- 1School of Life Science, Luoyang Normal University, Luoyang, Henan, China
- 2School of Chemical Engineering, Huaqiao University, Xiamen, Fujian, China
Background: As one of the largest plant-specific transcription factor families, NAC proteins are crucial for plant growth and development processes and responses to various abiotic and biotic stresses. The published sequenced chromosome-level genome of ‘Fengdan’peony provides a powerful tool for the analysis of the NAC gene family in this shrub.
Methods: The PoNAC gene family was identified and characterized using bioinformatic analysis, and RT-qPCR analysis was performed on some PoNACs from the ATAF and NAP subfamilies.
Results: In this study, a total of 82 NAC transcription factors (TFs) were identified in the ‘Fengdan’ peony genome, with the uneven anchorage of 78 PoNAC genes on 5 chromosomes, whereas only 4 PoNAC genes were found to be located on unanchored scaffolds. Through the phylogenetic analysis, 66 PoNAC genes were classified into 15 distinct subfamilies. The gene structure analysis revealed the variation in the number of exons from 0 to 14. Moreover, the motif analysis indicated that the identified PoNAC TFs possessed conserved NAC domains and motifs. The duplication events of PoNAC genes included whole-genome duplications (WGDs) or segmental duplications for 14 pairs, tandem duplications for 2 pairs, and proximal duplications for 3 pairs. GO analysis results suggested that the functions of PoNAC genes were mostly concentrated in the “biological process” GO category. Additionally, the analysis of the expression profiles of PoNAC genes in different plant organs revealed that only 45 genes were expressed in various tissues, some of them exhibited tissue-specific expression related to plant growth and development. RT-qPCR experiments demonstrated the responses of 8 genes from the ATAF and NAP subfamilies to ABA, heat and drought, suggesting that they may serve as important regulatory factor.
Introduction
During their growth and development, plants encounter both biotic and abiotic stressors, which directly impact these processes as well as their yield. To overcome these challenges, plants have evolved complex regulatory networks over the course of their long-term evolution, which not only play a crucial role in the growth and development, but also have significant importance for plants in coping with stress responses (Zuo et al., 2023). Transcription factors (TF) regulate the expression of genes by either activation or inhibition through binding to specific DNA sequences located in the upstream promoter region of their target genes. The NAC TF family constitutes one of the largest and most widely distributed transcription factor families across the plant kingdom. Its proteins share a common sequence in the NAC domains (Ooak et al., 2003), including no apical meristem (NAM; in glory) (Duval et al., 2002), ATAF1/2 (in Arabidopsis) (Christianson et al., 2010), and cup-shaped cotyledon (CUC2; in Arabidopsis) (Aida et al., 1997).
Apart from a highly variable C-terminal transcriptional regulatory region (TRR), members of the NAC gene family possess a highly conserved N-terminal domain consisting of around 150 amino acids, which can be further separated into five subdomains (A to E) (Singh et al., 2021). Among them, subdomain A is involved in the formation of functional dimers, while B and E subdomains drive the functional diversity of NAC genes, and C and D subdomains, which include nuclear localization signals perform the DNA-binding activity (Jia et al., 2019). Although the NAC domain of different plants shares structural similarities, it has different functions in various plant parts and under different stress factors. The roles that NAC genes play in plants comprise the development of the whole plant, leaves (Hasson et al., 2011), and lateral roots (Xie et al., 2000), meristem maintenance (Zhan et al., 2021), secondary cell wall synthesis (Mccarthy et al., 2011), leaf senescence (Wang et al., 2021; Melo et al., 2021), etc.
The overexpression of ANACO32 in Arabidopsis can cause the downregulation of the expression of some anthocyanin biosynthesis genes, including DFR, ANS, and LODX, under stress conditions, which results in the regulation of anthocyanin biosynthesis (Meraj et al., 2020). Under drought stress, the survival rate and antioxidant enzyme activity of Arabidopsis plants overexpressing the maize ZmSNAC06-T02 gene were both higher than those of the wild type (Wang et al., 2025), while GmNAC20 in soybean can improve the tolerance of rice to cold stress (Yarra and Wei, 2021). Moreover, CaNAC2c can perform various functions in pepper, including the activation of the heat shock transcription factor CaHSFA5, prevention of the accumulation of H2O2, and enhancement of heat stress tolerance in the plant (Cai et al., 2021), and the arabidopsis ataf1 mutant was found to have higher survival rate and fresh weight than its wild-type counterpart under heat stress (Alshareef et al., 2022).
‘Fengdan’ peony (Paeonia ostii) is a traditionally famous flower native to China (Sheng et al., 2023) whose ornamental, medicinal, and oil values (Yang et al., 2020; Wang et al., 2019) have driven the rapid expansion and development of its seedling industry in recent years (Guo et al., 2023). Tree peonies often experience environmental stress factors such as heat, drought and low temperature during their growth and development, which may consequently be negatively affected and even lead to plant death. Therefore, the mechanisms of stress resistance in tree peonies and the cultivation of their stress-resistant varieties have attracted increasing attention from researchers (Yang et al., 2024; Ma et al., 2022; Gai et al., 2024; Xu et al., 2017). Due to its paramount importance in various developmental processes and stress responses in tree peonies, studying the NAC gene family in tree peonies is crucial. In this study, members of NAC transcription factor family were identified and performed bioinformatic analyses based on the annotated genome of “Fengdan” peony. Furthermore, “Fengdan” peony was subjected to drought stress, hot stress, and ABA treatments, with RT-qPCR expression analysis conducted on 8 selected PoNAC genes. The results provide insights into the structure and function of PoNAC genes.
Materials and methods
Plant materials and treatments
Three-year-old healthy seedlings of ‘Fengdan’ peony with uniform size without any pest and disease symptoms, grown on the campus of Luoyang Normal University, were transplanted into 30-L pots filled with soil in winter. The potted tree peonies were first placed under natural conditions and then transferred to an indoor plant growth room under a 14-h photoperiod at 25°C, with a light intensity of 25 μmol m-2s-1 and relative humidity of 70%. The application of the ABA treatment and heat and drought stresses was performed separately. Upon the maturity of leaves, plants were placed in the incubator at 42°C to impose heat treatment for 45 days, while the 100μmol/L ABA solution and 20% PEG 6000 solution were sprayed on the leaves of plants in the thermostatic growth room to perform the ABA treatment and simulate drought stress, respectively, and control plants were sprayed with ddH2O. First, leaves were collected at 0 h, 3 h, 6 h, 12 h, and 24 h after the treatments, frozen in liquid nitrogen, and finally stored at –80°C for the subsequent extraction of total RNA and analysis of gene expression. The Total RNA Extraction Kit (Lingjun, Shanghai, China) was used to extract total RNA from ‘Fengdan’peony plants, which was then reverse-transcribed into cDNA using PrimeScript RT Reagent Kit, a reverse transcription kit (TaKaRa, Dalian, China) for a RT-qPCR assay.
Genome-wide identification of NAC gene family members in ‘Fengdan’peony and prediction of protein subcellular localization
All ‘Fengdan’peony genome data were obtained from the China National GeneBank DataBase (CNGBdb) (https:db.cngb.org/search/assembly/CAN0050666), while the genomic data for Arabidopsis thaliana were derived from the Arabidopsis Information Resource (TAIR) (https://www.arabidopsis.org/). The Hidden Markov Model (HMM) profile of the NAM domain (PF02365) was retrieved from the InterPro database (https://www.ebi.ac.uk/interpro/) to identify NAC genes from the ‘Fengdan’ peony genome using the HMMER 3.3.2 software, and thereafter, the generated candidate proteins were filtered based on a score value of higher than 50 and an E-value of lower than 1 × 10−5 by NCBI Conserved Domain Search (CD-Search) (https://www.ncbi.nlm.nih.gov/Structure/bwrpsb/bwrpsb.cgi) and SMART (http://smart.embl-heidelberg.de). To mine the NAC family members of ‘Fengdan’ peony, the proteins without the NAM domains and repeats were manually deleted. The ExPASy ProtParam tool (http://web.expasy.org/protparam/) (Gasteiger et al., 2003) and WoLF PSORT (https://wolfpsort.hgc.jp/) (Horton et al., 2006) were used to predict the subcellular localization and physical and chemical properties of the identified NAC proteins.
