- 1Department of Plant Pathology, Pir Mehr Ali Shah Arid Agriculture University, Rawalpindi, Pakistan
- 2Citrus Research and Education Center (CREC), University of Florida, Lake Alfred, FL, United States
- 3Department of Horticulture, Pir Mehr Ali Shah Arid Agriculture University, Rawalpindi, Pakistan
- 4Institute of Food and Nutritional Sciences, Pir Mehr Ali Shah Arid Agriculture University, Rawalpindi, Pakistan
- 5School of Biochemistry and Biotechnology, University of the Punjab, Lahore, Pakistan
Here we report the effects of aqueous extracts of the plant Moringa oleifera and rhizobacterial strains, Bacillus australimaris (BA-LWD73) and B. thuringiensis (BT-WAG41), applied singly and in combination, on tomato growth and Meloidogyne incognita infection. Plant height, root length, shoot and root biomass (fresh and dry), were significantly increased by most treatments compared to the control. The combined treatment of BA-LWD73 + M. oleifera produced the highest plant height (96.94 cm) and root length (30.48 cm). The highest shoot biomass was observed in BA-LWD73 alone treatment while root biomass was generally lower in all treatments than in the control. M. incognita induced, root gall rating, number of eggs per root system, second stage juveniles (J2), and reproduction rate, were significantly reduced in treatments involving M. oleifera and BA-LWD73, either alone or in combination. The lowest root gall rating (2.67) and J2 count (680) were observed in B. australimaris LWD73 + M. oleifera treatment. FTIR analysis of M. oleifera extract indicated the presence of functional groups such as hydroxyl, C=C, S=O, and C–O, suggesting bioactive potential. GC-MS analysis revealed six phytocompounds, with 5-Hydroxymethylfurfural (71.76%) as the dominant component, all known for antimicrobial and nematicidal activities. Moreover, volatile organic compounds from BA-LWD73 included 1H-Indole (87.46%) and 2-Nonanone (6.54%), known for their nematicidal properties. These findings highlight the potential of rhizobacteria and plant extracts in promoting tomato growth and suppressing M. incognita infection.
1 Introduction
Root-knot nematodes (Meloidogyne spp.) have a devastating impact on vegetable crops worldwide, causing significant yield losses and compromising overall plant health (Ornat et al., 2001; Haq et al., 2022; Yaseen et al., 2023; Shahid et al., 2024). These polyphagous nematodes have an extensive host range, infecting over 3,000 plant species (Kayani et al., 2013; Kayani and Mukhtar, 2018; Hussain and Mukhtar, 2019; Khairy et al., 2021; Saeed et al., 2021, 2023). Upon infection, they induce the formation of root galls, which disrupt normal root functions and impair the ability of plants to efficiently absorb water and nutrients from the soil (Karssen and Moens, 2006; Nazir et al., 2019; Khan et al., 2019; Saeed and Mukhtar, 2024). This leads to stunted growth and reduced crop yields (Mukhtar et al., 2013a; Abbas et al., 2024). Moreover, root-knot nematode infections often predispose plants to secondary infections by soil-borne pathogens, exacerbating damage and further diminishing plant health (Asghar et al., 2020; Ahmed et al., 2021; Yaseen et al., 2024; 2025).
The severity of the damage caused by root-knot nematodes depends on various factors, including environmental conditions, nematode population density, species virulence, and the susceptibility of the host plant (Tariq-Khan et al., 2017, 2020, 2025). Managing these nematodes is particularly challenging due to their high reproductive potential and remarkable adaptability (Hussain et al., 2011; Ayub et al., 2024). Although chemical nematicides have proven effective in controlling root-knot nematodes, their adverse environmental impacts have driven a shift toward developing alternative, management strategies (Yaseen and Mukhtar, 2024).
Tomato (Lycopersicon esculentum L.) is a major crop in Pakistan, cultivated on 68,863 hectares with an average yield of 11.08 t/ha, significantly lower than in China (59.2 t/ha), Turkey (79.3 t/ha), and India (25.1 t/ha) (FAO, 2021). The crop is vulnerable to various diseases caused by fungal, bacterial, viral, and nematode infections, leading to substantial yield losses (Aslam et al., 2017; Hassan et al., 2024; Iqbal et al., 2024; Mustafa et al., 2024; Vagelas, 2024).
Root-knot nematodes pose a significant threat, causing yield losses of up to 29%. In India, nematode-related losses reach $21.7 million, and the Mediterranean region reports losses of 62% (Kumar et al., 2020; Fullana et al., 2023). Pakistan also faces considerable economic damage from root-knot nematodes resulting in reduced plant vigor, chlorosis, stunted growth, and potential crop failure (Oka et al., 2000). Infected plants develop weak root systems, making them easily uprooted (Anwar and Javed, 2010).
The use of biological control agents, primarily bacteria and fungi, presents a promising alternative approach to suppressing nematode populations. Similarly, plant-based natural products have proven effective against various pathogens including root-knot nematodes (Aslam et al., 2024; Atiq et al., 2024; Manzoor et al., 2024; Taha et al., 2024). Incorporating macerated leaves of Cannabis sativa and Azadirachta indica into the soil significantly reduced M. javanica populations and infection severity in plants in a dose-dependent manner (Kayani et al., 2012; Mukhtar et al., 2013b). Likewise, the combined application of dried neem leaves and Trichoderma harzianum on tomato plants increased reductions in root galls, egg mass production, and egg hatching compared to their individual applications. This synergistic effect also enhanced plant height and fresh shoot weight, with outcomes strongly influenced by dosage and exposure time (Azeem et al., 2021).
Alternative nematode management strategies include gene editing, natural nematicides (bacteria, fungi, botanicals), and cultural practices, reducing reliance on chemical controls (Afzal and Mukhtar, 2024). Plant growth-promoting rhizobacteria (PGPR) are essential for enhancing plant growth and mitigating stress through increased mineral availability, phytohormone production, alleviating heavy metal stress, and biocontrol of pathogens leading to better nutrient uptake, growth, and crop yields (Khandagale et al., 2024; Zhang et al., 2024). For sustainable rice and sugarcane production, bacteria from the genera Bacillus, Rhizobium, Comamonas, Cyanobacteria, Nodosilinea, Levinella, and Pseudomonas effectively produce nitrogen and solubilize essential nutrients (Khandagale et al., 2024). A consortium of B. cereus AR156, B. subtilis SM21, and Serratia sp. XY21 has been shown to reduce root-knot disease severity while enhancing cucumber yield and fruit quality (Zhang et al., 2024).
Moringa oleifera has shown promise as a natural nematicide, with its bioactive compounds disrupting nematode life cycles and improving plant vigor and yield. When combined with beneficial rhizobacteria, M. oleifera enhances nematode suppression, offering an eco-friendly approach to nematode management (Geldenhuys, 2023; Aminisarteshnizi, 2024).
The present study, therefore, aims to evaluate the nematicidal potential of M. oleifera, both alone and in combination with PGPR, to identify effective, alternative solutions for managing Meloidogyne incognita in tomato.
