- 1Biology Department, Faculty of Science at Yanbu, Taibah University, Yanbu El-Bahr, Saudi Arabia
- 2Botany and Microbiology Department, Faculty of Science, University of Cairo, Giza, Egypt
- 3Central Lab of Organic Agriculture, Agricultural Research Center (ARC), Giza, Egypt
- 4Department of Biology, College of Science, Princess Nourah bint Abdulrahman University, Riyadh, Saudi Arabia
- 5Microbiology and Immunology Unit, Natural and Health Sciences Research Center, Princess Nourah Bint Abdulrahman University, Riyadh, Saudi Arabia
- 6Environmental Health Sciences, Mailman School of Public Health, Columbia University, New York, NY, United States
- 7Environmental and Biomaterial Unit, Natural and Health Sciences Research Center, Princess Nourah Bint Abdulrahman University, Riyadh, Saudi Arabia
- 8Biology Department, Faculty of Science, Taibah University, Al-Madinah Al-Munawarah, Saudi Arabia
- 9Department of Botany and Microbiology, Faculty of Science, Beni-Suef University, Beni-Suef, Egypt
Introduction: Neodymium (Nd), a rare earth element (REEs), is widely utilized in industry. Although the detailed biological role of Nd in plant biology is unclear, recent reports have noted its oxidative phytotoxicity at concentrations higher than 200 mg kg-1 soil. At present it is unclear if these detrimental effects could be offset by the global rise in atmospheric carbon dioxide concentration ([CO2]) which has been shown to enhance photosynthesis and growth in a wide range of C3 plant species.
Methods: To assess any amelioration effects of [CO2], a phytotoxic dose of Nd (III) was given to wheat grown under two scenarios of atmospheric CO2, ambient levels of CO2 (aCO2, 420 ppm) and eCO2 (620 ppm) to assess growth and photosynthesis.
Results and discussion: Our results suggest that at ambient [CO2], Nd treatment retarded wheat growth, photosynthesis and induced severe oxidative stress. In contrast, eCO2 reduced the accumulation of Nd in wheat tissues and mitigated its negative impact on biomass production and photosynthesis related parameters, i.e., photosynthetic rate, chlorophyll content, Rubisco activity and photochemical efficiency of PSII (Fv/Fm). Elevated [CO2] also supported the antioxidant defense system in Nd-treated wheat, enhanced production of enzymatic antioxidants, and more efficient ascorbate-glutathione recycling was noted. While additional data are needed, these initial results suggest that rising [CO2] could reduce Nd-induced oxidative stress in wheat.
1 Introduction
Extensive human activities are slowly increasing atmospheric carbon dioxide concentration [CO2] in the atmosphere (Nunes, 2023). In addition to its role as a greenhouse gas, [CO2] is the primary source of carbon for plant photosynthesis and its increase has been associated with improved growth and fecundity for a number of C3 plant species, including crops (AbdElgawad et al., 2022b, 2023; Ziska, 2022).
Although plant response to [CO2] per se is acknowledged as beneficial, interactions with other abiotic parameters, including heavy metals, is still unclear. Work on arsenic in rice has suggested that elevated [CO2] may increase arsenic concentration in the rice grain (Wang et al., 2023); conversely, elevated [CO2] attenuated the toxicity of NiO-nanoparticles on wheat (Saleh et al., 2019); arsenic oxide nanoparticles on Zea mays (Selim et al., 2021); and silicon dioxide nanoparticles on pea (Shabbaj et al., 2021).
Rare earth elements (REEs), which include scandium, yttrium, and the 15 lanthanides, possess distinctive properties that make them highly valuable across various industries and products. REEs are integral to high-tech devices such as rechargeable batteries, computers, mobile phones, medical imaging equipment, LCD screens, and radar systems (Hu et al., 2016). Additionally, in agriculture, these elements are used in small concentrations as fertilizers; however, elevated levels can negatively impact edible horticultural crops, leading to environmental and human health risks. The bioaccumulation of REEs has been shown to adversely affect aquatic organisms (Revel et al., 2025), as well as terrestrial life, including plants, animals, and humans (Wang et al., 2024; Petrini et al., 2025). Therefore, it is important to investigate the potential hazards associated with REEs accumulation under different environmental conditions. Understanding these dynamics is critical for promoting safe and responsible use of these elements in various applications.
The rising levels of atmospheric [CO2] may enhance plant growth and resilience, potentially counteracting the adverse effects of rare earth elements (REEs) however, its role to compensate, or exacerbate, any REE in plant effects has not been evaluated. Neodymium (Nd) is a lanthanide and falls within the category of rare earth elements (REEs). Available reports indicate that Nd concentrations in uncontaminated soils can range from 5.8 to 53 mg kg-1 (Carpenter et al., 2015; Patra et al., 2020; Wang et al., 2020). Although its biological role of Nd is still obscure, phytotoxicity of Nd has been reported (Zhao et al., 2019). The phytotoxicity of a high Nd dose (319.00 mg kg-1) on plant growth was stronger compared to other REEs, i.e., Sm, Tb, Dy and Er (Carpenter et al., 2015). Therefore, more investigations are needed to address Nd effect on some important crops in the human nutrition such as wheat (Triticum aestivum) which has been chosen for the current study.
Wheat is a crucial staple in the human diet, particularly in developing countries (Rizvi et al., 2020). While there is established evidence regarding the negative effects of heavy metals such as bismuth, cadmium, copper, and zinc on various biological processes in wheat (Mohammed et al., 2023, Athar and Ahmad, 2002; Hammami et al., 2022), the interaction between rising atmospheric CO2 levels and heavy metal contamination remains inconsistent. For instance, a Free-Air CO2 Enrichment (FACE) study found that elevated CO2 levels resulted in reduced copper (Cu) concentrations but increased cadmium (Cd) concentrations in both the shoots and grains of wheat and rice grown in contaminated soils (Guo et al., 2011).
While detailed investigations on neodymium (Nd) exposure in relation to elevated [CO2] per se are currently limited, this study aims to fill that gap. Therefore, the objectives currently were to assess the redox status of wheat under Nd stress, both with and without supplemental CO2, and to examine the impact on key physiological processes, including photosynthesis. To evaluate the accumulation of Nd in plant tissues, along with key growth parameters and photosynthetic efficiency. To monitor changes in wheat stress markers and analyze the activity of molecular and enzymatic reactive oxygen species (ROS) scavengers, as well as the components of the ascorbate-glutathione cycle. Through this comprehensive assessment, authors aimed to deepen our understanding of how elevated CO2 can influence wheat’s response to Nd exposure, providing valuable insights into both plant physiology and environmental interactions.
2 Methods
2.1 Plant growth and treatments
Wheat (Triticum aestivum L., cv Giza 112) grains were surface sterilized using sodium hypochlorite (5% v/v) for 20 minutes before being seeded in pots with an artificial soil that were 15 cm deep and 13 cm in diameter. For 1 g of air-dried soil, the following was the soil composition: carbon (11.7 mg), ammonium N (1.1 mg), nitrate nitrogen (14.8 mg), phosphorus (9.4 mg), and humidity (0.33 g water). Four different set-ups were used to arrange the plants: 1) ambient CO2 (aCO2, 420 ppm CO2; 2) 200 mg Nd3+kg-1 soil under aCO2, (Nd); 3) elevated CO2 (eCO2, 620 ppm) and 4) 200 mg Nd3+kg-1 soil under eCO2, (Nd+eCO2). A preliminary experiment determined the Nd (III) concentration, and the eCO2 level was determined using the IPCC-SRES B2-state estimate of eCO2 for the year 2100 (Murray and Ebi, 2012). Nd was applied in the form of NdCl3.6H2O. Pots were kept in a growth chamber at a temperature of 21/18°C with a photoperiod of 16/8h (photosynthetically active radiation of 350 µmol photons m-2 s-1) and 60% humidity. In the growth room, the pots were placed at random and regularly watered. The experiment was repeated, but this time the two CO2 levels in the cabinets were switched. The plants were collected four weeks after the seeds were sown, the shoots and roots’ fresh and dried weights were measured. The plant material was transported in liquid N and stored at -80°C. In order to conduct further research, soil samples were collected and stored at -20°C in an ice box. Five replicates of each treatment (pot) were performed.
2.2 Photosynthesis related parameters
According to (Abdelgawad et al., 2015), light-saturated photosynthetic rate (Asat) and stomatal conductance (gs) were measured from the youngest-fully enlarged leaf using the LI-COR LI-6400, a device made by LI-COR Inc. in Lincoln, Nebraska, USA. Photosynthesis was evaluated at light intensity of 1,500 μmol m−2 s−1. The CO2 concentration in the leaf chamber was maintained at 420 µmol mol–1 for the aCO2 group and 620 µmol mol–1 for the eCO2 group, with the temperature controlled at 25 ± 0.5°C. photosynthetic pigment concentration was extracted in acetone and quantified according to Porra et al. (1989). Using a fluorimeter (PAM2000, Walz, Effeltrich, Germany) over a 30-minute period, chlorophyll fluorescence was calculated from the dark-adapted leaves.