Analysis of chromosomal localization, duplication events, and Ka/Ks of NAC gene family members in ‘Fengdan’ peony
Data on the chromosomal location of NAC genes were obtained from the genome GFF3 file of ‘Fengdan’ peony. TBtools software (Chen et al., 2023) was used for the analysis of duplication events of NAC genes in ‘Fengdan’ peony and visual display. To calculate the non-synonymous (Ka) and synonymous (Ks) substitution rates for duplicated genes, KaKs_Calculator (Zhang, 2022) was used.
Phylogenetic analysis of the NAC gene family
To further explore the phylogenetic relationships among ‘Fengdan’ peony NAC gene family members, the phylogenetic tree of NAC proteins from P. ostii and A. thaliana was constructed in the MEGA-11.0 software (Sudhir et al., 2018) using the neighbor-joining (NJ) method with 1000 bootstrap replicates and then edited with the Interactive Tree of Life online tool (iTOL; https://itol.embl.de/). All PoNAC proteins were classified according to the branching and classification standards of ANAC proteins from Arabidopsis (Ooak et al., 2003).
Analysis of the gene structure, conserved motifs, and conserved domains of the NAC gene family
To further clarify the evolutionary relationships among PoNAC gene family members, and predict the conserved sequence of NAC proteins in ‘Fengdan’ peony, the MEME online tool ( https://meme-suite.org/meme/tools/meme ) (Timothy et al., 2015) with 15 motifs was used, and the Hit Data File was obtained from the NCBI Batch CD-Search (http://www.ncbi.nih.gov) (Marchler-Bauer et al., 2015), while the NAC gene structure in ‘Fengdan’ peony was analyzed using the organized genome GFF3 file. Tbtools was used to visualize the conserved motifs, conserved domains, and gene structure (Chen et al., 2023).
Gene ontology enrichment analysis
Gene ontology (GO) functional annotation of the PoNAC genes was performed based on three distinct aspects, including cellular component, molecular function, and biological process using the WEGO online tool (https://wego.genomics.cn/) (Ye et al., 2018).
Analysis of transcript abundance of the NAC gene family in different tissues of ‘Fengdan’ peony
The raw data of the expression of NAC genes in various tissues of tree peony, such as seeds, leaves, and buds were explored based on the genomic data from previous studies (Zhang et al., 2020). Thereafter, to present the results, a heatmap was generated by TBtools using the gene log2 (FPKM+1) values, which represent the gene expression levels (Chen et al., 2023).
RT-qPCR analysis
The primers presented in Supplementary Table 1 were designed using the GenScript online website (https://www.genscript.com/tools/real-time-pcr-taqman-primer-design-tool), while the analysis of gene expression by RT-qPCR was performed with three technical replicates using ubiquitin (Wang et al., 2012) as an appropriate reference gene. Each qPCR reaction (20 µl) containing 10 µl TB Green Premix Ex Taq II (2×), 2µl cDNA as template, 0.5 µl forward primer, 0.5 µl reverse primers, and 7 µl nuclease-free water was run under the conditions and according to the procedures described in previous studies (Guo X, et al., 2024). To quantify the expression levels of PoNAC genes, the 2−ΔΔCt method was used, and data were analyzed with analysis of variance (ANOVA) using SPSS 26.0 and visualized with Graphpad Prism 9.3.
Results
Identification of PoNAC genes in ‘Fengdan’ peony
In this study, based on the whole-genome data of ‘Fengdan’ peony, 82 NAC proteins were identified and then named PoNAC1-PoNAC82 according to the number of gene sequences, and their characteristics are provided in Supplementary Table 2. These proteins encoded by the PoNAC genes contained amino acids within the range of 110 (PoNAC32) to 726 (PoNAC5), and their molecular weights (MW) ranged from 12.72 (PoNAC5) to 83.09 KDa (PoNAC32), with an average value of 39.71 KDa. The isoelectric points (pI) of these proteins varied from 4.75 (PoNAC21) to 9.99 (PoNAC25) (average value of 7.22). Except for PoNAC31, all PoNAC proteins had the negative grand average of hydropathicity index (GRAVY), indicating that they were hydrophilic proteins. Furthermore, the prediction of subcellular localization of these proteins revealed that they were mostly present in the nucleus (46), followed by cytoplasm (15) and chloroplast (10).
Chromosome mapping and analysis of duplication events and evolutionary selection pressure of PoNACs
A total of 4 genes (PoNAC5, PoNAC65, PoNAC12, and PoNAC81) were found to be located on unchr_scaffold_974, unchr_scaffold_5036, and unchr_scaffold_2044. Figure 1A shows the distribution of 78 PoNAC genes on different chromosomes. The chromosomes 1, 2, 3, 4, and 5 contained 16 (20.51%), 19 (24.3%), 14 (17.95%), 15 (19.23%), and 14 (17.95%) PoNAC genes, respectively, which were mainly distributed at both ends, but less in the middle of each chromosome.
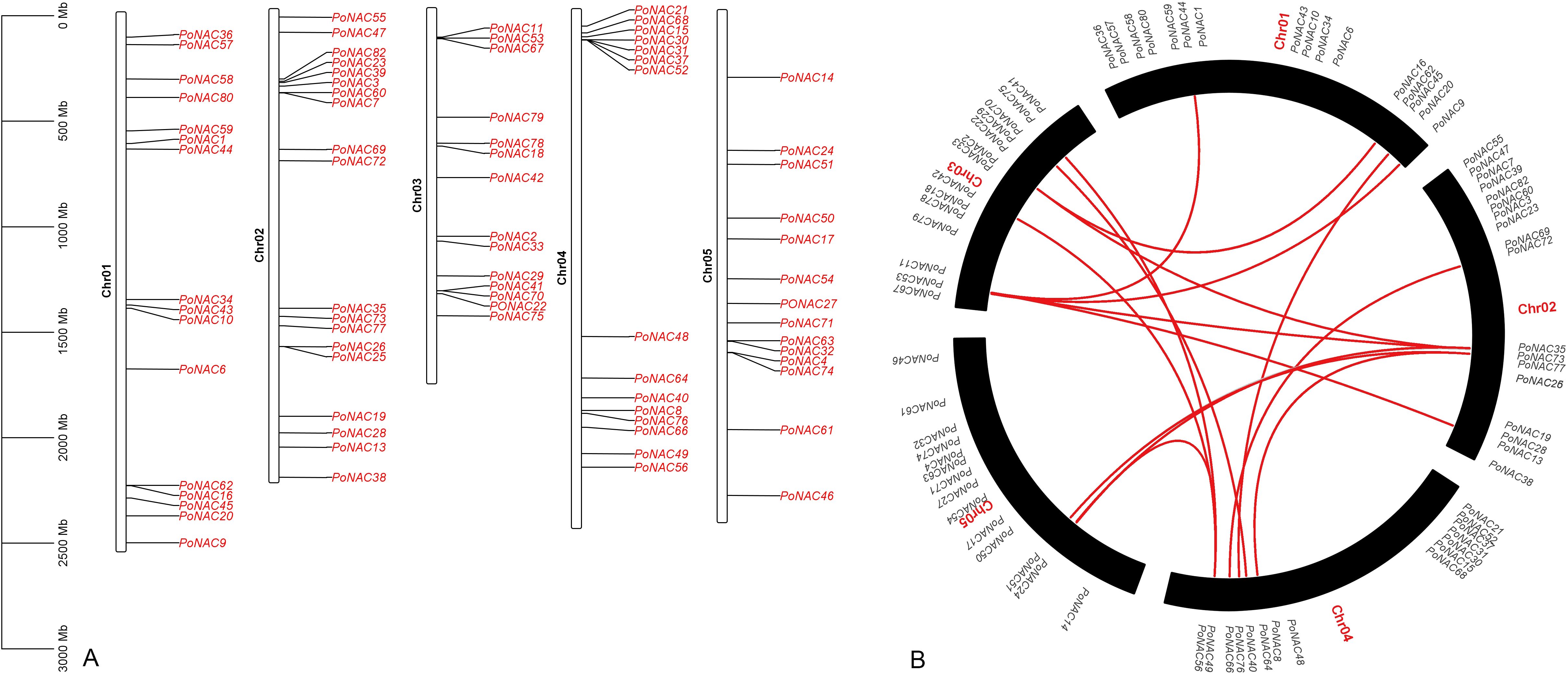
Figure 1. Chromosomal distribution and duplication events of PoNAC genes in ‘Fengdan’ peony. (A) Localization of PoNAC genes on chromosomes. The scale bar on the left indicates the length (Mb) of chromosomes (B) Duplication events of PoNAC genes. The red line in the circle indicates the collinearity gene pair.
