289. doi: 10.3389/fpls.2025.1584289
Received: 27 February 2025; Accepted: 16 April 2025;
Published: 08 May 2025.
Edited by:
Changwei Bi, Nanjing Forestry University, ChinaCopyright © 2025 Zhang, Liu, Liu, Wei, Xiang, Zhao, Gao, Yin, Qin and Yuan. This is an open-access article distributed under the terms of the Creative Commons Attribution License (CC BY). The use, distribution or reproduction in other forums is permitted, provided the original author(s) and the copyright owner(s) are credited and that the original publication in this journal is cited, in accordance with accepted academic practice. No use, distribution or reproduction is permitted which does not comply with these terms.
*Correspondence: Tao Yuan, eXVhbnRhb3N3QDE2My5jb20=
†These authors have contributed equally to this work