2.3 Determination of neodymium accumulation
The dried plant samples were treated in 13 M nitric acid at 185°C for 25 minutes for Nd extraction (Agusa et al., 2005). Inductively coupled plasma mass spectrometry (ICP-MS; model 820-MS) with a glass nebulizer operating at 0.4 mL/minute was being used to determine the element concentration. For calibration curves, external standards with concentrations ranging from 1 to 600 g/L were created. Yttrium was also introduced to regulate nebulizer efficacy as an internal standard during extraction. In 0.23 M nitric acid, standard minerals were created.
2.4 Oxidative stress markers
Malondialdehyde (MDA) was identified in plant samples using the thiobarbituric acid method as a marker for lipid peroxidation (Hodges et al., 1999). Xylenol orange method was used for determining H2O2 in plant samples after extraction in 0.1% trichloroacetic acid (Jiang et al., 1990).
2.5 Total antioxidant capacity and antioxidant metabolites
Plant materials that were extracted in 2 mL of 80% ice-cold ethanol by a MagNALyser were ground using liquid-N2. The ferric reducing/antioxidant power (FRAP) assay was used to calculate the total antioxidant capacity (TAC) of the extracts and was performed at 600 nm on a microplate reader, using Trolox as a standard (Benzie and Strain, 1999). HPLC analysis was used to evaluate the levels of reduced ascorbate (ASC) and reduced glutathione (GSH) (Potters et al., 2004). The total ascorbate (ASC+DHA) and glutathione (GSH+GSSG) concentration was assessed after reduction by DTT. Phenolic compounds were extracted from plant samples with 80% ethanol. After that, measurements of polyphenols (Zhang et al., 2006) and flavonoids (Chang et al., 2002) were performed using a spectrophotometer (Shimadzu UV-Vis 1601 PC, Japan). After hexane extraction, extracts were collected (CentriVap concentrator, Labconco, Kansas, USA; normal phase conditions, Particil Pac 5 m column material, length 250 mm, i.d. 4.6 mm) for tocopherols quantification by HPLC (Shimadzu, Hertogenbosch, the Netherlands; normal phase conditions). Dimethyl tocol was used as an internal standard at 5 ppm.
2.6 Antioxidant enzymes and glutathione-S-transferase
For the extraction of antioxidant enzymes, MagNALyser (Roche, Vilvoorde, Belgium) was used along with Triton X-100 (0.25%, v/v), polyvinyl pyrrolidone (10%, w/v), ASC (1 mM) and phenylmethylsulfonyl fluoride (1 mM) in a potassium phosphate buffer (50 mM, pH 7.0). After centrifugation (10 minutes, 13000 rpm, 4°C) the supernatant was obtained and the activities of catalase (CAT, EC 1.11.1.6), peroxidase (POX, EC 1.11.1), superoxide dismutase (SOD, EC 1.15.1.1), ascorbate peroxidase (APX, EC 1.11.1.11), glutathione peroxidase (GPX, EC 1.11.1.9), glutathione reductase (GR, EC 1.6.4.2), dehydroascorbate reductase (DHAR, EC 1.8.5.1) and monodehydroascorbate reductase (MDHAR, EC 1.6.5.4) were measured. The inhibition of nitroblue tetrazolium reduction at 560 nm was used to measure the activity of SOD (Dhindsa et al., 1982). The method recommended by Kumar and Khan (1982) was employed to evaluate the pyrogallol oxidation in order to determine POX activity. According to Aebi (1984), the dissociation of H2O2 at 240 nm was used to test the activity of CAT. The measurements of APX, DHAR, MDHAR, and GR activity followed the guidelines provided by Murshed et al. (2008). The NADPH oxidation decrement at 340 nm as published by (Drotar et al., 1985) was used to estimate the activity of GPX. Glutathione-S-transferase (GST, EC 2.5.1.18) was evaluated using potassium phosphate buffer (50 mM, pH 7.0) (Diopan et al., 2008). The method described by Lowry et al. (1951) was employed to determine the amounts of soluble proteins in the extracts.
2.7 Statistical analyses
Experiments were carried out using a completely randomized block design. The Statistical Analysis System (SPSS Inc., Chicago, IL, USA) was used to analyze the data. The Kolmogorov-Smirnov and Levene’s tests were used to determine data normality and variance homogeneity. The data were all subjected to Two-way analysis of variance (ANOVA). Tukey’s Test (p = 0.05) was used as a post hoc test for mean separation. Each experiment was replicated five times (n = 5).
3 Results
3.1 Growth and photosynthesis
Neodymium treatment significantly decreased the FW and DW of wheat plants by 57 and 60%, respectively, relative to the control (Table 1). In contrast, eCO2 alone showed a fertilization effect on wheat plants, significantly improving biomass production, by 43 and 40% for FW and DW, respectively (Table 1). The combined treatment (Nd+eCO2) showed a moderate increase in FW and DW relative to Nd alone, but significantly less than that of the elevated [CO2] treatment (Table 1). In parallel with FW and DW, the rate of photosynthesis in wheat plants treated with Nd alone declined sharply (- 44%) relative to the control (Table 1). Such reduction was concomitant with a significant decrease in chlorophyll content, chlorophyll fluorescence, stomatal conductance and Rubisco activity. On the other hand, eCO2 alone resulted in a significant improvement in photosynthetic rate, chlorophyll content and chlorophyll fluorescence at 24, 29 and 7.5% respectively, but had no significant effect on stomatal conductance or Rubisco activity compared to control (Table 1). The co-application of eCO2 on Nd-stressed plants significantly enhanced the rate of photosynthesis, chlorophyll content, Rubisco activity and photochemical efficiency of PSII (Fv/Fm) relative to Nd alone. A two Way ANOVA indicated significant interaction of Nd and eCO2 in the FW, DW, Photosynthesis, GS and the pigments except CHLa (Supplementary Data Sheet S1).
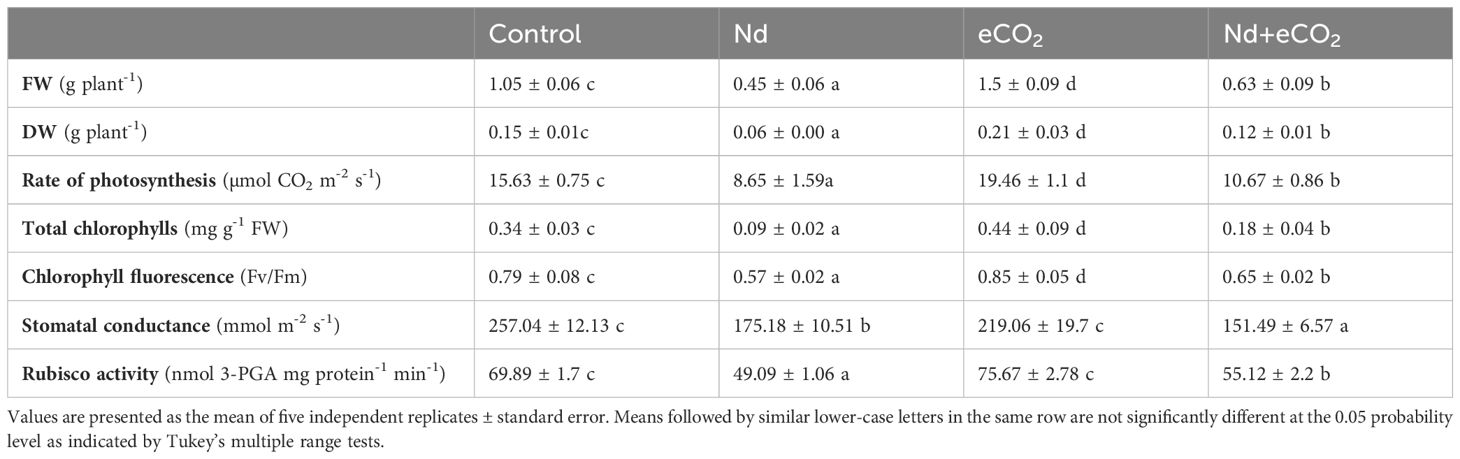
Table 1. Effect of neodymium (Nd), elevated CO2 (eCO2) and their synchronous application (Nd + eCO2) on the fresh (FW) and dry (DW) biomasses and the photosynthesis related parameters in wheat plants.
3.2 Accumulation of Nd and stress markers
As expected, application of Nd sharply increased the accumulation of Nd in plant shoots (Figure 1A); however, Nd accumulation was significantly reduced (~50%) at elevated [CO2]. Nd treatment induced about 3-fold accumulation of H2O2 and a two-fold increase in the level of MDA (Figures 1B, C). Elevated [CO2] per se had no significant impact on H2O2 and MDA levels compared to the control. Interestingly, the synchronous application of eCO2 with Nd significantly reduced the production of H2O2 and MDA by about 50 and 22%, respectively, compared to Nd treatment alone. A significant interaction effect was noted for H2O2 and MDA (Supplementary Data Sheet S1).