2 Materials and methods
2.1 Inoculum preparation of nematode
Meloidogyne incognita culture, maintained in the Citrus Research and Education Center (CREC), University of Florida, USA, was initiated from a single egg mass on a susceptible tomato host (cv. HM1824) and identified based on perineal pattern morphology (Taylor and Netscher, 1974). Infected root fragments were shaken in 0.5% sodium hypochlorite (NaOCl) to release eggs. The suspension was filtered through a 200-mesh sieve to remove debris and a 500-mesh sieve to collect eggs. The eggs were rinsed three times with tap water to eliminate residual bleach. Freshly hatched second-stage juveniles (J2) were obtained by incubating eggs at 25°C for 48 h and used as inoculum (Kayani et al., 2018).
2.2 Preparation of aqueous extracts of M. oleifera
Fresh M. oleifera leaves were washed, blended in sterile water, and left for 12 h. The mixture was filtered sequentially through muslin cloth, Whatman No. 1 filter paper, and a Millipore filter. Different extract concentrations (0 to 100%) were prepared by dilution with distilled water (Mukhtar et al., 2013c, d).
2.3 Isolation and purification of rhizobacteria
Rhizosphere soil from tomato plants was collected, and rhizobacteria were isolated via serial dilution (Shahzaman et al., 2015; Aziz et al., 2024; Aslam et al., 2025). Dilutions (10-5, 10-6 and 10-7) were plated on nutrient agar (NA) and incubated at 26 ± 2°C. After 24 h, individual colonies were purified by streaking (Aslam and Mukhtar, 2023a, b, 2024).
2.4 Molecular characterization of rhizobacterial isolates
Genomic DNA was extracted using a commercial kit. The 16S rRNA gene was amplified via PCR with universal primers 27F and 1492R (Kumar et al., 2018). PCR conditions: initial denaturation (94°C, 3 min); 35 cycles of 94°C (40 s), 60°C (50 s), 72°C (1 min); final extension (72°C, 10 min). Amplified DNA was visualized, purified, quantified (NanoDrop), and sequenced (Eurofins Genomics, USA).
2.5 Sub-culturing and preparation of rhizobacterial suspensions
Identified strains were cultured on NA, transferred to Luria Bertani broth, and incubated at 25°C (48 h, rotary shaker). Cell suspensions were adjusted to OD600 = 1.0 (109 CFU/mL), centrifuged (5000 rpm, 15 min, 4°C), washed, and resuspended in sterile water (Nikoo et al., 2014).
2.6 Effect of rhizobacteria and plant extracts on plant growth and M. incognita infection
Tomato plants (21-day-old) were inoculated with 5000 J2 M. incognita (5 mL suspension). After 48 h, 50 mL of rhizobacterial suspension (109 CFU/mL) or M. oleifera extract was applied via soil drench (Mukhtar et al., 2013b). Controls received water. Six replicates per treatment were maintained (Table 1).
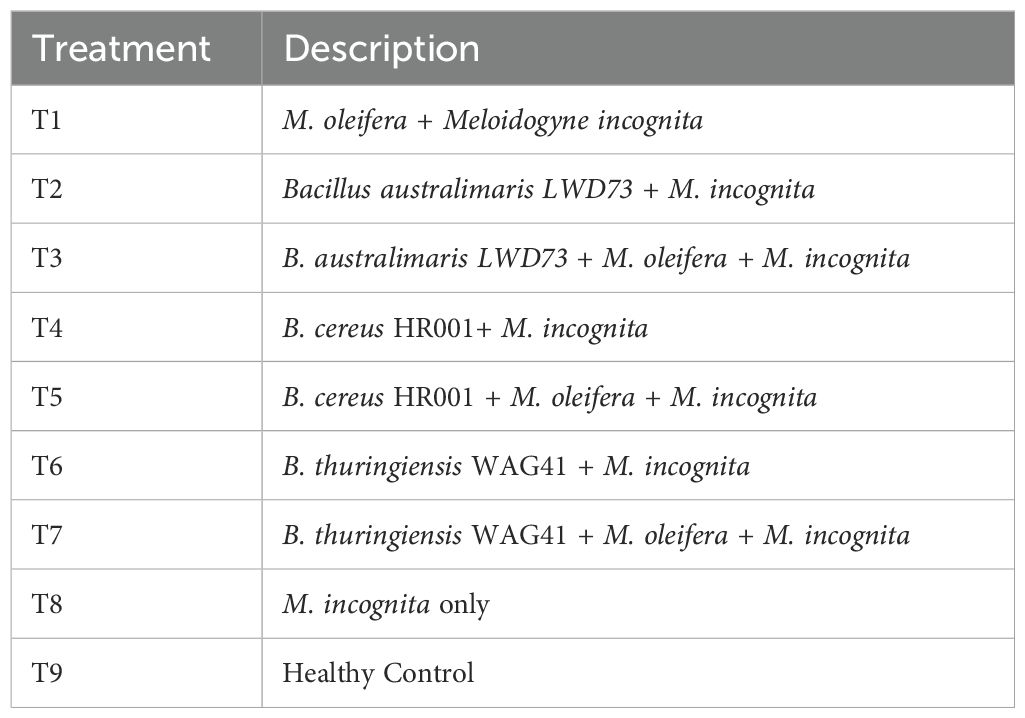
Table 1. Treatments involving rhizobacteria and moringa leaf extracts for assessing plant growth and Meloidogyne incognita infection.
After 45 days, growth parameters (plant height, root/shoot length, fresh/dry weights) and infection parameters (root gall rating, eggs per root, J2/100 cc soil, nematode reproduction factor) were assessed (Mukhtar et al., 2021).
2.7 Detection of volatile organic compounds from rhizobacteria
Bacilllus australimaris LWD73 was cultured overnight at 37°C in LB broth. The bacterial culture was adjusted to an optical density (OD) of 0.132 at 600 nm (0.5 McFarland units) using a spectrophotometer. A 5 mL aliquot was transferred to a headspace (HS) vial, and a Solid Phase Microextraction (SPME) fiber was inserted. The HS vial was incubated in a water bath at 60°C for 60 min. After incubation, the SPME fiber was immediately exposed to the GC injection port for 28 min. Each treatment was replicated nine times (Tait et al., 2014).
2.8 Compound identification from plant extracts
Nematicidal compounds in aqueous M. oleifera extracts were identified using Fourier Transform Infrared Spectroscopy (FTIR) (Elzey et al., 2016) and Gas Chromatography-Mass Spectrometry (GC-MS) (Ahmed et al., 2024).
2.9 Statistical analysis
Data on plant growth and infection parameters were analyzed using one-way analysis of variance (ANOVA) under a completely randomized design (CRD). Comparisons of means were conducted using Tukey’s Honestly Significant Difference (HSD) test. Statistical analyses were performed using GenStat software (12th Edition, Version 12.1.0.3278, www.vsni.co.uk), at a significance level of p < 0.05.
3 Results
3.1 Molecular characterization of rhizobacteria
The molecular characterization of rhizobacterial isolates was conducted using the 16S rRNA gene region, amplified with universal primers 27F and 1492R. The amplification successfully yielded amplicons of approximately 1500 base pairs, as visualized through gel electrophoresis (Figure 1). This fragment size corresponds to the expected region of the 16S rRNA gene, which is widely used for bacterial identification and phylogenetic studies.