To facilitate the analysis of gene duplication events in tree peonies, all unchr_scaffolds were deleted from the ‘Fengdan’ peony genome, and only the information carried in 5 chromosomes was retained (Figure 1B). A total of 19 pairs of gene duplication events were detected in ‘Fengdan’ peony during its evolution, of which 14 pairs underwent whole-genome or segmental duplication, while 2 pairs(PoNAC12-PoNAC81 and PoNAC31-PoNAC37) were tandemly duplicated genes, and 3 pairs including PoNAC25-PoNAC26, PoNAC30-PoNAC52, and PoNAC41-PoNAC70 experienced proximal duplication.
The selection pressure of genes undergoing duplication events was estimated based on the Ka (non-identical)/Ks (identical) values, which were calculated using the Ka/Ks calculator software (Supplementary Table 3). The Ks values of PoNAC gene pairs ranged from 0.00966144 to 4.02808, with 15 pairs (78.9%) having Ks values of above 1. The Ka/Ks values of 18 pairs (94,7%), however, were below 1, indicating the evolution of these genes under strong purifying selection. Only 1 pair (Ka/Ks > 1) might have undergone evolution and functional changes under positive selection after duplication.
Analysis of phylogenetic relationships of PoNAC gene family members in ‘Fengdan’ peony
The phylogenetic tree was constructed by combining 82 proteins from ‘Fengdan’ peony with 105 ANAC proteins from Arabidopsis using MEGA11.0 software (Figure 2). These 82 PoNACs in ‘Fengdan’ peony were further divided into 15 subfamilies including ONAC003 (7), ANAC063 (1), TERN (3), ONAC022 (14), SENU5 (2), NAP (5), ANAC3 (3), ATAF (5), NAC2 (5), ANAC011 (8), TIP (2), OsNAC8 (2), OsNAC7 (7), NAC1 (2), and NAM (4) according to the phylogenetic tree. However, the ANAC001 subfamily contained no ‘Fengdan’ peony PoNACs. Since PoNAC protein sequences and unclassified protein sequences from Arabidopsis were phylogenetically clustered, 16 PoNAC protein sequences could not be classified into subfamilies temporarily. Figure 2 shows some specific NAC subfamilies in both Arabidopsis and ‘Fengdan’ peony, most of which are common among the two.
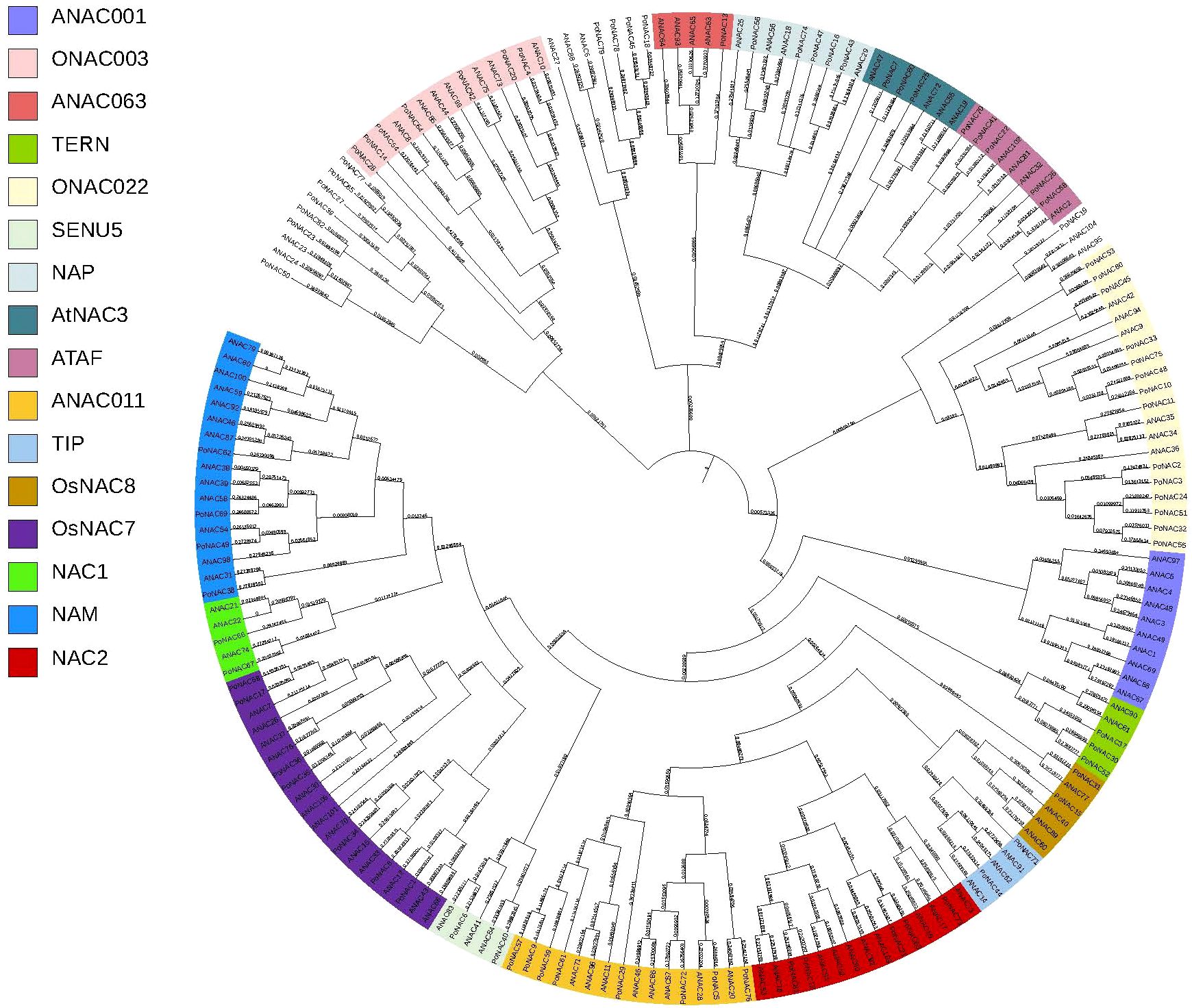
Figure 2. A phylogenetic tree of NAC proteins from ‘Fengdan’peony and Arabidopsis. The colors on the left represent different subfamilies.
The gene structure and motif analysis of PoNAC genes
The analysis of PoNAC gene family members’ conserved motifs, domains, and exon-intron structures was performed. A phylogenetic tree was reconstructed from evolutionary data using the neighbor-joining (NJ) method based on the multiple sequence alignment for the PoNAC gene family (Figure 3A). A total of 15 motifs representing the structural similarity and diversity of NAC proteins in ‘Fengdan’ peony were discovered using the MEME program (Figure 3B). The length of these conserved motifs varied from 11 to 50 amino acids, among which at least 2-8 were present in all 82 PoNAC proteins, and these motifs and phylogenetic relationships showed consistent results. Members having similar conserved motifs grouped in the same phylogenetic clades may also share similar functions. Moreover, all PoNAC proteins contained a conserved NAM domain, which is a specific conserved domain of the NAC gene family, indicating the reliable identification of the genes (Figure 3C). To further examine the structural characteristics of the NAC gene family in tree peonies, the distribution of exons and introns per PoNAC gene was visualized (Figure 3D). All PoNAC genes had a number of introns varied from 0 to 14, and gene members within the same subfamily exhibited a similar number of introns. Among them, ONAC003 subfamily members had 4-6 introns, while NAP subfamily members possessed 2 introns, and 1-2 introns were present in ATAF subfamily members. The existence of introns causes the structural diversity of NAC genes and their functional diversification. The analysis results of phylogenetic relationships, conserved motifs, conserved domains, and gene structure indicated that PoNACs remained highly conserved throughout the long evolutionary process.