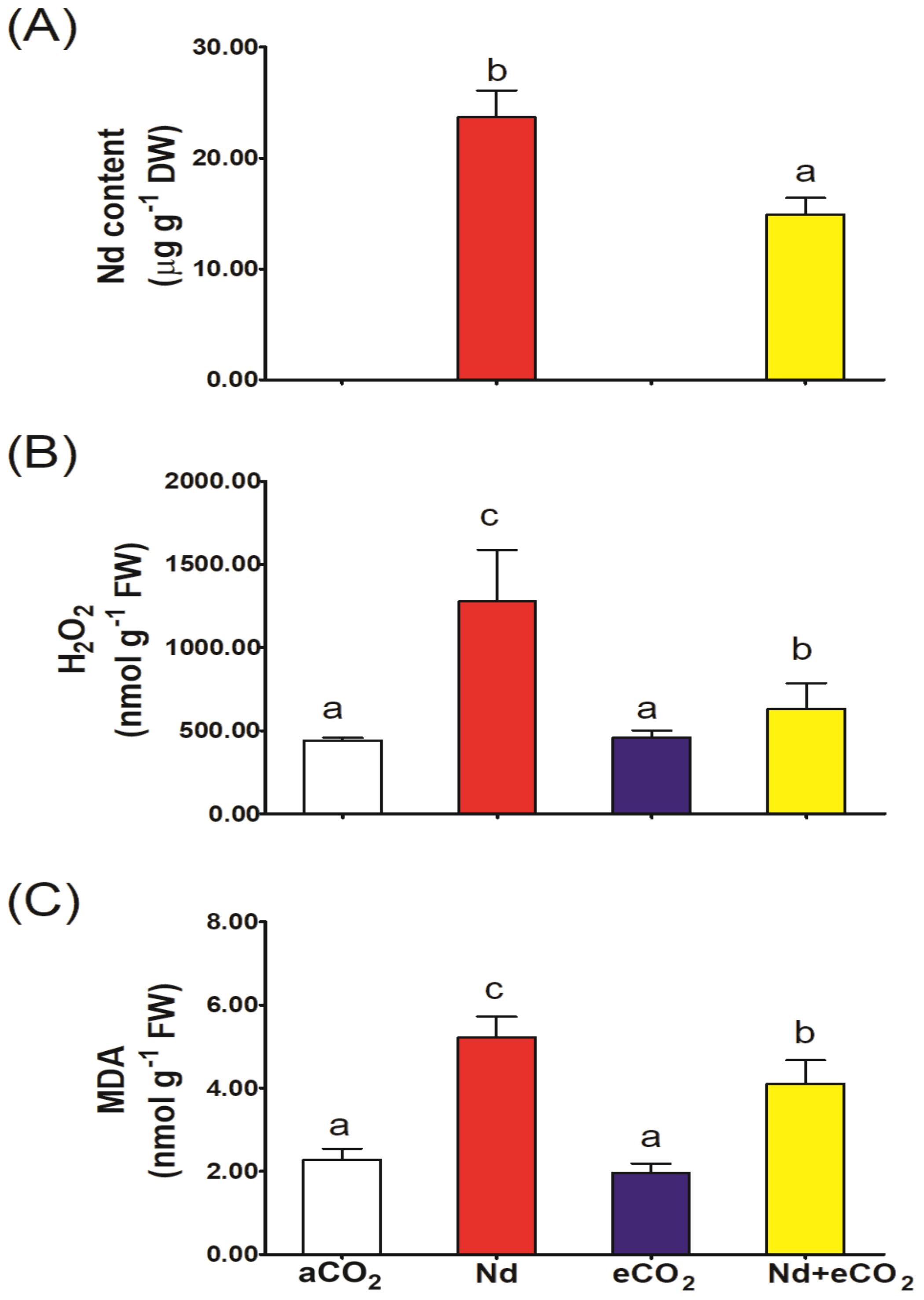
Figure 1. Effect of neodymium (Nd), elevated CO2 (eCO2) and their combination (Nd+eCO2) on the accumulation of Nd and oxidative stress markers in shoots of 4-weeks old wheat (Triticum aestivum) plants. (A) Nd concentration, mg g-1 DW; (B) H2O2, nmol g-1 FW; (C) malondialdehyde, MDA nmol g-1 FW. Each value is the mean of 5 independent replicates and the vertical bars demonstrates the standard error. Similar lower-case letters on the bars, within the same graph, indicate non-significant difference at the 0.05 probability level as indicated by Tukey’s multiple range tests.
3.3 Molecular antioxidants and total antioxidant capacity
Wheat plants treated with Nd only significantly accumulated polyphenols but not flavonoids, when compared to the control. However, plants subjected to eCO2 under Nd-free conditions contained significantly higher levels of flavonoids and total polyphenols (Figures 2A, B). Both Nd and elevate [CO2] significantly increased the content of carotenoids and total antioxidant capacity (FRAP) in wheat plants (Figures 2C, D) relative to Nd per se.
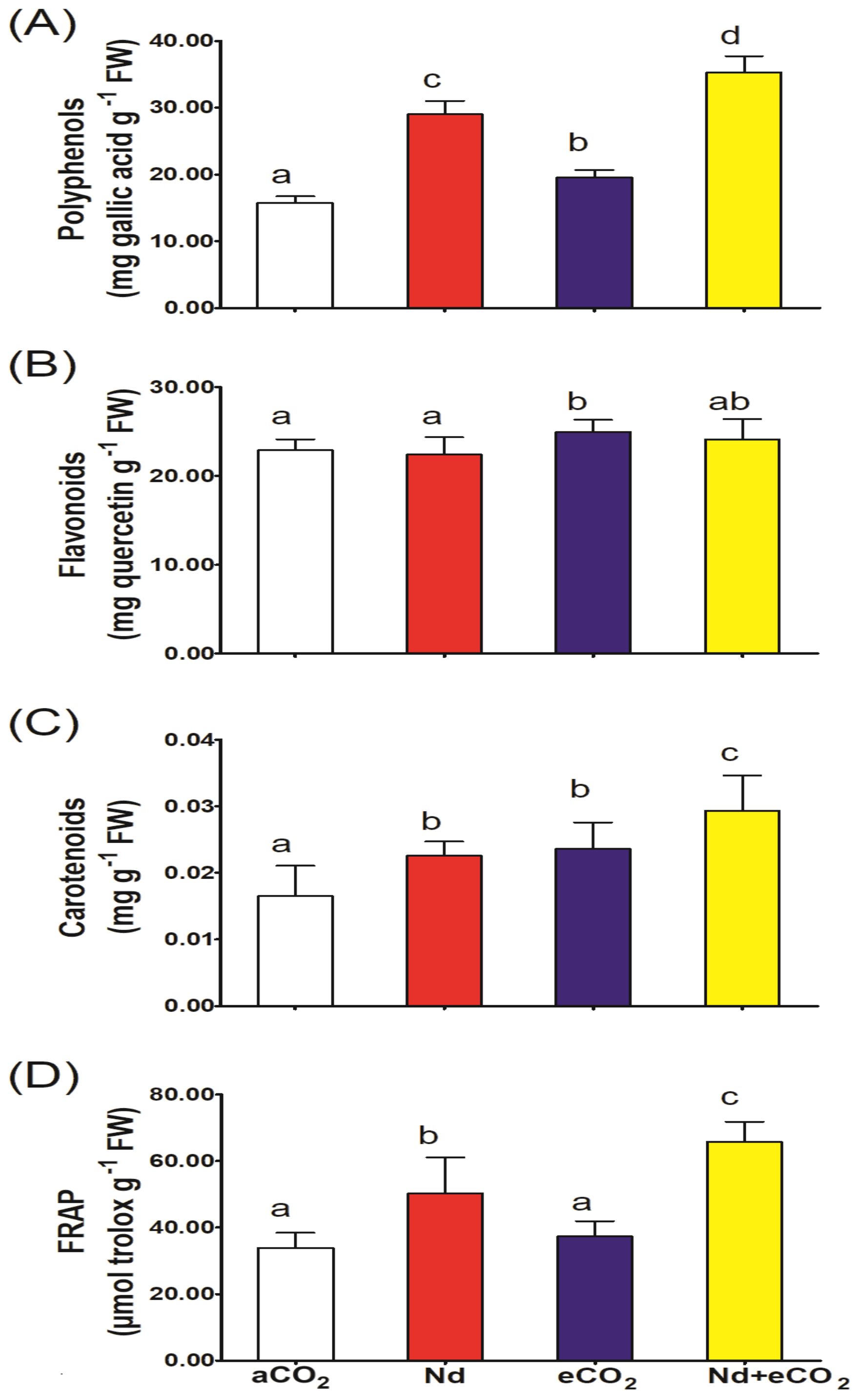
Figure 2. Effect of neodymium (Nd), elevated CO2 (eCO2) and their combination (Nd+eCO2) on the accumulation of molecular antioxidants and total antioxidant capacity (FRAP) in shoots of 4-weeks old wheat (Triticum aestivum) plants. (A) polyphenols, mg gallic acid g-1 FW; (B) flavonoids, mg quercetin g-1 FW; (C) carotenoids, mg g-1 FW; (D) FRAP mmol troloxg-1 FW. Each value is the mean of 5 independent replicates and the vertical bars demonstrates the standard error. Similar lower-case letters on the bars, within the same graph, indicate non-significant difference at the 0.05 probability level as indicated by Tukey’s multiple range tests.