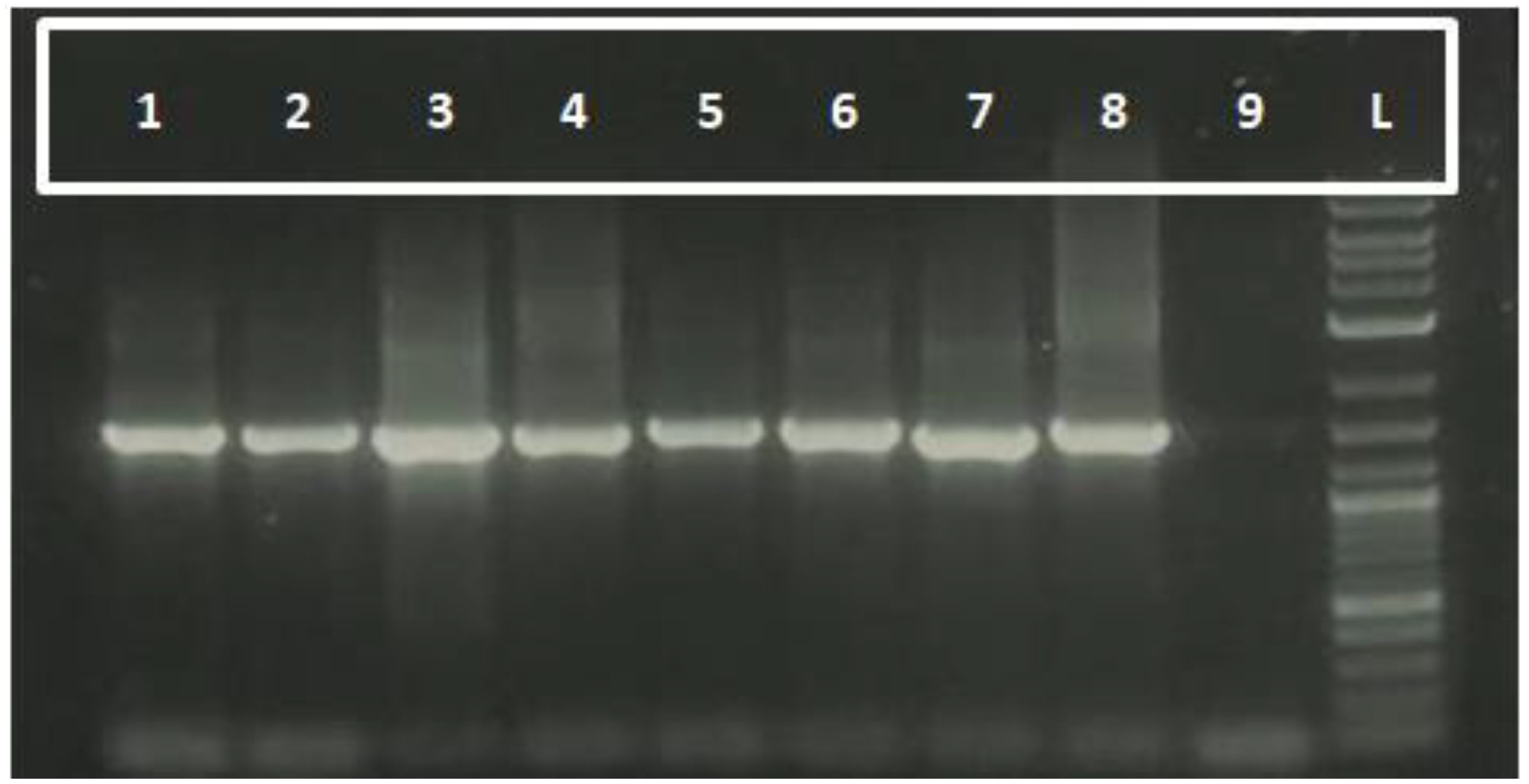
Figure 1. Molecular validation of rhizobacterial isolates using 16S rRNA Primers. Lane 1–8 represents the rhizobacterial isolates, 9 as control and L represents 1kb Ladder.
The sequencing data obtained from the amplified products were compared against the National Center for Biotechnology Information (NCBI) database using the BLAST algorithm to confirm the identity of the isolates at the species level. The rhizobacterial isolates, along with their respective GenBank accession numbers, are listed in Table 2. The high sequence similarity (>99%) with reference sequences in the database validated the taxonomic classification of the isolates.
3.2 Effect of rhizobacteria and plant extracts on tomato growth
The effect of different treatments of M. oleifera, both individually and in combination, was evaluated against M. incognita in terms of plant growth parameters (plant height, root length, fresh shoot weight, dry shoot weight, fresh root weight, dry root weight) and M. incognita infection parameters (root gall ratings, eggs per root system, J2/60 cc soil, and reproduction factor).
Plant height and root length were higher in nearly all treatments compared to the nematode control, but only treatment 3 differed significantly (Figure 2). The highest plant height (96.94 cm) and root length (30.48 cm) were recorded in T3 (BA-LWD73 + M. oleifera), whereas the lowest values (63.50 cm and 22.44 cm, respectively) were observed in T7 (BT-WAG41 + M. oleifera).