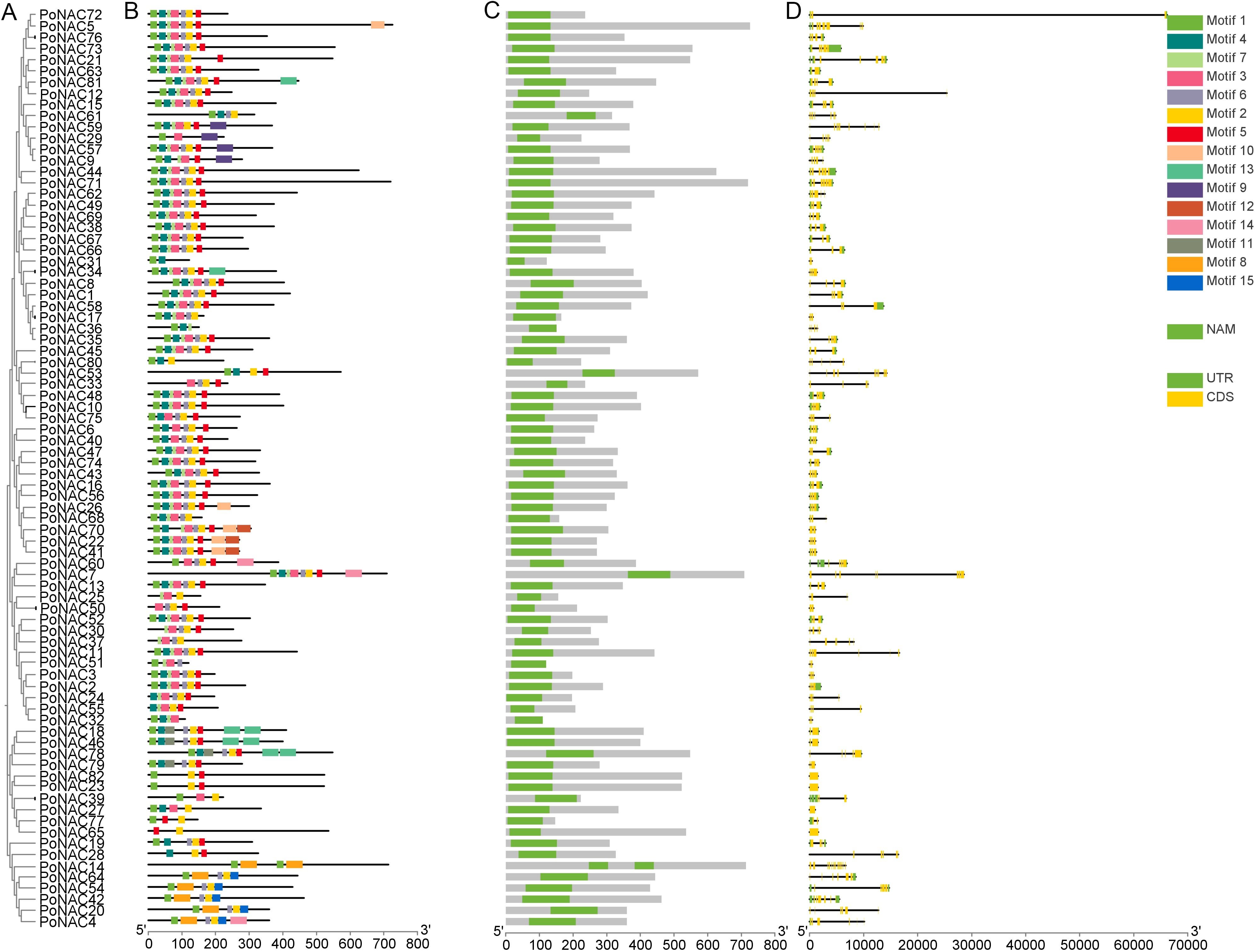
Figure 3. Classification, conserved motifs, and gene structure analysis of PoNAC. (A) Phylogenetic relationship of PoNAC based on NJ method. (B) Motif distribution of PoNAC proteins, different motifs are represented by different colors, ranging from1 to 15. (C) Conserved domain organization in PoNAC proteins. (D) Exon-intron structures of PoNAC genes.
Gene ontology annotation
A total of 193 different GO annotation classes of PoNAC genes have been found (Figure 4), of which 11 are “molecular function” terms, mainly associated with the nucleus (nucleus), organelles (organelle, intracellular membrane-bounded organelle, intracellular anatomical structure, obsolete intracellular part, membrane-bounded organelle, and intracellular organelle) and binding (organic cyclic compound binding, heterocyclic compound binding, nucleic acid binding, and double-stranded DNA binding). On the other hand, the “cellular component” comprised 7 predominant categories mainly involved in DNA binding (DNA binding, DNA-binding transcription factor activity, sequence-specific DNA binding, and sequence-specific double-stranded DNA binding) and transcription regulation (transcription cis-regulatory region binding, transcription regulator activity, and transcription regulatory region nucleic acid binding). The remaining 175 classes belong to the subcategory “biological processes”, which is mainly related to cell division (positive regulation of asymmetric cell division, somatic stem cell division, regulation of asymmetric cell division, and stem cell division), organ development (positive regulation of leaf senescence, positive regulation of leaf development, root cap development, formation of plant organ boundary, formation of anatomical boundary, regulation of leaf senescence, and meristem initiation), flavonoid biosynthesis (regulation of flavonoid biosynthetic process), and stress responses (regulation of defense response to fungus, and positive regulation of response to water deprivation).
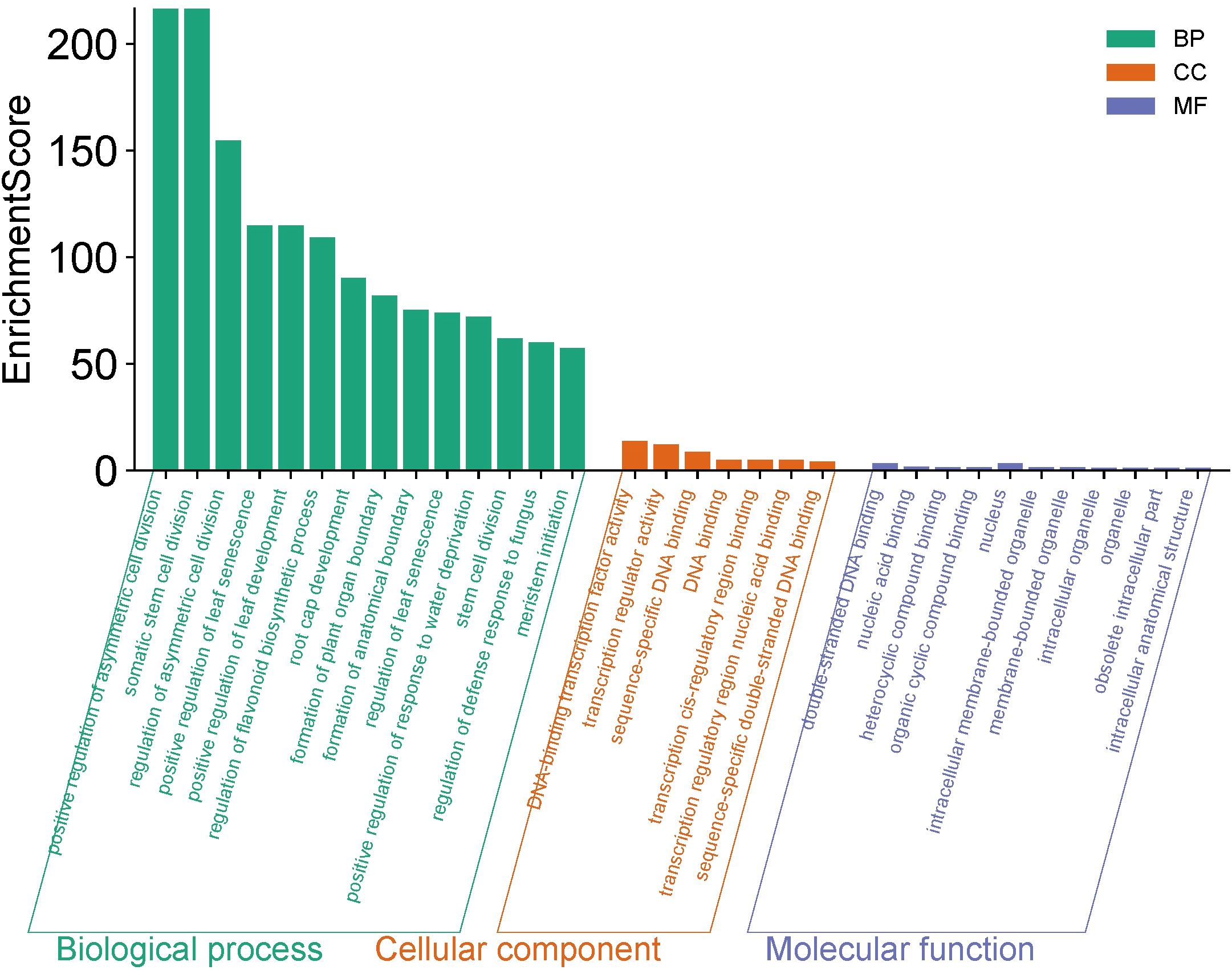
Figure 4. The GO annotation of PoNAC genes in ‘Fengdan’ peony. Biological process (BP) was marked in green, cellular component (CC) in red and molecular function (MF) in blue.