3.4 Antioxidants enzymes and glutathione-S-transferase
Neodymium treatment upregulated the activities of SOD, POX and GPX by about 69, 38 and 29%, respectively, relative to the control (Figures 3A–C). On the other hand, eCO2 alone treatment decreased the activity of SOD and had no impact on GPX. No significant variation was detected in the catalase activity in response to either treatment (Figure 3D). GST activity was significantly enhanced (23%) in response to Nd treatment, but not eCO2 (Figure 3E). The activities of POX, GPX and GST showed further improvements in response to the synchronous application of eCO2 and Nd (120, 27 and 31%, respectively), compared to the Nd alone treatment.
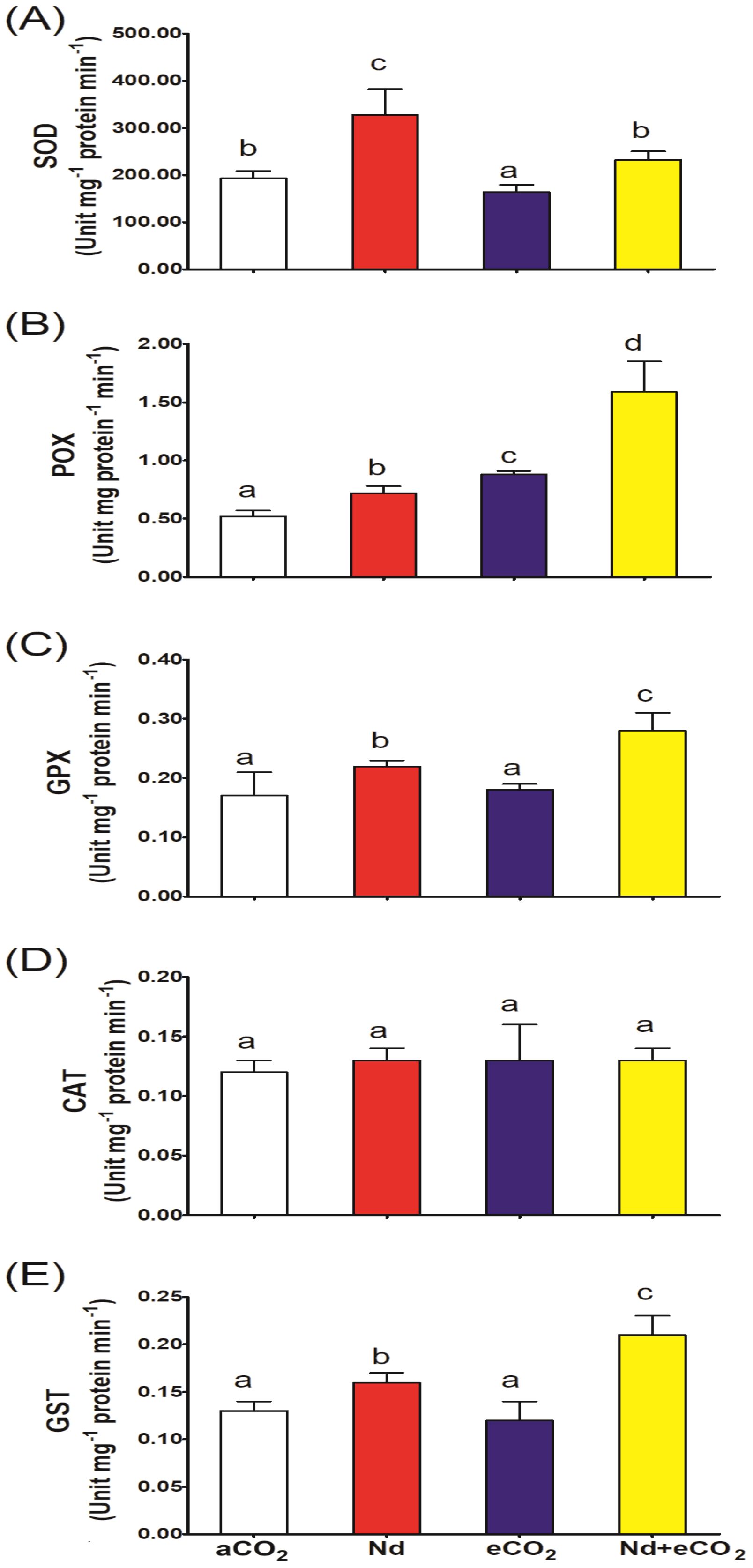
Figure 3. Effect of neodymium (Nd), elevated CO2 (eCO2) and their combination (Nd+eCO2) on the activities of antioxidant and detoxification enzymes (unit mg-1 protein min-1) in shoots of 4-weeks old wheat (Triticum aestivum) plants. (A) SOD, superoxide dismutase; (B) POX, peroxidase; (C) GPX, glutathione peroxidase; (D) CAT, catalase; (E) GST, glutathione-S-transferase. Each value is the mean of 5 independent replicates and the vertical bars demonstrates the standard error. Similar lower-case letters on the bars, within the same graph, indicate non-significant difference at the 0.05 probability level as indicated by Tukey’s multiple range tests.
3.5 Metabolites and enzymes of ascorbate-glutathione cycle
Nd treatment per se increased the accumulation of all metabolites of the ASC-GSH cycle relative to the control (Figure 4). However, the impact of Nd was much more evident on the oxidized forms where it increased DHA and GSSG levels 4x and 2.5x, respectively (Figures 4B, E). Accordingly, Nd stress resulted in sharp reduction in the ASC/DHA and GSH/GSSG redox balances (Figures 4C, F). Such Nd-induced negative impact was associated with inhibition in the activities of ASC-GSH cycling enzymes (APX, DHR, MDHAR and GR; Figures 5A–D). On the other hand, eCO2 alone had no significant impact on the ASC/DHA ratio but improved that of GSH/GSSG (59%). If additional CO2 is provided, the levels of ASC and GSH increased (13 and 15%, respectively) and the activities of DHAR, MDHAR and GR increased (22, 20 and 25%, respectively) when compared to their respective values in Nd alone treated plants. In addition, eCO2 resulted in a significant elevation in the GSH/GSSG redox balance (65%) in Nd-stressed plants.
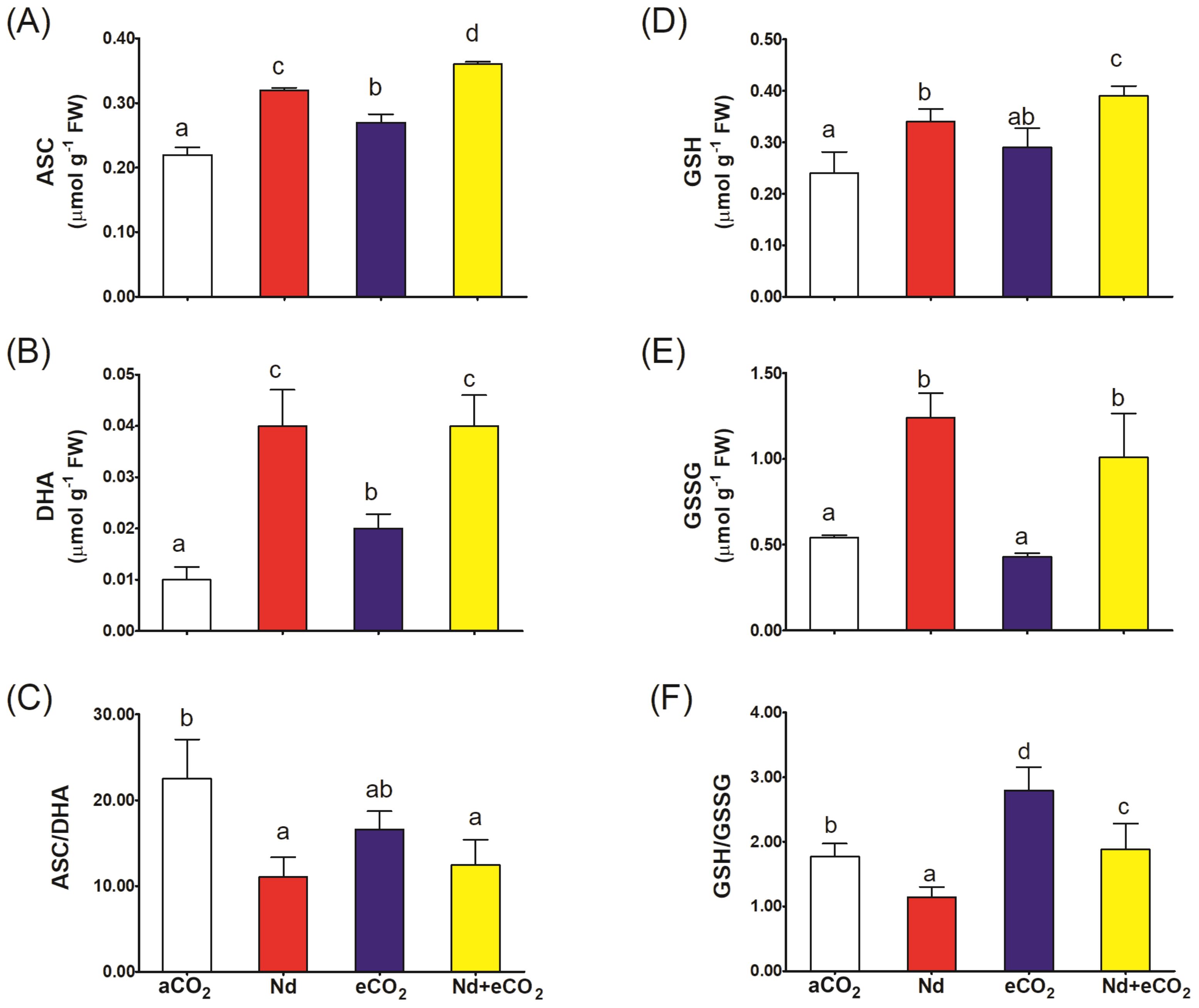
Figure 4. Effect of neodymium (Nd), elevated CO2 (eCO2) and their combination (Nd+eCO2) on the levels of the metabolites of ascorbate-glutathione (ASC-GSH) cycle (µmol g-1 FW) in shoots of 4-weeks old wheat (Triticum aestivum) plants. (A) ASC, reduced ascorbate; (B) DHA, oxidized ascorbate; (C) ASC/DHA ratio; (D) GSH, reduced glutathione; (E) GSSG, oxidized glutathione; (F) GSH/GSSG ratio. Each value is the mean of 5 independent replicates and the vertical bars demonstrates the standard error. Similar lower-case letters on the bars, within the same graph, indicate non-significant difference at the 0.05 probability level as indicated by Tukey’s multiple range tests.