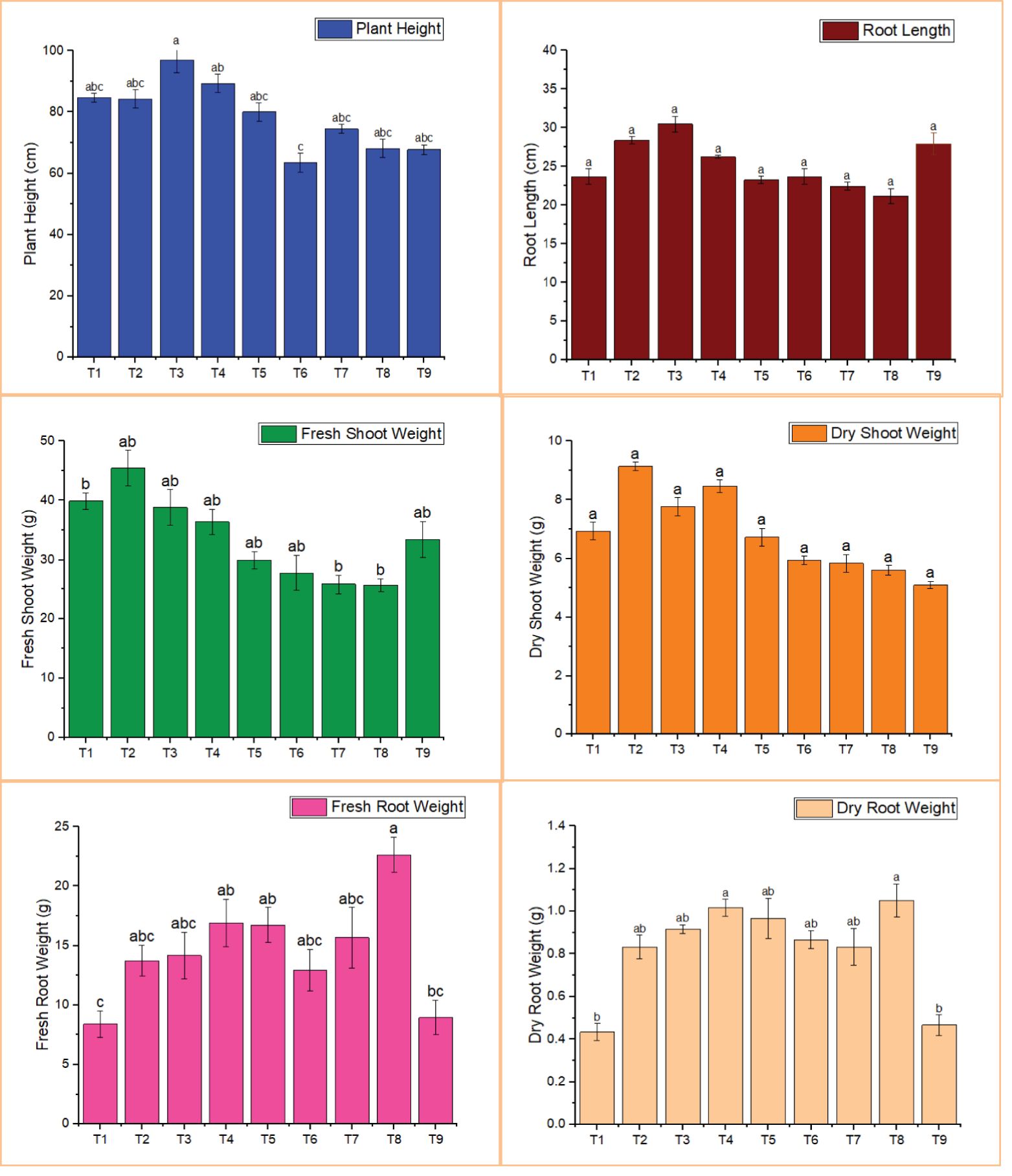
Figure 2. Effect of M. oleifera and rhizobacteria on the growth parameters of tomato. T1, M. oleifera + M. incognita; T2, B. australimaris LWD73 + M. incognita; T3, B. australimaris LWD73 + M. oleifera + M. incognita; T4, B. cereus HR001 + M. incognita; T5, B. cereus HR001 + M. oleifera + M. incognita; T6, B. thuringiensis WAG41 + M. incognita; T7, B. thuringiensis WAG41 + M. oleifera + M. incognita; T8, M. incognita only and T9, Healthy Control.
For fresh shoot weight and dry shoot weight, the highest values were recorded in T2, which involved the single treatment of BA-LWD73, with fresh and dry shoot weights of 45.43 g and 9.13 g, respectively. The lowest fresh shoot weight (25.80 g) was observed in T7 (BT-WAG41 + M. oleifera), while the lowest dry shoot weight (5.93 g) was recorded in both T6 and T7 (BT-WAG41 alone and in combination with M. oleifera) (Figure 2).
Fresh and dry root weights were lower in all treatments, significantly so in T1 and T9, compared to the nematode control. The lowest fresh root weight (8.40 g) was recorded in T1, which received the single treatment of M. oleifera. The lowest dry root weight (5.83 g) was observed in T7 (BT-WAG41 + M. oleifera) (Figure 2).
3.3 Effect of rhizobacteria and plant extracts on M. incognita infection
Root gall ratings were lower in most treatments, significantly so in treatments T1-T3, compared to the positive control (Figure 3). The lowest root gall rating (2.67) was observed in T3, which consisted of the combined treatment of BA-LWD73 and M. oleifera, whereas T8 (M. incognita only, control) exhibited the highest root gall rating.
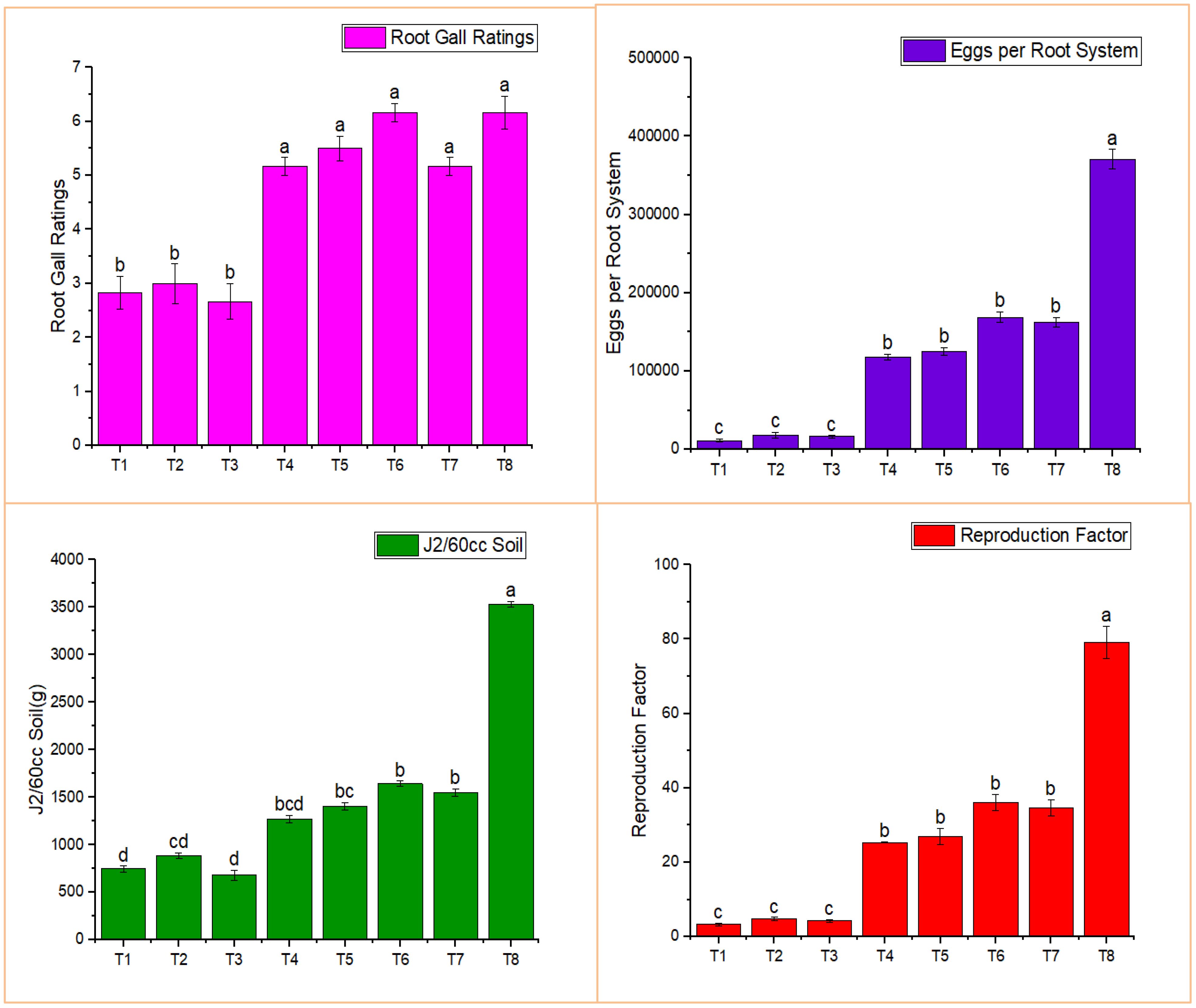
Figure 3. Effect of M. oleifera and rhizobacteria on infection parameters of M. incognita. T1, M. oleifera + M. incognita; T2, B. australimaris LWD73 + M. incognita; T3, B. australimaris LWD73 + M. oleifera + M. incognita; T4, B. cereus HR001 + M. incognita; T5, B. cereus HR001 + M. oleifera + M. incognita; T6, B. thuringiensis WAG41 + M. incognita; T7, B. thuringiensis WAG41 + M. oleifera + M. incognita; T8, M. incognita only and T9, Healthy Control.