Expression profiles of PoNAC genes in different tissues
Figure 5 presents the expression profiles of PoNAC genes investigated in 6 different tissues based on previous RNA-Seq data. The results showed the differential expression of only 45 in at least one tissue out of all 82 identified PoNAC genes. Cluster analysis of genes according to their expression profiles in different tissues revealed that they were divided into 8 groups, of which 4 groups(I, II, IV and VII) exhibited tissue specificity expression patterns, while 3 group (III, V, VI) were either not expressed in all tissues or expressed at extremely low levels, and 1 group (VIII)displayed expression in all tissues but with no obvious regularity of expression levels. 12 PoNAC genes, highly expressed in all tested tissues, included PoNAC6, PoNAC12, PoNAC68, etc., indicating that they may be the key regulators of plant growth and development. High levels of the expression of PoNAC56 were observed in petals, whereas PoNAC6, PoNAC12, PoNAC68, and PoNAC71 were highly expressed in pistils, and PoNAC6 and PoNAC68 were expressed at high levels in leaves. Furthermore, PoNAC6 and PoNAC12 achieved high expression levels in buds, while the high expression of PoNAC48, PoNAC56, PoNAC6, and PoNAC26 was obtained in seeds, and PoNAC39 and PoNAC56 showed stamen-specific high expression, indicating their important roles in the development of different organs. All these tissue-specific PoNAC genes are considered potential targets for further studying their regulatory functions.
Expression analysis of PoNAC genes in ‘Fengdan’ peony under abiotic stresses and ABA treatment
To investigate the expression patterns and potential functions of PoNAC genes, 8 PoNAC genes associated with stress responses from the ATAF and NAP subfamilies were selected, and their expression levels were detected under hormone treatment (ABA) and abiotic stresses (heat and drought) using RT-qPCR (Figure 6). Upon the ABA treatment, all these 8 PoNAC genes showed upregulation, with the maximum upregulation achieved for PoNAC70 after 24 hours of the treatment, being 103 times higher than that observed for control. Except for a decrease in the expression level of PoNAC16 between 0 and 12 hours of heat treatment, the peak of the expression level of other genes occurred at 12 h. A significant upregulation in the expression level of PoNAC68 was noted, showing a 14-fold increase compared to that of the control group at 12 h. Under drought stress, the expression of PoNAC26 and PoNAC41 underwent little change within 24 h, while significant changes were recorded for other genes. A highly significant up-regulation trend was obtained for PoNAC68 at 3 hours after drought stress, and its expression level was 37 times higher than that of the control group.
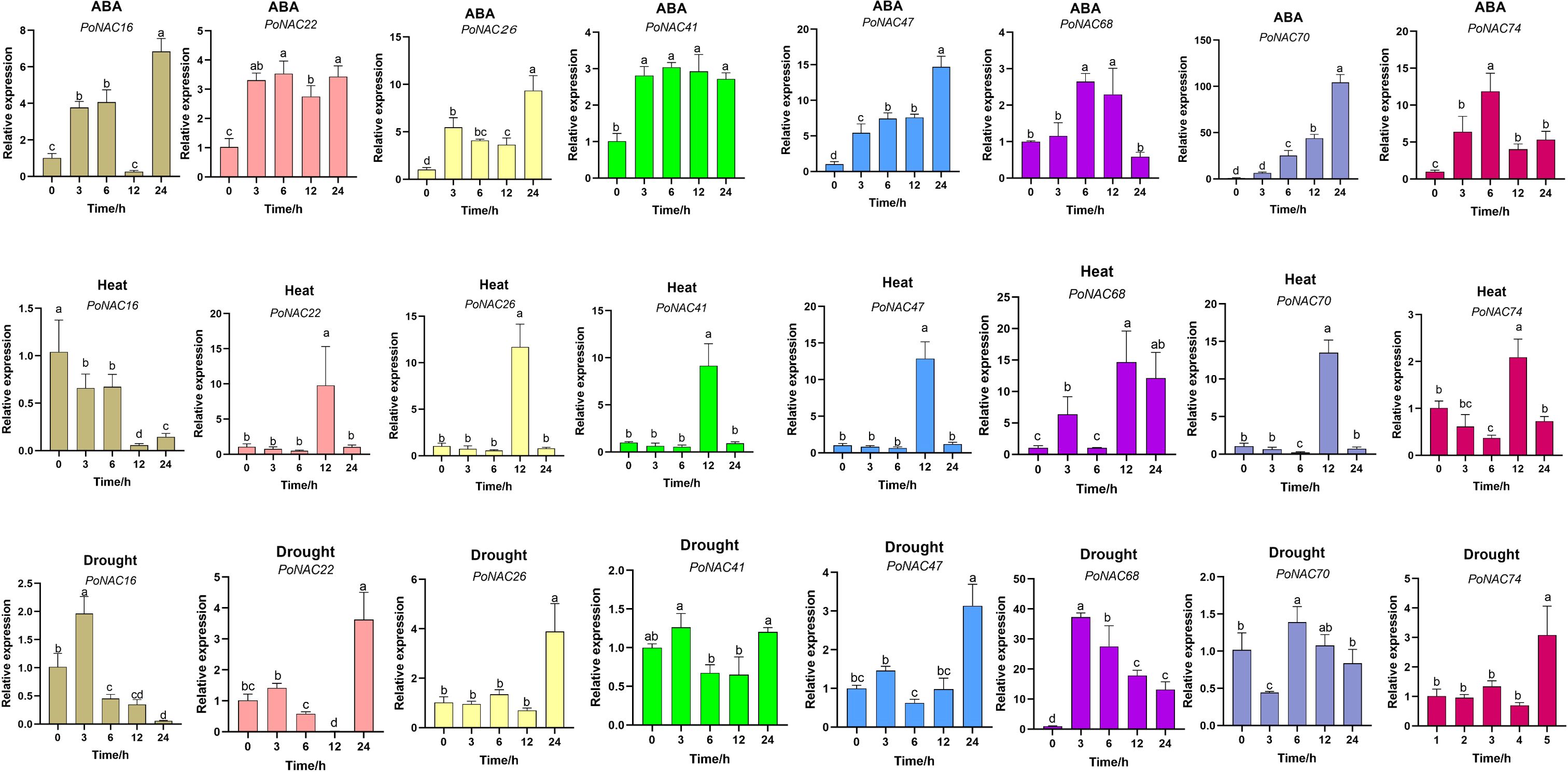
Figure 6. Expression levels of candidate PoNAC genes in the leaves of ‘Fengdan’ peony under different treatments. Error bars represent standard errors (SE) of three biological replicates. Different lowercase letters indicate statistically significant differences among means of treatments (P<0.05).
In conclusion, the PoNAC genes experienced significant upregulation of their expression under different treatments, and PoNAC68 was likely a major regulatory gene for abiotic stress responses in tree peonies.
Discussion
The NAC transcription factor family is of paramount importance in response to abiotic stresses (Nuruzzaman et al., 2013). NAC family members appear to have arisen in response to abiotic stresses in terrestrial plants during their transition from aquatic to terrestrial lifestyles (You et al., 2015) but have not yet been detected in multicellular algae. In this study, 82 PoNAC gene family members were identified based on the whole-genome data of tree peonies, which were higher in number than those found in Scutellaria baicalensis (56) (He et al., 2023), Broussonetia papyrifera (76) (Guo L. et al., 2024), and chickpea (72) (Singh et al., 2021), but lower than those in peanut (166) and pigeon bean (96) (Singh et al., 2021), Arabidopsis (117) and rice (151) (Ooak et al., 2003), oat (333) (Xu et al., 2024), and salvia miltiorrhiza (84) (Zhang et al., 2021). The variation in the number of NAC family members among species reflects the evolutionary divergence between plants.