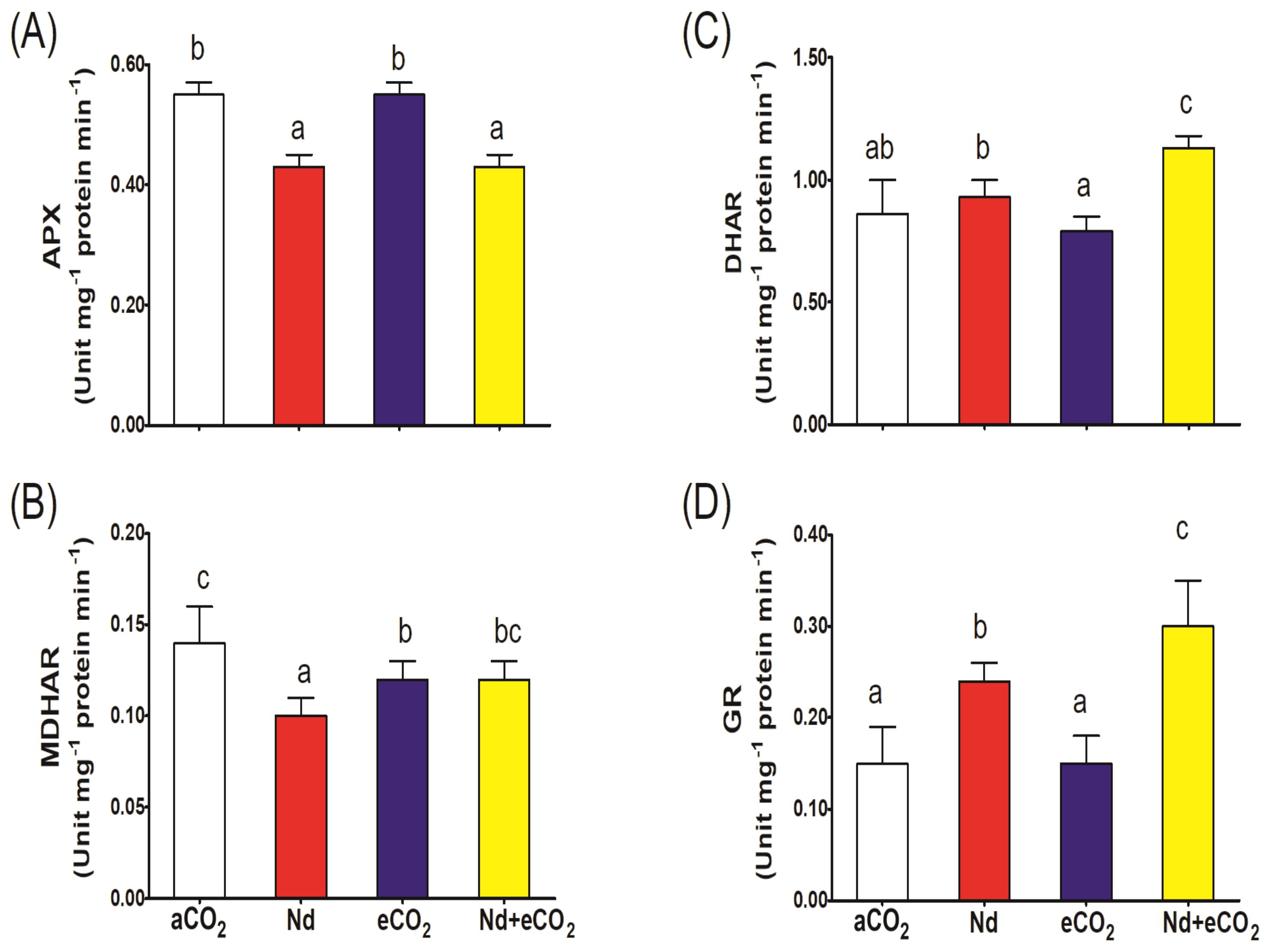
Figure 5. Effect of neodymium (Nd), elevated CO2 (eCO2) and their combination (Nd+eCO2) on the activities of the enzymes of ascorbate-glutathione (ASC-GSH) cycle (unit mg-1 protein min-1) in shoots of 4-weeks old wheat (Triticum aestivum) plants. (A) APX, ascorbate peroxidase; (B) MDHAR, monodehydroascorbate reductase; (C) DHAR, dehydroascorbate reductase; (D) GR, glutathione reductase. Each value is the mean of 5 independent replicates and the vertical bars demonstrates the standard error. Similar lower-case letters on the bars, within the same graph, indicate non-significant difference at the 0.05 probability level as indicated by Tukey’s multiple range tests.
4 Discussion
4.1 Elevated CO2 can mitigate the negative impact of Nd on wheat growth and photosynthesis
The current results highlight that Nd soil contamination can notably hinder the early growth of wheat seedlings. Although the phytotoxic effects of Nd are not fully understood, they are believed to be concentration-dependent. At low to moderate concentrations, Nd has been shown to promote plant growth, including that of wheat and rice (Luo et al., 2008; Rezaee et al., 2018). However, at higher concentrations (10 and 25 mg l⁻¹ Nd₂O₃), Nd exhibits inhibitory effects on plant growth (Basu and Dhal, 2016).
Similar behavior was reported in Helianthus annuus and Brassica chinensis subjected to different levels of Nd (Rezaee et al., 2018; Rezaee, 2016). The phytotoxic effects of Nd were reported at various concentrations depending on plant species and environmental conditions. Currently 200 mg Nd3+kg-1 soil indicated adverse effect on wheat where Carpenter et al. (2015) reported that Nd at 319 mg/kg were significantly toxic and adversely affected plant growth and overall biomass when studied some native and crop species. Zhao et al. (2019) also assessed the effects of different concentrations of Nd on the growth of various crop species. It was found that concentrations as low as 100 mg/kg of Nd resulted in notable reductions in root development of wheat.
The reduction in plant fresh and dry biomass is a logical consequence of the phytotoxicity of metals on the important physiological processes, e.g., photosynthesis and nutrient utilization (Malik et al., 2019). Like most REEs, Nd may interfere with the key plant metabolic processes and components such as rate of photosynthesis, total chlorophylls, chlorophyll fluorescence, stomatal conductance and rubisco activity since all were significantly decreased in relation to control. In this regard, Rezaee (2016) concluded that the inhibition in chlorophyll biosynthesis in B. chinensis started at Nd concentration of 200 ppm. Nd exposure has been shown to disrupt chlorophyll fluorescence in Myriophyllum aquaticum coupled with significant chlorophyll degradation (Gjata et al., 2024). Furthermore, exposure to Nd also inhibited rubisco enzyme activity, a critical component in carbon fixation during photosynthesis which is in accordance with report about Cd and Cu toxicity in tobacco plants (Khairy et al., 2016). As one of REEs, lanthanum disrupts photosynthesis and affects the over whole plant health (Jiao et al., 2024). Exposure to Nd has been demonstrated to disrupt chlorophyll fluorescence in Myriophyllum aquaticum, leading to significant chlorophyll degradation (Gjata et al., 2024). Additionally, Nd exposure inhibits the activity of the rubisco enzyme, which is essential for carbon fixation during photosynthesis, similar to the effects of cadmium (Cd) and copper (Cu) toxicity observed in tobacco plants (Khairy et al., 2016). As a member of the rare earth elements (REEs), lanthanum also interferes with photosynthesis, impacting overall plant health (Jiao et al., 2024). Currently elevated [CO2] by itself significantly improved the growth and photosynthesis in wheat, which is consistent with the report of Kang et al. (2021) when investigated wheat and rice. Further, the aerial growth and photosynthetic rates were increased as consequences to eCO2 in oak seedlings (Sanchez-Luca et al., 2023). Synchronous application of eCO2 and HMs was reported to lessen the toxic effects of HMs on plant growth and yield, especially in C3 plant systems, through inducing the synthesis of chlorophyll and its fluorescence, stomatal conductance, and net photosynthetic rate (Habeeb et al., 2020; Saleh et al., 2021). It is of interest to note here that additional CO2 only partially relieved the negative impact of Nd on plant crucial processes. Similarly, eCO2 improved the biomass production and photosynthesis of wheat and antagonized the negative impact of NiO nanoparticles and Al on these processes (Saleh et al., 2019; AbdElgawad et al., 2021). To date, there are no previous studies on the interactive effects of Nd and eCO2 on plants. However, the ameliorative effect of eCO2 on HM toxicity is not universal and depends on the specific HM and plant species. Current data therefore highlights a significant interaction between Nd and Elevated [CO2], where the beneficial effects of Elevated [CO2] are partially offset by the presence of Nd, suggesting the need for further research into the mechanisms of this interaction.Top of FormBottom of Form
4.2 Accumulation of Nd in wheat plants induces less oxidative damage under eCO2 environment
The combined effect of Nd and elevated [CO2] can help mitigate the oxidative stress typically induced by Nd accumulation in wheat plants. This interaction is crucial for understanding how these conditions affect plant health and productivity. Wheat plants grown in NdCl3 contaminated soil accumulated high levels of Nd in their shoots and showed the highest levels of H2O2 and MDA. Similar results were reported in lentil seedlings treated with Nd (Gjata et al., 2022). Hyperaccumulation of H2O2 and MDA in plants is an evident sign for severe oxidative cellular damage. Indeed, it has been reported that REEs could alter the redox activity and plasma membrane permeability of plant cells, which can lead to membrane disfunction and increased ROS and MDA levels (Oral et al., 2017; Peng et al., 2007; Zicari et al., 2018). Consistent with the current results, elevated levels of H2O2 and MDA were recorded in onion plants treated with Nd (Gjata et al., 2022). It suggests that the reactive oxygen species (ROS) production is a common plant response suffer metal stress conditions.