The single and combined applications of M. oleifera and BA-LWD73 resulted in 11,500, 18,000, and 16,500 eggs per root system, respectively. The lowest number of J2 was recovered from the single and combined applications of M. oleifera and BA-LWD73, with values of 746.83, 884.33, and 680 J2 per 60 cm³ of soil, respectively. Similarly, the lowest reproduction factors were recorded in the single and combined applications of M. oleifera and BA-LWD73, with values of 3.35, 4.84, and 4.25, respectively (Figure 3).
3.4 FTIR and GC-MS analysis of aqueous extracts of M. oleifera
The FTIR spectra of M. oleifera are shown in Figure 4. The first and second peaks, observed at wavelengths 2916.36 cm-¹ and 2848.86 cm-¹, correspond to strong and broad stretching vibrations of hydroxyl groups, predominantly associated with alcohols. The third peak, at 1620.20 cm-¹, exhibited medium stretching vibrations, primarily indicating the presence of a C=C functional group, characteristic of alkenes. The fourth peak, at 1415.53 cm-¹, showed strong stretching vibrations attributed to S=O bonds, suggesting the presence of sulfates. The fifth peak, ranging from 1315.45 cm-¹ to 1022.27 cm-¹, exhibited strong stretching vibrations corresponding to C-O functional groups, predominantly signifying tertiary alcohols. The final peak, observed at 513.06 cm-¹, was characteristic of halo compounds.
The GC-MS chromatogram of the aqueous extract of M. oleifera revealed six distinct peaks, corresponding to six phytocompounds identified through comparison with the NIST library: 4(1H)-Pyrimidinone (3.02%), 2,6-Dimethyl acetic acid [(aminocarbonyl)amino]oxo (3.01%), Maltol (9.58%), 4H-Pyran-4-one, 2,3-dihydro-3,5-dihydroxy-6-methyl (10.30%), 5-Hydroxymethylfurfural (71.76%), and 4(1H)-Pyrimidinone, 2-(methylthio) (2.32%) (Table 3). These phytocompounds are reported to exhibit antimicrobial and nematicidal properties.
3.5 Detection of VOCs using SPME-GC-MS
Volatile organic compounds (VOCs) produced by B. australimaris strain LWD-73 were identified based on GC-MS analysis and comparison with the NIST library (Figure 5, Table 4). Four peaks were detected, corresponding to 2-Nonanone (6.54%), 1H-Indole (87.46%), Tetradecanol (0.99%), and 9-Hexadecenoic acid (5.02%).
4 Discussion
M. incognita is one of the most economically important plant-parasitic nematodes worldwide, causing significant yield losses in a wide range of crops (Mukhtar and Hussain, 2019). It is highly prevalent in tropical and subtropical regions making it a major threat to agricultural productivity (Mukhtar and Kayani, 2019, 2020). Its widespread prevalence necessitates effective management strategies to mitigate its impact on agricultural productivity and food security.
In this study, the aqueous extract of M. oleifera, both alone and in combination with rhizobacterial strains, effectively reduced M. incognita populations and enhanced plant growth. Treatments combining M. oleifera with B. australimaris strain LWD-73 demonstrated superior performance compared to other treatments, significantly reducing nematode infection and promoting plant vigor.
Numerous plant species possess nematicidal constituents that suppress nematode populations and improve plant health (Mukhtar et al., 2013b). All parts of M. oleifera can be utilized as biopesticides due to their ability to suppress pathogens and enhance crop health (Yaseen and Hájos, 2020).
M. oleifera contains diverse bioactive compounds, including aldehydes, flavonoids, alcohols, phenols, and terpenoids, which may act individually or synergistically to affect nematodes. These compounds disrupt nematode feeding and reproduction, inhibit egg hatching, and exhibit juvenile toxicity. However, the precise mechanisms underlying their nematicidal activity remain unclear (Mukhtar et al., 2013b; Yaseen and Hájos, 2020).
Microbes also play a pivotal role in managing polyphagous root-knot nematodes across various crops and soil conditions (Mukhtar, 2018). They enhance plant health, induce systemic resistance, and help mitigate a wide range of biotic stresses. Rhizobacteria are particularly valuable for their contributions to nutrient uptake, phytohormone production, heavy metal stress mitigation, and increased crop yields (Groover et al., 2020; Bhat et al., 2023). Certain bacterial volatile organic compounds (VOCs), such as dimethyl disulfide, 2-nonanone, 1H-indole, tetradecanol, and 9-hexadecenoic acid, exhibit strong nematicidal activity against root-knot nematodes (Bhattacharyya et al., 2015; Agisha et al., 2019; Bhat et al., 2023).
FTIR and GC-MS analyses of M. oleifera extracts confirmed the presence of flavonoids, alcohols, and phenols, which are known for their antimicrobial and nematicidal properties. The nematicidal activity of M. oleifera was positively correlated with the abundance of these phytochemicals (Alam and Nuby, 2022; Anekwe et al., 2023).
Among the rhizobacteria tested, B. australimaris strain LWD-73 exhibited the most potent effects against M. incognita. SPME-GC-MS analysis identified key volatile organic compounds produced by B. australimaris, including 2-nonanone, 9-hexadecenoic acid, tetradecanol, and 1H-indole, all of which possess strong antimicrobial, antifungal, and nematicidal properties. For instance, 2-nonanone demonstrates significant nematicidal activity by inhibiting egg hatching, disrupting feeding, and reducing nematode populations, thereby limiting root damage over time (Huang et al., 2010; Cheng et al., 2017). Moreover, this compound exhibits antifungal activity against pathogens such as Verticillium longisporum and Botrytis cinerea (Rybakova et al., 2017).
Indole, another prominent VOC, interferes with nematode egg-laying and survival, induces oxidative stress at high concentrations, and triggers methuosis at low concentrations, ultimately leading to nematode mortality (Bommarius et al., 2013; Lee et al., 2017). Moreover, indole functions as a key chemical signal that promotes plant growth and influences auxin signaling. For example, indole emitted by Escherichia coli has been shown to enhance the root architecture of Arabidopsis thaliana (Bhattacharyya et al., 2015; Elzey et al., 2016; Ahmed et al., 2024).