A total of 19 pairs of PoNAC duplicated genes were identified, among which 14 pairs underwent WGD or segmental duplication, while 3 pairs were proximal duplicated genes, and 2 pairs were tandemly duplicated. It is speculated that all three types of duplication events, which have contributed to the expansion of the NAC family in ‘Fengdan’ peony, helping the plant to resist various stresses and adapt to constantly changing environments, mainly occurred in ONAC022, OSNAC7, and ANAC011 subfamilies. PoNAC35 and PoNAC72 in the OsNAC7 subfamily underwent WGD or segmental duplications with multiple copies, potentially driving the expansion of the PoNAC family. After gene duplication events, PoNAC35 and PoNAC72 retained their original function, while other gene copies could accumulate mutations without losing their functions, following which new gene regulatory networks arise and new functions and gene regulatory mechanisms evolve, which facilitate the better adaptation of ‘Fengdan’ peony to their environment (He and Zhang, 2005).
Each of these 82 PoNAC proteins, which were identified in ‘Fengdan’ peony in the present study and then categorized into 15 subfamilies, had their own unique functions. NAM subfamily members in Arabidopsis are crucial for floral organ development, regulating the flowering time, and meristem maintenance (Collinge and Boller, 2001). The high expression of PoNAC56 in the NAM subfamily in petals, stamens, and seeds leads to the potential regulation of floral organ development and the formation of floral apical meristems in tree peonies. NAC proteins in ATAF, NAP, and OsNAC003 subfamilies may participate in stress response processes (Ooak et al., 2003). PoNACs in these subfamilies, on the other hand, could perform vital functions in tree peonies, such as growth regulation and stress responses. PoNAC68 in the ATAF subfamily is homologous to ATAF1 (ANAC2), an NAC transcription factor in Arabidopsis. It is anticipated that besides response to biotic and abiotic stresses, PoNAC68 has various fundamental roles in seed development, fruit maturation, promotion of leaf senescence and cotyledon opening, and enhancement of tolerance to salt stress in plants (Han et al., 2023).
Upon the exposure of plants to external stimuli, this information is transmitted to stress response systems through signal transduction pathways, which activate the plant’s resistance responses, thereby mitigating the damage caused by stress. NAC genes are of great importance in these processes and can be expressed in large quantities at high temperatures. For example, the expression of SmNAC19 and SmNAC75 in eggplant occurred at high levels after 3 h of heat stress (Wan et al., 2021), while the expression of NAC genes in ryegrass reached its peak at 24 or 48 hour post heat stress (Nie et al., 2020). NAC transcription factors improve plant tolerance to heat stress potentially through two pathways (the pathway of activation of HSF and HSPs, and the one of salicylic acid biosynthesis). In Arabidopsis, RCF2, the CBF gene regulator, transfers heat signal through dephosphorylation of NAC019, which subsequently binds to downstream of HSF promoters, leading to the activation of the expression of downstream of HSF and HSPs, and consequently helps the plant resist heat stress (Guan et al., 2014). SIJA2 in tobacco could not only regulate the expression of the salicylic acid degradation gene but also reduce the accumulation of salicylic acid (SA), which may have been involved in heat stress response through the salicylic acid biosynthesis pathway (Liu et al., 2017). The variation of expression patterns of the selected 8 PoNAC genes occurred under heat stress, among which the expression of 6 PoNAC genes was rapidly induced within 0-12 hours, followed by a gradual decrease after 24 hours, while that of the remaining PoNAC genes underwent an initial decrease and then increased, indicating the quick response of peonies to heat stress. However, the regulatory mechanisms of PoNAC genes underlying heat stress response remain to be further investigated.
The gene duplication and functional diversification of NAC genes in plants during their evolution cause significant differences in their responses to stress. The ABA-dependent pathway is involved in drought stress response in plants (Yoshida et al., 2014). In Arabidopsis under drought stress, AtNAC016 not only directly binds to the promoter of AREB1 and causes the subsequent inhibition of its transcription but also directly targets AtNAP, which leads to the simultaneous regulation of AtAREB1. Although mutants of AtNAC016 and AtNAP exhibited stronger drought resistance, reduced drought tolerance was observed in plant lines overexpressing them (Sakuraba et al., 2015). Meanwhile, apart from the direct activation of the transcription of the genes involved in GA inactivation (SlGA2ox3) and SA synthesis (SlPAL3), SlNAP also improved the drought resistance of tomatoes by activating the ABA synthesis gene SlNCED1 (Wang et al., 2020). The increased drought resistance is also accompanied by reduced accumulation of reactive oxygen species (ROS) in plants. In rice, besides indirectly regulating PCD by activating OsAP37 to stimulate caspase activity, OsNAC2 suppresses OsCOX11 to reduce ROS accumulation, which leads to enhanced drought resistance in the plant (Li et al., 2022). Under drought stress, PoNAC26, PoNAC41, and PoNAC74, which lacked the ABA-responsive element (ABRE) promoter, were expressed at lower levels, while the others containing the ABRE promoter exhibited higher expression levels, implying that the expression of PoNAC in ‘Fengdan’ peony may be controlled through the ABA-dependent pathway.
Conclusions
In summary, a total of 82 PoNAC gene family members were identified from the genome of the ‘Fengdan’ peony and then classified into 15 subfamilies based on the phylogenetic analysis. PoNAC genes were relatively conserved while showing structural variation during the evolution. Additionally, duplication events occurred for 19 PoNAC gene pairs, which might drive the expansion of the NAC family in ‘Fengdan’ peony. The GO analysis results suggested that PoNAC genes were mostly concentrated in the “biological process” category. The differential expression patterns of 45 PoNAC genes in different tissues were observed, indicating their roles in the growth and development of some plant tissues. Meanwhile, the expressions of PoNAC genes were induced in response to the ABA treatment, and abiotic stresses. Overall, the systematic analysis of the NAC gene family in tree peonies contributed to our further study of the distinct functions of PoNAC genes, which lay a foundation for molecular breeding of tree peonies to enhance their stress resistance.
Data availability statement
The original contributions presented in the study are included in the article/Supplementary Material. Further inquiries can be directed to the corresponding authors.
Author contributions
XY: Conceptualization, Methodology, Project administration, Supervision, Writing – original draft, Writing – review & editing. QM: Formal analysis, Investigation, Software, Writing – original draft. HH: Project administration, Resources, Validation, Writing – review & editing. QG: Data curation, Formal analysis, Project administration, Writing – original draft. QW: Software, Validation, Writing – review & editing. YY: Resources, Software, Validation, Writing – review & editing. YZ: Conceptualization, Formal analysis, Project administration, Resources, Validation, Visualization, Writing – review & editing.
Funding
The author(s) declare that no financial support was received for the research and/or publication of this article.
Conflict of interest
The authors declare that the research was conducted in the absence of any commercial or financial relationships that could be construed as a potential conflict of interest.
Generative AI statement
The author(s) declare that no Generative AI was used in the creation of this manuscript.
Publisher’s note
All claims expressed in this article are solely those of the authors and do not necessarily represent those of their affiliated organizations, or those of the publisher, the editors and the reviewers. Any product that may be evaluated in this article, or claim that may be made by its manufacturer, is not guaranteed or endorsed by the publisher.
Supplementary material
The Supplementary Material for this article can be found online at: https://www.frontiersin.org/articles/10.3389/fpls.2025.1559667/full#supplementary-material.
Abbreviations
NAC, NAM, ATAF, CUC2; NAM, no apical meristem; CUC, cup-shaped cotyledon; TF, transcription factor; SalAs, salvianolic acids; GB, glycine betaine; FPKM, Fragments Per Kilobase of exon model per Million mapped reads; RT-qPCR, Quantitative real-time polymerase chain reaction; GO, Gene ontology; PCD, Programmed cell death.