Interestingly, elevated [CO2] reduced the accumulation of Nd in wheat plants and, therefore, the levels of oxidative stress markers, H2O2 and MDA were also reduced. Lower Nd levels could be associated with eCO2 induced reductions in stomatal conductance and lower Nd uptake through transportational flow. In addition, elevated [CO2] is known to downregulate photorespiration, a major H2O2-producing process in plants that is enhanced during oxidative stress (Foyer and Noctor, 2000). The decline in Nd observed here is consistent with other studies in wheat showing that elevated [CO2] can reduce the uptake and accumulation of Ni (Saleh et al., 2019) and Al (AbdElgawad et al., 2021). In addition, the effect of eCO2 on photorespiration and ROS production in wheat and other crops has been previously reported (Saleh et al., 2019, 2021; AbdElgawad et al., 2022b). Indium oxide nanoparticles induced the H2O2, lipid peroxidation levels which has been mitigated by elevated [CO2] in maize and barely (Shabbaj et al., 2022).
To compensate for Nd-induced ROS production, wheat plants synthesized more polyphenols, and carotenoids, which are integrated into TAC, i.e., FRAR. Similarly, wheat plants suffering HMs-induced oxidative stress were reported to accumulate various molecular antioxidants (Saleh et al., 2019, 2021). Application of elevated [CO2], either alone or in conjunction with Nd, also induced more polyphenols and carotenoids. The upregulation of molecular antioxidants in plants grown in elevated [CO2] environments, under either HMs free or stressed conditions, have been previously reported (AbdElgawad et al., 2023; Al Jaouni et al., 2018; Saleh et al., 2018).
Overproduction of ROS can be compensated, in part, by stimulating the production of antioxidizing and detoxifying enzymes. This was observed in the current study with noted increases in SOD, POX, GPX and GST in Nd-treated plants. This stimulation has also been observed for SOD and POD enzymes in spinach, wheat and rice plants treated with Nd (Chao et al., 2009; Shi et al., 2021).
Elevated [CO2] also stimulated the activities of POX, GPX and GST which could explain the decreased levels of H2O2 and MDA in Nd + eCO2 treated plants, compared to Nd alone. Similarly, Elevated [CO2] was reported to upregulate the enzymatic defense pool in several plant species, including wheat, under HMs stressed conditions (Saleh et al., 2019, 2021; AbdElgawad et al., 2021, 2022b, 2022a).
Besides the higher concentrations of H2O2 and MDA, declines in GSH/GSSG and ASC/DHA redox balances were recorded in Nd-treated plants. Such reductions in GSH/GSSG and ASC/DHA redox balances could be explained by the negative impact of Nd on ASC-GSH recycling enzymes, e.g., APX and MDHAR. Similarly, 25 μM Nd was reported to decrease the ASC/total ASC ratio in roots of lentil seedlings (Gjata et al., 2022). Elevation of GSH/GSSG ratio in response to eCO2, as a result for upregulating the activities of DHAR and GR, indicates its ability to adapt to the oxidative damage induced by Nd. Several studies have reported the positive impact of eCO2 on ASC-GSH recycling in plants subjected to HMs (Saleh et al., 2019, 2021; AbdElgawad et al., 2021, 2022b, 2022a). The interaction between Nd and eCO2 showed that elevated CO2 have high effects on plant response under Nd stress. Significant interactions of Nd and eCO2 were observed for most of the investigated parameters. Such findings suggested that the combined Nd and elevated CO2 may trigger antioxidant defences system that may help to ameliorate some of the negative impacts of Nd by enhancing the plant’s antioxidant capacity. Further research into the ecological implications of REE accumulation in agricultural systems, especially under changing atmospheric conditions such as increased CO2 is needed.
5 Conclusion
The current study provides the first comprehensive analysis of oxidative stress in wheat caused by neodymium (Nd) and explores the potential adaptive benefits of elevated atmospheric CO2 levels. While increased CO2 concentrations do not completely mitigate the growth reductions induced by Nd, they do alleviate some oxidative stress. Potential strategies for mitigating the effects of Nd under elevated CO2 include: 1) reducing Nd uptake and accumulation in photosynthetic tissues while lowering stomatal conductance; 2) mitigating the negative impact of Nd on photosynthetic carbon assimilation by enhancing photochemical efficiency and Rubisco activity; and 3) minimizing the increase in reactive oxygen species (ROS) generated by Nd at both production and elimination stages. Although these findings are promising, they remain preliminary, and further research is essential to fully understand the metabolic interactions between elevated CO2 and rare earth elements (REEs).
Data availability statement
The original contributions presented in the study are included in the article/Supplementary Material. Further inquiries can be directed to the corresponding author.
Author contributions
AS: Conceptualization, Data curation, Investigation, Validation, Writing – original draft, Writing – review & editing. MH: Conceptualization, Writing – original draft, Writing – review & editing. AM: Data curation, Writing – original draft, Writing – review & editing. LZ: Writing – original draft, Writing – review & editing. MA: Investigation, Writing – review & editing. AK: Conceptualization, Investigation, Writing – original draft. MM: Conceptualization, Data curation, Formal Analysis, Writing – original draft. HA: Conceptualization, Supervision, Validation, Writing – original draft. HEA: Investigation, Software, Writing – original draft.
Funding
The author(s) declare that financial support was received for the research, authorship, and/or publication of this article. This research has been supported by Princess Nourah bint Abdulrahman University Researchers Supporting Project number (PNURSP2025R740), Princess Nourah bint Abdulrahman University, Riyadh, Saudi Arabia.
Acknowledgments
The authors are grateful to Princess Nourah bint Abdulrahman University Researchers Supporting Project number (PNURSP2025R740), Princess Nourah bint Abdulrahman University, Riyadh, Saudi Arabia.
Conflict of interest
The authors declare that the research was conducted in the absence of any commercial or financial relationships that could be construed as a potential conflict of interest.
Generative AI statement
The author(s) declare that no Generative AI was used in the creation of this manuscript.
Publisher’s note
All claims expressed in this article are solely those of the authors and do not necessarily represent those of their affiliated organizations, or those of the publisher, the editors and the reviewers. Any product that may be evaluated in this article, or claim that may be made by its manufacturer, is not guaranteed or endorsed by the publisher.