9-Hexadecenoic acid (palmitoleic acid) exhibits nematicidal activity by inducing toxicity in larvae and eggs, acting as a repellent, and interfering with nematode feeding and reproduction. It may also stimulate plant defenses and influence soil microbial communities, presenting a promising approach to integrated nematode management (Cordovez et al., 2017).
Tetradecanol, emitted by Paenibacillus polymyxa strain J2-4, has demonstrated strong fumigant activity against M. incognita, further emphasizing the potential of volatile organic compounds in nematode management strategies (Song et al., 2024). These findings highlight the significance of plant-derived phytochemicals and microbial VOCs as sustainable tools for controlling nematodes and improving crop health.
5 Conclusions
The present study identified a novel bacterial strain, Bacillus australimaris strain LWD-73, with significant nematicidal activity, offering potential for the effective management of Meloidogyne incognita. The integration of this bacterial strain with moringa leaf extract demonstrated compatibility. Both moringa and the selected rhizobacteria are rich in phytochemicals with potent nematicidal and antimicrobial properties. These bioactive compounds provide promising solutions for nematode management and can be incorporated into integrated pest management (IPM) strategies. Small-scale vegetable growers can benefit from using aqueous moringa leaf extracts as a cost-effective and accessible method for nematode control, ultimately improving the health and productivity of vegetable crops.
Data availability statement
The raw data supporting the conclusions of this article will be made available by the authors, without undue reservation.
Author contributions
WA: Data curation, Formal analysis, Investigation, Software, Writing – original draft, Writing – review & editing. TM: Conceptualization, Data curation, Formal analysis, Methodology, Supervision, Writing – review & editing. MIH: Data curation, Supervision, Writing – review & editing. MAK: Data curation, Supervision, Writing – review & editing. MSI: Data curation, Formal analysis, Writing – review & editing. AH: Data curation, Writing – review & editing. HR: Data curation, Formal analysis, Validation, Writing – review & editing. LWD: Funding acquisition, Resources, Supervision, Validation, Writing – review & editing.
Funding
The author(s) declare that financial support was received for the research and/or publication of this article. This research was funded by the Higher Education Commission (HEC) of Pakistan under the “Indigenous Ph.D. Fellowship Program” and the “International Research Support Initiative Program” (IRSIP).
Conflict of interest
The authors declare that the research was conducted in the absence of any commercial or financial relationships that could be construed as a potential conflict of interest.
Generative AI statement
The author(s) declare that Generative AI was used in the creation of this manuscript. Chat GPT was used to rearrange abstract.
Publisher’s note
All claims expressed in this article are solely those of the authors and do not necessarily represent those of their affiliated organizations, or those of the publisher, the editors and the reviewers. Any product that may be evaluated in this article, or claim that may be made by its manufacturer, is not guaranteed or endorsed by the publisher.
References
Abbas, M., Saadedin, S., Suleiman, A. (2024). Cloning and overexpression of Zea mays cystatin 2 (ccii) gene in Bacillus subtilis to reduce root-knot nematode infection in cucumber plants in Iraq. Plant Prot. 8, 621–633. doi: 10.33804/pp.008.04.5335
Afzal, A., Mukhtar, T. (2024). Revolutionizing nematode management to achieve global food security goals—An overview. Heliyon 10, e25325. doi: 10.1016/j.heliyon.2024.e25325
Agisha, V. N., Kumar, A., Eapen, S. J., Sheoran, N., Suseelabhai, R. (2019). Broad-spectrum antimicrobial activity of volatile organic compounds from endophytic Pseudomonas putida BP25 against diverse plant pathogens. Biocontrol Sci. Technol. 29, 1069–1089. doi: 10.1080/09583157.2019.1657067
Ahmed, M. H., Ashfaq, M., Mukhtar, T., Khan, M. A. (2021). Categorization of available cucumber genotypes against Zucchini yellow mosaic virus and root-knot nematode (Meloidogyne incognita). Int. J. Agric. Biol. 25, 955–961. doi: 10.17957/IJAB/15.1751
Ahmed, D., Shahab, S., Ahmad, M., Pathak, N. (2024). “Mahua oilseed cake: Chemical compounds and nematicidal potential,” in Oilseed Cake for Nematode Management (Boca Raton, Florida, USA: CRC Press), 81–88.
Alam, E. A., Nuby, A. S. E. (2022). Phytochemical and nematicidal screening on some extracts of different plant parts of Egyptian Moringa oleifera L. Pak. J. Phytopath. 34, 293–306. doi: 10.33866/phytopathol.034.02.0818
Aminisarteshnizi, M. (2024). Efficacy of moringa (Moringa oleifera) formulations on suppression of root-knot nematodes (Meloidogyne incognita) and growth of tomato (Solanum lycopersicum). Res. Crops 25, 185–189. doi: 10.31830/2348-7542.2024.ROC-1019
Anekwe, I. I., Ifenyinwa, C. C., Amadi, E. S., Ursulla, N. N., Chukwuebuka, I. F. (2023). Characterization of aqueous extract of Moringa oleifera leaves using GC-MS analysis. J. Adv. Microbiol. 23, 8–18. doi: 10.9734/jamb/2023/v23i6727
Asghar, A., Mukhtar, T., Raja, M. U., Gulzar, A. (2020). Interaction between Meloidogyne javanica and Ralstonia solanacearum in chili. Pak. J. Zool. 52, 1525–1530. doi: 10.17582/journal.pjz/20190501030529
Aslam, N., Atiq, M., Rajput, N. A., Akram, A., Arif, A. M., Kachelo, G. A., et al. (2024). Explicating botanical bactericides as an intervention tool towards citrus canker. Plant Prot. 8, 25–32. doi: 10.33804/pp.008.01.4788
Aslam, M. N., Mukhtar, T. (2023a). Characterization of Ralstonia solanacearum causing bacterial wilt from major chili growing areas of Pakistan. Bragantia 82, e20230001. doi: 10.1590/1678-4499.20230001
Aslam, M. N., Mukhtar, T. (2023b). Distributional spectrum of bacterial wilt of chili incited by Ralstonia solanacearum in Pakistan. Bragantia 82, e20220196. doi: 10.1590/1678-4499-2022-0196
Aslam, M. N., Mukhtar, T. (2024). Evaluation of virulence among Pakistani isolates of Ralstonia solanacearum inducing bacterial wilt in chilies across different agro-ecological zones. Bragantia 83, e20230181. doi: 10.1590/1678-4499.20230181
Aslam, M. N., Khaliq, H., Zhao, H., Moosa, A., Maqsood, A., Farooqi, M. A., et al (2025). Thymol as a novel plant‑derived antibacterial agent for suppressing Xanthomonas citri pv. malvacearum in cotton. Curr. Microbiol. 82, 99. doi: 10.1007/s00284-025-04077-5
Aslam, M. N., Mukhtar, T., Hussain, M. A., Raheel, M. (2017). Assessment of resistance to bacterial wilt incited by Ralstonia solanacearum in tomato germplasm. J. Plant Dis. Prot. 124, 585–590. doi: 10.1007/s41348-017-0100-1
Atiq, M., Malik, L., Rajput, N. A., Shabbir, M., Ashiq, M., Matloob, M. J., et al. (2024). Evaluating the efficacy of plant-mediated copper-silver nanoparticles for controlling Cercospora leaf spot in mung beans. Plant Prot. 8, 661–670. doi: 10.33804/pp.008.04.5381
Ayub, H. M., Akram, M., Mehak, A., Ajmal, M., Ilyas, I., Seerat, W., et al. (2024). A preliminary study on the interaction between Meloidogyne incognita and some strains of Pseudomonas spp. on growth performance of tomato under greenhouse conditions. Plant Prot. 8, 341–350. doi: 10.33804/008.02.5196
Azeem, W., Mukhtar, T., Hamid, T. (2021). Evaluation of Trichoderma harzianum and Azadirachta indica in the management of Meloidogyne incognita in tomato. Pak. J. Zool. 53, 119–125. doi: 10.17582/journal.pjz/20190905100940
Aziz, S., Jamshed, S. A., Mukhtar, T., Irshad, G., Ijaz, S. S., Raja, M. U. (2024). Evaluation of Bacillus spp. as biocontrol agents against chili leaf spot caused by Xanthomonas vesicatoria. J. Plant Dis. Protect. 131, 987–997. doi: 10.1007/s41348-024-00866-5
Bhat, A. A., Shakeel, A., Waqar, S., Handoo, Z. A., Khan, A. A. (2023). Microbes vs. nematodes: Insights into biocontrol through antagonistic organisms to control root-knot nematodes. Plants 12, 451.