References
Aida, M., Ishida, T., Fukaki, H., Fujisawa, H., Tasaka, M. (1997). Genes involved in organ separation in Arabidopsis: an analysis of the cup-shaped cotyledon mutant. Plant Cell. 9, 841–857. doi: 10.1105/tpc.9.6.841
Alshareef, N. O., Otterbach, S. L., Allu, A. D., Woo, Y. H., Werk, T., Kamranfar, I., et al. (2022). NAC transcription factors ATAF1 and ANAC055 affect the heat stress response in Arabidopsis. Sci. Rep. 12, 11264. doi: 10.1038/s41598-022-14429-x
Cai, W., Yang, S., Wu, R., Cao, E., Shen, L., Guan, D., et al. (2021). Pepper NAC-type transcription factor NAC2c balances the trade-off between growth and defense responses. Plant Physiol. 186, 2169–2189. doi: 10.1093/plphys/kiab190
Chen, C., Wu, Y., Li, J., Wang, X., Zeng, Z., Xu, J., et al. (2023). TBtools-II: A “one for all, all for one” bioinformatics platform for biological big-data mining. Mol. Plant 16, 1733–1742. doi: 10.1016/j.molp.2023.09.010
Christianson, J. A., Dennis, E. S., Llewellyn, D. J., Wilson, I. W. (2010). ATAF NAC transcription factors: regulators of plant stress signaling. Plant Signal. Behav. 5, 428–432. doi: 10.4161/psb.5.4.10847
Collinge, M., Boller, T. (2001). Differential induction of two potato genes, Stprx2 and StNAC, in response to infection by Phytophthora infestans and to wounding. Plant Mol. Biol. 46, 521–529. doi: 10.1023/A:1010639225091
Duval, M., Hsieh, T. F., Kim, S. Y., Thomas, T. L. (2002). Molecular characterization of AtNAM: a member of the Arabidopsis NAC domain superfamily. Plant Mol. Biol. 50, 237–248. doi: 10.1023/A:1016028530943
Gai, W. L., Liu, C. Y., Yang, M. J., Li, F., Xin, H., Gai, S. P. (2024). Calcium signaling facilitates chilling- and GA- induced dormancy release in tree peony. Front. Plant Science. 15. doi: 10.3389/fpls.2024.1362804
Gasteiger, E., Gattiker, A., Hoogland, C., Ivanyi, I., Appel, R. D., Bairoch, A.. (2003). ExPASy: the proteomics server for in-depth protein knowledge and analysis. Nucleic Acids Res. 31, 3784–3788. doi: 10.1093/nar/gkg563
Guan, Q. M., Yue, X. L., Zeng, H. T., Zhu, J. H. (2014). The protein phosphatase RCF2 and its interacting partner NAC019 are critical for heat stress-responsive gene regulation and thermotolerance in Arabidopsis. Plant Cell. 26, 438–453. doi: 10.1105/tpc.113.118927
Guo, L., Li, Y., Wei, Z., Wang, C., Hou, X. (2023). Reference genes selection of Paeonia ostii ‘fengdan’ under osmotic stresses and hormone treatments by RT-qPCR. Mol. Biol. Rep. 50, 133–143. doi: 10.1007/s11033-022-07938-5
Guo, L., Liao, Y., Deng, S., Li, J., Bu, X., Zhu, C., et al. (2024). Genome-wide analysis of NAC transcription factors and exploration of candidate genes regulating selenium metabolism in Broussonetia papyrifera. Planta. 260, 1. doi: 10.1007/s00425-024-04438-7
Guo, X., Yan, X., Li, Y. (2024). Genome-wide identification and expression analysis of the WRKY gene family in Rhododendron henanense subsp. lingbaoense. Peer J. 12, e17435. doi: 10.7717/peerj.17435
Han, K. J., Zhao, Y., Sun, Y., Li, Y. (2023). NACs, generalist in plant life. Plant Biotechnol. J. 21, 2433–2457. doi: 10.1111/pbi.14161
Hasson, A., Plessis, A., Blein, T., Adroher, B., Grigg, S., Tsiantis, M., et al. (2011). Evolution and diverse roles of the CUP-SHAPED COTYLEDON genes in Arabidopsis leaf development. Plant Cell. 23, 54–68. doi: 10.2307/41433803
He, H., Li, Q., Fang, L., Yang, W. P., Xu, F., Yan, Y., et al. (2023). Comprehensive analysis of NAC transcription factors in Scutellaria baicalensis and their response to exogenous ABA and GA3. Int. J. Biol. Macromolecules. 244, 125290. doi: 10.1016/j.ijbiomac.2023.125290
He, X., Zhang, J. (2005). Rapid subfunctionalization accompanied by prolonged and substantial neofunctionalization in duplicate gene evolution. Genetics. 169, 1157–1164. doi: 10.1534/genetics.104.037051
Horton, P., Park, K. J., Obayashi, T., Nakai, K. (2006). WoLF PSORT: protein localization prediction software. Nucleic Acids Res. 35, W585–W587. doi: 10.1093/nar/gkm259
Jia, D. F., Jiang, Q., van Nocker, S., Gong, X., Ma, F. (2019). An apple (Malus domestica) NAC transcription factor enhances drought tolerance in transgenic apple plants. Plant Physiologh Biochem. 139, 504–512. doi: 10.1016/j.plaphy.2019.04.011
Li, Y., Han, S., Sun, X., Khan, N. U., Zhong, Q., Zhang, Z., et al. (2022). Variations in OsSPL10 confer drought tolerance by directly regulating OsNAC2 expression and ROS production in rice. J. Integr. Plant Biol. 65, 918–933. doi: 10.1111/jipb.13414
Liu, Z. M., Yue, M. M., Yang, D. Y., Zhu, S. B., Ma, N. N., Meng, Q. W. (2017). Over-expression of SlJA2 decreased heat tolerance of transgenic tobacco plants via salicylic acid pathway. Plant Cell Rep. 36, 529–542. doi: 10.1007/s00299-017-2100-9
Ma, J., Wang, Q., Wei, L. L., Zhao, Y., Zhang, G. Z., Wang, J., et al. (2022). Responses of the tree peony (Paeonia suffruticosa, Paeoniaceae) cultivar ‘Yu Hong’ to heat stress revealed by iTRAQ-based quantitative proteomics. Proteome Science. 20, 1–14. doi: 10.1186/s12953-022-00202-5
Marchler-Bauer, A., Derbyshire, M. K., Gonzales, N. R., Lu, S., Farideh, C., Geer, L. Y., et al. (2015). CDD: NCBI’s conserved domain database. Nucleic Acids Res. 43, D222–D226. doi: 10.1093/nar/gku1221
Mccarthy, R. L., Zhong, R., Ye, Z. H. (2011). Secondary wall NAC binding element (SNBE), a key cis-acting element required for target gene activation by secondary wall NAC master switches. Plant Signaling behavior. 6, 1282–1285. doi: 10.4161/psb.6.9.16402
Melo, B. P., Lourenco-Tessutti, I. T., Fraga, O. T., Pinheiro, L. B., Fontes, E. P.B. (2021). Contrasting roles of GmNAC065 and GmNAC085 in natural senescence, plant development, multiple stresses and cell death responses. Sci. Rep. 11, 11178. doi: 10.1038/s41598-021-90767-6
Meraj, T. A., Fu, J., Raza, M. A., Zhu, C., Wang, Q. (2020). Transcriptional factors regulate plant stress responses through mediating secondary metabolism. Genes (Basel). 11, 346. doi: 10.3390/GENES11040346
Nie, G., Yang, X. Y., Yang, Z. F., Zhong, M., Zhu, Y., Zhou, J., et al. (2020). Genome-wide investigation of the NAC transcript factor family in perennial ryegrass (Lolium perenne L.) and expression analysis under various abiotic stressor. Genomics. 112, 4224–4231. doi: 10.1016/j.ygeno.2020.06.033
Nuruzzaman, M., Sharoni, A. M., Kikuchi, S. (2013). Roles of NAC transcription factors in the regulation of biotic and abiotic stress responses in plants. Front. Microbiol. 4. doi: 10.3389/fmicb.2013.00248
Ooak, H., Satoh, K., Doi, K., Nagata, T., Otomo, Y., Murakami, K., et al. (2003). Comprehensive analysis of NAC family genes in Oryza sativa and Arabidopsis thaliana. DNA Res. 10, 239–247. doi: 10.1093/dnares/10.6.239