Supplementary material
The Supplementary Material for this article can be found online at: https://www.frontiersin.org/articles/10.3389/fpls.2025.1521460/full#supplementary-material
References
AbdElgawad, H., de Soua, A., Alotaibi, M. O., Mohammed, A. E., Schoenaers, S., Selim, S., et al. (2021). The differential tolerance of C3 and C4 cereals to aluminum toxicity is faded under future CO2 climate. Plant Physiol. Biochem. 169, 249–258. doi: 10.1016/j.plaphy.2021.11.018
AbdElgawad, H., El-Sawah, A. M., Mohammed, A. E., Alotaibi, M. O., Yehia, R. S., Selim, S., et al. (2022a). Increasing atmospheric CO2 differentially supports arsenite stress mitigating impact of arbuscular mycorrhizal fungi in wheat and soybean plants. Chemosphere 296, 134044. doi: 10.1016/j.chemosphere.2022.134044
Abdelgawad, H., Farfan-vignolo, E. R., De Vos, D., Asard, H. (2015). Elevated CO2 mitigates drought and temperature-induced oxidative stress differently in grasses and legumes. Plant Sci. 231, 1–10. doi: 10.1016/j.plantsci.2014.11.001
AbdElgawad, H., Mohammed, A. E., van Dijk, J. R., Beemster, G. T. S., Alotaibi, M. O., Saleh, A. M. (2023). The impact of chromium toxicity on the yield and quality of rice grains produced under ambient and elevated levels of CO 2. Front. Plant Sci. 14. doi: 10.3389/fpls.2023.1019859
AbdElgawad, H., Sheteiwy, M. S., Saleh, A. M., Mohammed, A. E., Alotaibi, M. O., Beemster, G. T. S., et al. (2022b). Elevated CO2 differentially mitigates chromium (VI) toxicity in two rice cultivars by modulating mineral homeostasis and improving redox status. Chemosphere 307, 135880. doi: 10.1016/j.chemosphere.2022.135880
Aebi, H. (1984). “[13] catalase in vitro,” in Methods Enzymol Academic press 105, 121–126. doi: 10.1016/S0076-6879(84)05016-3
Agusa, T., Kunito, T., Yasunaga, G., Iwata, H., Subramanian, A., Ismail, A., et al. (2005). Concentrations of trace elements in marine fish and its risk assessment in Malaysia. Mar. pollut. Bull. 51, 896–911. doi: 10.1016/j.marpolbul.2005.06.007
Al Jaouni, S., Saleh, A. M., Wadaan, M. A. M., Hozzein, W. N., Selim, S., AbdElgawad, H. (2018). Elevated CO2 induces a global metabolic change in basil (Ocimum basilicum L.) and peppermint (Mentha piperita L.) and improves their biological activity. J. Plant Physiol. 224–225, 121–131. doi: 10.1016/j.jplph.2018.03.016
Athar, R., Ahmad, M. (2002). Heavy metal toxicity: Effect on plant growth and metal uptake by wheat, and on free living Azotobacter. Water Air Soil pollut. 138, 165–180. doi: 10.1023/A:1015594815016
Basu, A., Dhal, N. K. (2016). Bioaccumulation of neodymium oxide (REE) and its effects on the growth and physiological changes of wheat and rice seedlings: A hydroponics study under plant growth chamber. e-Planet, 14 (2), 33–40.
Benzie, I. F. F., Strain, J. J. (1999). “[2] Ferric reducing/antioxidant power assay: Direct measure of total antioxidant activity of biological fluids and modified version for simultaneous measurement of total antioxidant power and ascorbic acid concentration,” in Methods in enzymology (Elsevier), 15–27.
Carpenter, D., Boutin, C., Allison, J. E., Parsons, J. L., Ellis, D. M. (2015). Uptake and effects of six rare earth elements (REEs) on selected native and crop species growing in contaminated soils. PLoS One 10 (6), e0129936. doi: 10.1371/journal.pone.0129936
Chang, C.-C., Yang, M.-H., Wen, H.-M., Chern, J.-C. (2002). Estimation of total flavonoid content in propolis by two complementary colorimetric methods. J. Food Drug Anal. 10 (3). doi: 10.38212/2224-6614.2748
Chao, L., Weiqian, C., Yun, L., Hao, H., Liang, C., Xiaoqing, L., et al. (2009). Cerium under calcium deficiency-influence on the antioxidative defense system in spinach plants. Plant Soil 323, 285–294. doi: 10.1007/s11104-009-9937-9
Dhindsa, R. S., Plumb-Dhindsa, P. L., Reid, D. M. (1982). Leaf senescence and lipid peroxidation: Effects of some phytohormones, and scavengers of free radicals and singlet oxygen. Physiol. Plant 56, 453–457. doi: 10.1111/j.1399-3054.1982.tb04539.x
Diopan, V., Shestivska, V., Adam, V., Macek, T., Mackova, M., Havel, L., et al. (2008). Determination of content of metallothionein and low molecular mass stress peptides in transgenic tobacco plants. Plant Cell Tissue Organ Culture 2008 94:3 94, 291–298. doi: 10.1007/S11240-008-9356-2
Drotar, A., Phelps, P., Fall, R. (1985). Evidence for glutathione peroxidase activities in cultured plant cells. Plant Sci. 42, 35–40. doi: 10.1016/0168-9452(85)90025-1
Foyer, C. H., Noctor, G. (2000). Tansley Review No. 112 Oxygen processing in photosynthesis: regulation and signalling. New Phytol. 146, 359–388. doi: 10.1046/j.1469-8137.2000.00667.x
Gjata, I., Tommasi, F., De Leonardis, S., Dipierro, N., Paciolla, C. (2022). Cytological alterations and oxidative stress induced by Cerium and Neodymium in lentil seedlings and onion bulbs. Front. Environ. Sci. 10. doi: 10.3389/fenvs.2022.969162
Gjata, I., van Drimmelen, C. K. E., Tommasi, F., Paciolla, C., Heise, S. (2024). Impact of Rare Earth Elements in sediments on the growth and photosynthetic efficiency of the benthic plant Myriophyllum aquaticum. J. Soils Sediments. 24, 3814–382. doi: 10.1007/s11368-024-03867-x
Guo, H., Zhu, J., Zhou, H., Sun, Y., Yin, Y., Pei, D., et al. (2011). Elevated CO 2 levels affects the concentrations of copper and cadmium in crops grown in soil contaminated with heavy metals under fully open-air field conditions. Environ. Sci. Technol. 45 (16), 6997–7003. doi: 10.1021/es2001584
Habeeb, T. H., Abdel-mawgoud, M., Yehia, R. S., Khalil, A. M. A., Saleh, A. M., AbdElgawad, H. (2020). Interactive impact of arbuscular mycorrhizal fungi and elevated CO 2 on growth and functional food value of thymus vulgare. J. Fungi 6, 1–14. doi: 10.3390/jof6030168
Hammami, H., Parsa, M., Bayat, H., Aminifard, M. H. (2022). The behavior of heavy metals in relation to their influence on the common bean (Phaseolus vulgaris) symbiosis. Environ. Exp. Bot. 193, 104670. doi: 10.1016/j.envexpbot.2021.104670
Hodges, D. M., DeLong, J. M., Forney, C. F., Prange, R. K. (1999). Improving the thiobarbituric acid-reactive-substances assay for estimating lipid peroxidation in plant tissues containing anthocyanin and other interfering compounds. Planta 207, 604–611. doi: 10.1007/s004250050524
Hu, H., Wang, L., Li, Y., Sun, J., Zhou, Q., Huang, X. (2016). Insight into mechanism of lanthanum (III) induced damage to plant photosynthesis. Ecotoxicol Environ. Saf. 127, 43–50. doi: 10.1016/j.ecoenv.2016.01.008
Jiang, Z.-Y., Woollard, A. C. S., Wolff, S. P. (1990). Hydrogen peroxide production during experimental protein glycation. FEBS Lett. 268, 69–71. doi: 10.1016/0014-5793(90)80974-N
Jiao, Y., He, D., Zhang, S., Cheng, M., Chen, S., Dong, T., et al. (2024). Lanthanum interferes with the fundamental rhythms of stomatal opening, expression of related genes, and evapotranspiration in Arabidopsis thaliana. Ecotoxicol Environ. Saf. 281, 116576. doi: 10.1016/j.ecoenv.2024.116576
Kang, H., Zhu, T., Zhang, Y., Ke, X., Sun, W., Hu, Z., et al. (2021). Elevated CO2 enhances dynamic photosynthesis in rice and wheat. Front. Plant Sci. 12. doi: 10.3389/fpls.2021.727374
Khairy, A. I. H., Oh, M. J., Lee, S. M., Kim, D. S., Roh, K. S. (2016). Nitric oxide overcomes Cd and Cu toxicity in in vitro-grown tobacco plants through increasing contents and activities of rubisco and rubisco activase. Biochim. Open 2, 41–51. doi: 10.1016/j.biopen.2016.02.002
Kumar, K. B., Khan, P. A. (1982). Peroxidase and polyphenol oxidase in excised ragi (Eleusine corocana cv PR 202) leaves during senescence. Indian J. Exp. Botany.
Lowry, O. H., Rosebrough, N. J., Farr, A. L., Randall, R. J. (1951). Protein measurement with the Folin phenol reagent. J. Biol. Chem. 193, 265–275. doi: 10.1016/s0021-9258(19)52451-6
Luo, J., Zhang, J., Wang, Y. (2008). Changes in endogenous hormone levels and redox status during enhanced adventitious rooting by rare earth element neodymium of Dendrobium densiflorum shoot cuttings. J. Rare Earths 26, 869–874. doi: 10.1016/S1002-0721(09)60023-5
Malik, B., Pirzadah, T. B., Tahir, I., Ul Rehman, R. (2019). Growth and physiological responses in chicory towards mercury induced in vitro oxidative stress. Plant Physiol. Rep. 24, 236–248. doi: 10.1007/s40502-019-00442-2
Mohammed, A. E., Pawelzik, E., Nour, M. M., Alotaibi, M. O., Abdelgawad, H., Saleh, A. M. (2023). Mycorrhized wheat and bean plants tolerate bismuth contaminated soil via improved metal detoxification and antioxidant defense systems. Plant Physiology and Biochemistry 205, 108148.