Bhattacharyya, D., Garladinne, M., Lee, Y. H. (2015). Volatile indole produced by rhizobacterium Proteus vulgaris JBLS202 stimulates growth of Arabidopsis thaliana through auxin, cytokinin, and brassinosteroid pathways. J. Plant Growth Regul. 34, 158–168. doi: 10.1007/s00344-014-9453-x
Bommarius, B., Anyanful, A., Izrayelit, Y., Bhatt, S., Cartwright, E., Wang, W., et al. (2013). A family of indoles regulate virulence and Shiga toxin production in pathogenic E. coli. PloS One 8, e54456. doi: 10.1371/journal.pone.0054456
Cheng, W., Yang, J., Nie, Q., Huang, D., Yu, C., Zheng, L., et al. (2017). Volatile organic compounds from Paenibacillus polymyxa KM2501–1 control Meloidogyne incognita by multiple strategies. Sci. Rep. 7, 16213. doi: 10.1038/s41598-017-16631-8
Cordovez, V., Mommer, L., Moisan, K., Lucas-Barbosa, D., Pierik, R., Mumm, R., et al. (2017). Plant phenotypic and transcriptional changes induced by volatiles from the fungal root pathogen Rhizoctonia solani. Front. Plant Sci. 8, 1262. doi: 10.3389/fpls.2017.01262
Elzey, B., Pollard, D., Fakayode, S. O. (2016). Determination of adulterated neem and flaxseed oil compositions by FTIR spectroscopy and multivariate regression analysis. Food Control 68, 303–309. doi: 10.1016/j.foodcont.2016.04.008
FAO (2021). World agriculture: Towards 2015/2030 – An FAO perspective (Viale delle Terme di Caracalla, 00100 Rome, Italy: Information Division FAO).
Fullana, A. M., Exposito, A., Escudero, N., Cunquero, M., Loza-Alvarez, P., Gine, A., et al. (2023). Crop rotation with Meloidogyne-resistant germplasm is useful to manage and revert the (a)virulent populations of Mi1.2 gene and reduce yield losses. Front. Plant Sci. 14. doi: 10.3389/fpls.2023.1133095
Geldenhuys, G. (2023). Efficacy of moringa (Moringa oleifera) formulations on suppression of root-knot nematodes (Meloidogyne javanica) and growth of eggplant (Solanum melongena). Res. Crops 24, 428–431. doi: 10.31830/2348-7542.2023.ROC-961
Groover, W., Held, D., Lawrence, K., Carson, K. (2020). Plant growth-promoting rhizobacteria: A novel management strategy for Meloidogyne incognita on turfgrass. Pest Manage. Sci. 76, 3127–3138. doi: 10.1002/ps.5862
Haq, M. A., Mukhtar, T., Haq, M. I., Khalid, A. (2022). Reproduction of root-knot nematode, Meloidogyne incognita, on Solanum melongena genotypes determines their host status. Pak. J. Zool. 54, 2097–2103. doi: 10.17582/journal.pjz/20210510080523
Hassan, A., Abbas, H., Abbasi, W. M., ur Rahman, M. S., Akram, W., Rafiq, F., et al. (2024). Evaluation of rhizospheric Pseudomonas spp. for the management of Fusarium wilt of tomato in Cholistan, Pakistan. Plant Prot. 8, 433–445. doi: 10.33804/pp.008.03.5238
Huang, Y., Xu, C., Ma, L., Zhang, K., Duan, C., Mo, M. (2010). Characterisation of volatiles produced from Bacillus megaterium YFM3.25 and their nematicidal activity against Meloidogyne incognita. Eur. J. Plant Pathol. 126, 417–422. doi: 10.1007/s10658-009-9551-5
Hussain, M. A., Mukhtar, T. (2019). Root-knot nematodes infecting okra in major vegetable-growing districts of Punjab, Pakistan. Pak. J. Zool. 51, 1137–1143. doi: 10.17582/journal.pjz/2019.51.3.1137.1143
Hussain, M. A., Mukhtar, T., Kayani, M. Z. (2011). Efficacy evaluation of Azadirachta indica, Calotropis procera, Datura stramonium and Tagetes erecta against root-knot nematodes Meloidogyne incognita. Pak. J. Bot. 43, 197–204.
Iqbal, R. J., Jatoi, G. H., Ahmad, A., Lutf, A., Hussain, Q., Sukhera, M. N., et al. (2024). Integrated management of tomato gray mold disease using selective plant extracts and rhizobacteria. Plant Prot. 8, 781–787. doi: 10.33804/pp.008.04.5528
Karssen, G., Moens, M. (2006). “Root-knot nematodes,” in Plant Nematology. Eds. Perry, R. N., Moens, M. (CAB International, Wallingford), 59–90.