Sakuraba, Y., Kim, Y. S., Han, S. H., Lee, B. D., Paek, N. C. (2015). The Arabidopsis transcription factor NAC016 promotes drought stress responses by repressing AREB1 transcription through a trifurcate feed-forward regulatory loop involving NAP. Plant Cell. 27, 1771–1787. doi: 10.1105/tpc.15.00222
Sheng, Z. P., Meng, J. S., Tao, J., Zhao, D. (2023). Osmotic regulation, antioxidant enzyme activities and photosynthetic characteristics of tree peony (Paeonia suffruticosa Andr.) in response to high temperature stress. Phyton-international J. Exp. botany. 92, 3133–3147. doi: 10.32604/phyton.2023.028818
Singh, S., Koyama, H., Bhati, K. K., Alok, A. (2021). The biotechnological importance of the plant-specific NAC transcription factor family in crop improvement. J. Plant Res. 134, 475–495. doi: 10.1007/s10265-021-01281-9
Kumar, S., Stecher, G., Li, M., Knyaz, C., Tamura, K. (2018). Mega x: molecular evolutionary genetics analysis across computing platforms. Mol. Biol. 35, 1547–1549. doi: 10.1093/molbev/msy096
Bailey, T. L., Johnson, J., Grant, C. E., Noble, W. S. (2015). The MEME suite. Nucleic Acids Res. 43, W39–W49. doi: 10.1093/nar/gkv416
Wan, F. X., Gao, J., Wang, G. L., Niu, Y., Wang, L. Z., Zhang, X. G. (2021). Genome-wide identification of NAC transcription factor family and expression analysis of ATAF subfamily members under abiotic stress in eggplant. Scientia Horticulturae. 289, 110424. doi: 10.1016/j.scienta.2021.110424
Wang, F., Chen, Y., Yang, R. S., Yang, Q., Li, Z. (2025). Identification of zmSNAC06, a maize NAC family transcription factor with multiple transcripts conferring drought tolerance in arabidopsis. Plants. 14, 12. doi: 10.3390/plants14010012
Wang, H. L., Zhang, Y., Wang, T., Yang, Q., Li, Z. (2021). An alternative splicing variant of PtRD26 delays leaf senescence by regulating multiple NAC transcription factors in Populus. Plant Cell. 33, 1594–1614. doi: 10.1093/plcell/koab046
Wang, J., Zheng, C., Shao, X., Hu, Z., Li, J., Wang, P., et al. (2020). Transcriptomic and genetic approaches reveal an essential role of the NAC transcription factor SlNAP1 in the growth and defense response of tomato. Hortic. Res. 7, 209. doi: 10.1038/s41438-020-00442-6
Wang, X. J., Liang, H. Y., Guo, D. L., Guo, L., Duan, X., Jia, Q., et al. (2019). Integrated analysis of transcriptomic and proteomic data from tree peony (P. ostii) seeds reveals key developmental stages and candidate genes related to oil biosynthesis and fatty acid metabolism. Hortic. Res. 6, 111. doi: 10.1038/S41438-019-0194-7
Wang, Y. J., Dong, L., Zhang, C., Wang, X. Q. (2012). Reference gene selection for real-time quantitative PCR normalization in tree peony (Paeonia suffruticosa Andr.). J. Agric. Biotechnol. 20, 521–528. doi: 10.3969/j.issn.16747968.2012.05.008
Xie, Q., Frugis, G., Colgan, D., Chua, N. H. (2000). Arabidopsis NAC1 transduces auxin signal downstream of TIR1 to promote lateral root development. Genes Dev. 14, 3024–3036. doi: 10.1101/gad.852200
Xu, XX, Cheng, FY, Peng, LP, Xian, HL. Suggestions on Conservation and Utilization of Wild Tree Peony Resources of Subsect. Vagintae Based on Recent Investigation. J Plant Genet Res. (2017). doi: 10.13430/j.cnki.jpgr.2017.01.006
Xu, Y., Cheng, J., Hu, H., Yan, L., Jia, J. Q., Wu, B. (2024). Genome-wide identification of NAC family genes in oat and functional characterization of AsNAC109 in abiotic stress tolerance. Plants. 13, 1017. doi: 10.3390/plants13071017
Yang, X. Y., Huang, Y., Yao, Y. P., Bu, W., Zhang, M., Zheng, T., et al. (2024). Mining heat-resistant key genes of peony based on weighted gene co-expression network analysis. Genes. 15, 383. doi: 10.3390/genes15030383
Yang, Y., Sun, M., Li, S. S., Chen, Q., Wang, L. (2020). Germplasm resources and genetic breeding of Paeonia: a systematic review. Hortic. Res. 7, 107. doi: 10.1038/s41438-020-0332-2
Yarra, R., Wei, W. (2021). The NAC-type transcription factor GmNAC20 improves cold, salinity tolerance, and lateral root formation in transgenic rice plants. Funct. Integr. Genomics 21, 473–487. doi: 10.1007/s10142-021-00790-z
Ye, J., Zhang, Y., Cui, H., Liu, J., Wu, Y., Cheng, Y., et al. (2018). WEGO 2.0: a web tool for analyzing and plotting GO annotations 2018 update. Nucleic Acids Res. 46, W71–W75. doi: 10.1093/nar/gky400
Yoshida, T., Mogami, J., Yamaguchi-Shinozaki, K. (2014). ABA-dependent and ABA-independent signaling in response to osmotic stress in plants. Curr. Opin. Plant Biol. 21, 133–139. doi: 10.1016/j.pbi.2014.07.009
You, J., Zhang, L., Song, B., Qi, X., Chan, Z. (2015). Systematic analysis and identificationof stress-responsive genes of the NAC gene family in Brachypodium distachyon. PloS One 10, e0122027. doi: 10.1371/journal.pone.0122027
Zhan, J., Chu, Y., Wang, Y., Diao, Y., Zhao, Y., Liu, L., et al. (2021). The miR164-Gh-CUC2-GhBRC1 module regulates plant architecture through abscisic acid in cotton. Plant Biotechnol. J. 19, 1839–1851. doi: 10.1111/pbi.13599
Zhang, Z. (2022). KaKs_Calculator 3.0: Calculating selective pressure on coding and non-coding sequences. Genomics Proteomics Bioinf. 3), 3. doi: 10.1016/j.gpb.2021.12.002
Zhang, H., Xu, J., Chen, H., Jin, W., Liang, Z. (2021). Characterization of NAC family genes in Salvia miltiorrhiza and NAC2 potentially involved in the biosynthesis of tanshinones. Phytochemistry. 191, 112932. doi: 10.1016/j.phytochem.2021.112932
Zhang, Y., Xu, S., Cheng, Y., Wang, J., Wang, X., Liu, R., et al. (2020). Functional identification of PsMYB57 involved in anthocyanin regulation of tree peony. BMC Genet. 21, 124. doi: 10.1186/s12863-020-00930-7
Keywords: ‘Fengdan’ peony, NAC transcription factors, bioinformatics, abiotic stresses and ABA treatment, expression analysis
Citation: Yu X, Meng Q, Hou H, Guo Q, Wang Q, Yang Y and Zhang Y (2025) Characterization of the NAC gene family in ‘Fengdan’ peony (Paeonia ostii) insights into the evolution and expression patterns under abiotic stresses and ABA treatment. Front. Plant Sci. 16:1559667. doi: 10.3389/fpls.2025.1559667
Received: 13 January 2025; Accepted: 31 March 2025;
Published: 16 April 2025.
Edited by:
Yuanyue Shen, Beijing University of Agriculture, ChinaReviewed by:
Maria Carmen Valoroso, University of Milan, ItalyYun Huang, Beijing University of Agriculture, China
Copyright © 2025 Yu, Meng, Hou, Guo, Wang, Yang and Zhang. This is an open-access article distributed under the terms of the Creative Commons Attribution License (CC BY). The use, distribution or reproduction in other forums is permitted, provided the original author(s) and the copyright owner(s) are credited and that the original publication in this journal is cited, in accordance with accepted academic practice. No use, distribution or reproduction is permitted which does not comply with these terms.
*Correspondence: Xiangli Yu, eXV4bGkyMDAxQDE2My5jb20=; Yanzhao Zhang, eXpoYW9femhhbmdAMTYzLmNvbQ==