Murray, V., Ebi, K. L. (2012). IPCC special report on managing the risks of extreme events and disasters to advance climate change adaptation (SREX). J. Epidemiol. Community Health (1978) 66, 759–760. doi: 10.1136/jech-2012-201045
Murshed, R., Lopez-Lauri, F., Sallanon, H. (2008). Microplate quantification of enzymes of the plant ascorbate-glutathione cycle. Anal. Biochem. 383 (2), 320–322. doi: 10.1016/j.ab.2008.07.020
Nunes, L. J. R. (2023). The rising threat of atmospheric CO2: A review on the causes, impacts, and mitigation strategies. Environments 10 (4), 66. doi: 10.3390/environments10040066
Oral, R., Pagano, G., Siciliano, A., Gravina, M., Palumbo, A., Castellano, I., et al. (2017). Heavy rare earth elements affect early life stages in Paracentrotus lividus and Arbacia lixula sea urchins. Environ. Res. 154, 240–246. doi: 10.1016/j.envres.2017.01.011
Patra, A. C., Lenka, P., Sahoo, S. K., Jha, S. K., Kulkarni, M. S. (2020). Probing rare earth element distributions in soils of the mineralized Singhbhum region in India using INAA. Appl. Radiat. Isotopes 166, 109360. doi: 10.1016/j.apradiso.2020.109360
Peng, L., Weiying, Z., Xi, L., Yi, L. (2007). Structural basis for the biological effects of Pr(III) ions: Alteration of cell membrane permeability. Biol. Trace Elem Res. 120, 141–147. doi: 10.1007/s12011-007-8015-5
Petrini, A., Scortichini, G., Merola, C. (2025). Rare Earth Elements (REEs) in veterinary medicine: practical applications and tissue distribution in terrestrial vertebrate animals. Veterinaria Italiana 61, 1–9. doi: 10.12834/VetIt.3582.26784.1
Porra, R. J., Thompson, W. A., Kriedemann, P. E. (1989). Determination of accurate extinction coefficients and simultaneous equations for assaying chlorophylls a and b extracted with four different solvents: verification of the concentration of chlorophyll standards by atomic absorption spectroscopy. BBA - Bioenergetics 975 (3), 384–394. doi: 10.1016/S0005-2728(89)80347-0
Potters, G., Horemans, N., Bellone, S., Caubergs, R. J., Trost, P., Guisez, Y., et al. (2004). Dehydroascorbate influences the plant cell cycle through a glutathione-independent reduction mechanism. Plant Physiol. 134, 1479–1487. doi: 10.1104/pp.103.033548
Revel, M., van Drimmelen, C. K. E., Weltje, L., Hursthouse, A., Heise, S. (2025). Effects of rare earth elements in the aquatic environment: Implications for ecotoxicological testing. Crit. Rev. Environ. Sci. Technol. 55, 334–375. doi: 10.1080/10643389.2024.2406992
Rezaee, A. (2016). Accumulation and toxicity of lanthanum and neodymium in horticultural plants. (Doctoral dissertation, University of Guelph).
Rezaee, A., Hale, B., Santos, R. M., Chiang, Y. W. (2018). Accumulation and toxicity of lanthanum and neodymium in horticultural plants (Brassica chinensis L. and Helianthus annuus L.). Can. J. Chem. Eng. 96 (10), 2263–2272. doi: 10.1002/cjce.23152
Rizvi, A., Zaidi, A., Ameen, F., Ahmed, B., Alkahtani, M. D. F., Khan, M. S. (2020). Heavy metal induced stress on wheat: Phytotoxicity and microbiological management. RSC Adv. 10 (63), 38379–38403. doi: 10.1039/d0ra05610c
Saleh, A. M., Hassan, Y. M., Habeeb, T. H., Alkhalaf, A. A., Hozzein, W. N., Selim, S., et al. (2021). Interactive effects of mercuric oxide nanoparticles and future climate CO2 on maize plant. J. Hazard Mater 401, 123849. doi: 10.1016/j.jhazmat.2020.123849
Saleh, A. M., Hassan, Y. M., Selim, S., AbdElgawad, H. (2019). NiO-nanoparticles induce reduced phytotoxic hazards in wheat (Triticum aestivum L.) grown under future climate CO2. Chemosphere 220, 1047–1057. doi: 10.1016/J.CHEMOSPHERE.2019.01.023
Saleh, A. M., Selim, S., Jaouni, S. A., AbdElgawad, H. (2018). CO2 enrichment can enhance the nutritional and health benefits of parsley (Petroselinum crispum L.) and dill (Anethum graveolens L.). Food Chem. 269, 519–526. doi: 10.1016/j.foodchem.2018.07.046
Sanchez-Luca, R., Mayoral, C., Raw, M., Mousouraki, M. A., Luna, E. (2023). Elevated CO2alters photosynthesis, growth and susceptibility to powdery mildew of oak seedlings. Biochem. J. 480, 1429–1443. doi: 10.1042/BCJ20230002
Selim, S., Abuelsoud, W., Al-Sanea, M. M., AbdElgawad, H. (2021). Elevated CO2 differently suppresses the arsenic oxide nanoparticles-induced stress in C3 (Hordeum vulgare) and C4 (Zea maize) plants via altered homeostasis in metabolites specifically proline and anthocyanin metabolism. Plant Physiol. Biochem. 166, 235–245. doi: 10.1016/j.plaphy.2021.05.036
Shabbaj, I. I., Abdelgawad, H., Balkhyour, M. A., Tammar, A., Madany, M. M. Y. (2022). Elevated CO2 differentially mitigated oxidative stress induced by indium oxide nanoparticles in young and old leaves of C3 and C4 crops. Antioxidants 11 (2), 308. doi: 10.3390/antiox11020308
Shabbaj, I. I., Madany, M. M. Y., Tammar, A., Balkhyour, M. A., AbdElgawad, H. (2021). Silicon dioxide nanoparticles orchestrate carbon and nitrogen metabolism in pea seedlings to cope with broomrape infection. Environ. Sci. Nano 8 (7), 1960–1977. doi: 10.1039/d0en01278e
Shi, K., Liu, C., Lyu, K., Chen, J., Wang, X. (2021). The bioaccumulation and detoxification mechanism of neodymium on the shoot of rice seedlings. Am. J. Biochem. Biotechnol. 17 (2), 140–147. doi: 10.3844/ajbbsp.2021.140.147
Wang, L., Christakos, G., Wu, C., Wu, J. (2020). Spatial variability assessment of La and Nd concentrations in coastal China soils following 1000 years of land reclamation. J. Soils Sediments 20, 1651–1661. doi: 10.1007/s11368-019-02488-z
Wang, Y., Wang, X., Ai, F., Du, W., Yin, Y., Guo, H. (2023). Potential microbial mechanisms underlying the effects of rising atmospheric CO2 levels on the reduction and methylation processes of arsenic in the paddy soil. Sci. Total Environ. 888, 164240. doi: 10.1016/j.scitotenv.2023.164240
Wang, W., Yang, Y., Wang, D., Huang, L. (2024). Toxic effects of rare earth elements on human health: A review. Toxics 12, 317. doi: 10.3390/toxics12050317
Zhang, Q., Zhang, J., Shen, J., Silva, A., Dennis, D. A., Barrow, C. J. (2006). A simple 96-well microplate method for estimation of total polyphenol content in seaweeds. J. Appl. Phycol 18, 445–450. doi: 10.1007/s10811-006-9048-4
Zhao, C. M., Shi, X., Xie, S. Q., Liu, W. S., He, E. K., Tang, Y. T., et al. (2019). Ecological risk assessment of neodymium and yttrium on rare earth element mine sites in Ganzhou, China. Bull. Environ. Contam Toxicol. 103, 565–570. doi: 10.1007/s00128-019-02690-2
Zicari, M. A., d’Aquino, L., Paradiso, A., Mastrolitti, S., Tommasi, F. (2018). Effect of cerium on growth and antioxidant metabolism of Lemna minor L. Ecotoxicol Environ. Saf. 163, 536–543. doi: 10.1016/j.ecoenv.2018.07.113
Keywords: neodymium, elevated CO2, wheat, photosynthesis, antioxidants
Citation: Saleh AM, Haridy MSA, Mohammed AE, Ziska L, Alotaibi MO, Khalil AMA, Madany MMY, Abd Elgawad H and Amer HEA (2025) Carbon dioxide-enriched atmosphere diminished the phytotoxicity of neodymium in wheat (Triticum aestivum L.). Front. Plant Sci. 16:1521460. doi: 10.3389/fpls.2025.1521460
Received: 01 November 2024; Accepted: 18 March 2025;
Published: 28 April 2025.
Edited by:
Mohd. Kamran Khan, Selçuk University, TürkiyeReviewed by:
José Manuel Moutinho-Pereira, University of Trás-os-Montes and Alto Douro, PortugalHanuman Singh Jatav, Sri Karan Narendra Agriculture University, India
Copyright © 2025 Saleh, Haridy, Mohammed, Ziska, Alotaibi, Khalil, Madany, Abd Elgawad and Amer. This is an open-access article distributed under the terms of the Creative Commons Attribution License (CC BY). The use, distribution or reproduction in other forums is permitted, provided the original author(s) and the copyright owner(s) are credited and that the original publication in this journal is cited, in accordance with accepted academic practice. No use, distribution or reproduction is permitted which does not comply with these terms.
*Correspondence: Afrah E. Mohammed, QUZBTW9oYW1tZWRAcG51LmVkdS5zYQ==