Kayani, M. Z., Mukhtar, T. (2018). Reproductivity of Meloidogyne incognita on fifteen cucumber cultivars. Pak. J. Zool. 50, 1717–1722. doi: 10.17582/journal.pjz/2018.50.5.1717.1722
Kayani, M. Z., Mukhtar, T., Hussain, M. A. (2012). Evaluation of nematicidal effects of Cannabis sativa L. and Zanthoxylum alatum Roxb. against root-knot nematodes, Meloidogyne incognita. Crop Prot. 39, 52–56. doi: 10.1016/j.cropro.2012.03.005
Kayani, M. Z., Mukhtar, T., Hussain, M. A. (2018). Interaction between nematode inoculum density and plant age on growth and yield of cucumber and reproduction of Meloidogyne incognita. Pak. J. Zool. 50, 897–902. doi: 10.17582/journal.pjz/2018.50.3.897.902
Kayani, M. Z., Mukhtar, T., Hussain, M. A., Haque, M. I. (2013). Infestation assessment of root-knot nematodes (Meloidogyne spp.) associated with cucumber in the Pothowar region of Pakistan. Crop Prot. 47, 49–54. doi: 10.1016/j.cropro.2013.01.009
Khairy, D., Refaei, A., Mostafa, F. (2021). Management of Meloidogyne incognita infecting eggplant using moringa extracts, vermicompost, and two commercial bio-products. Egypt. J. Agronematol. 20, 1–16. doi: 10.21608/ejaj.2021.134910
Khan, M. T. A., Mukhtar, T., Saeed, M. (2019). Resistance or susceptibility of eight aubergine cultivars to Meloidogyne javanica. Pak. J. Zool. 51, 2187–2192. doi: 10.17582/journal.pjz/2019.51.6.2187.2192
Khandagale, P., Kansara, S., Padsala, J., Patel, P. (2024). Plant growth-promoting rhizobacteria for sustainable production of sugarcane and rice. Int. J. Plant Sci. 36, 298–305. doi: 10.9734/ijpss/2024/v36i34427
Kumar, V., Khan, M. R., Walia, R. K. (2020). Crop loss estimations due to plant-parasitic nematodes in major crops in India. Natl. Acad. Sci. Lett. 43, 275–280. doi: 10.1007/s40009-019-00860-3
Kumar, S., Ramanjini Gowda, P., Saikia, B., Debbarma, J., Velmurugan, N., Chikkaputtaiah, C. (2018). Screening of tomato genotypes against bacterial wilt (Ralstonia solanacearum) and validation of resistance-linked DNA markers. Australas. Plant Pathol. 47, 365–374. doi: 10.1007/s13313-018-0576-2
Lee, J. H., Kim, Y. G., Kim, M., Kim, E., Choi, H., Kim, Y., et al. (2017). Indole-associated predator–prey interactions between the nematode Caenorhabditis elegans and bacteria. Environ. Microbiol. 19, 1776–1790. doi: 10.1111/emi.2017.19.issue-5
Manzoor, F., Atiq, M., Aleem, M., Naveed, K., Khan, N. A., Kachelo, G. A., et al. (2024). Appraisal of antifungal potential of chemicals and plant extracts against brown leaf spot of soybean caused by Septoria glycine. Plant Prot. 8, 447–455. doi: 10.33804/pp.008.03.5243
Mukhtar, T. (2018). Management of root-knot nematode, Meloidogyne incognita, in tomato with two Trichoderma species. Pak. J. Zool. 50, 1589–1592. doi: 10.17582/journal.pjz/2018.50.4.sc15
Mukhtar, T., Arshad, I., Kayani, M. Z., Hussain, M. A., Kayani, S. B., Rahoo, A. M., et al. (2013a). Estimation of damage to okra (Abelmoschus esculentus) by root-knot disease incited by Meloidogyne incognita. Pak. J. Bot. 45, 1023–1027.
Mukhtar, T., Hussain, M. A. (2019). Pathogenic potential of Javanese root-knot nematode on susceptible and resistant okra cultivars. Pak. J. Zool. 51, 1891–1897. doi: 10.17582/journal.pjz/2019.51.5.1891.1897
Mukhtar, T., Hussain, M. A., Kayani, M. Z. (2013c). Biocontrol potential of Pasteuria penetrans, Pochonia chlamydosporia, Paecilomyces lilacinus and Trichoderma harzianum against Meloidogyne incognita in okra. Phytopathol. Mediterr. 52, 66–76.
Mukhtar, T., Kayani, M. Z. (2019). Growth and yield responses of fifteen cucumber cultivars to root-knot nematode (Meloidogyne incognita). Acta Sci. Pol. Hortorum Cultus 18, 45–52. doi: 10.24326/asphc.2019.3.5
Mukhtar, T., Kayani, M. Z. (2020). Comparison of the damaging effects of Meloidogyne incognita on a resistant and susceptible cultivar of cucumber. Bragantia 79, 83–93. doi: 10.1590/1678-4499.20190359
Mukhtar, T., Kayani, M. Z., Hussain, M. A. (2013b). Nematicidal activities of Cannabis sativa L. and Zanthoxylum alatum Roxb. against Meloidogyne incognita. Ind. Crops Prod. 42, 447–453. doi: 10.1016/j.indcrop.2012.06.027
Mukhtar, T., Kayani, M. Z., Hussain, M. A. (2013d). Response of selected cucumber cultivars to Meloidogyne incognita. Crop Prot. 44, 13–17. doi: 10.1016/j.cropro.2012.10.015
Mukhtar, T., Khan, M. T., Aslam, M. N. (2021). Bioefficacy of Trichoderma species against Javanese root-knot nematode, Meloidogyne javanica, in green gram. Gesunde Pflanzen 73, 265–272. doi: 10.1007/s10343-021-00544-8
Mustafa, A., Naseer, S., Saeed, S., Ahmad, S., Shahzad, U., Khan, A. A., et al. (2024). Exploring the potential of systemic fungicides and plant extracts in suppressing Fusarium wilt of tomato. Plant Prot. 8, 229–238. doi: 10.33804/pp.008.02.5113
Nazir, K., Mukhtar, T., Javed, H. (2019). In vitro effectiveness of silver nanoparticles against root-knot nematode (Meloidogyne incognita). Pak. J. Zool. 51, 2077–2083. doi: 10.17582/journal.pjz/2019.51.6.2077.2083
Nikoo, F. S., Sahebani, N., Aminian, H., Mokhtarnejad, L., Ghaderi, R. (2014). Induction of systemic resistance and defense-related enzymes in tomato plants using Pseudomonas fluorescens CHAO and salicylic acid against root-knot nematode Meloidogyne javanica. J. Plant Prot. Res. 54 (4), 383–389.
Oka, Y., Koltai, H., Bar-Eyal, M., Mor, M., Sharon, E., Chet, I., et al. (2000). New strategies for the control of plant parasitic nematodes. Pest Manage. Sci. 56, 983–988. doi: 10.1002/1526-4998(200011)56:11<983::AID-PS233>3.0.CO;2-X
Ornat, C., Verdejo-Lucas, S., Sorribas, F. J. (2001). A population of Meloidogyne javanica in Spain virulent to the Mi resistance gene in tomato. Plant Dis. 85, 271–276. doi: 10.1094/PDIS.2001.85.3.271
Rybakova, D., Rack-Wetzlinger, U., Cernava, T., Schaefer, A., Schmuck, M., Berg, G. (2017). Aerial warfare: a volatile dialogue between the plant pathogen Verticillium longisporum and its antagonist Paenibacillus polymyxa. Front. Plant Sci. 8, 278579. doi: 10.3389/fpls.2017.01294
Saeed, M., Mukhtar, T. (2024). Root-knot nematodes (Meloidogyne spp.) infecting peach (Prunus persica L.) in the Pothwar region of Pakistan. J. Agric. Sci. Technol. 26, 897–908.
Saeed, M., Mukhtar, T., Ahmed, R., Ahmad, T., Iqbal, M. A. (2023). Suppression of Meloidogyne javanica infection in peach (Prunus persica (L.) Batsch) using fungal biocontrol agents. Sustainability 15, 13833. doi: 10.3390/su151813833
Saeed, M., Mukhtar, T., Haq, M. I., Khan, M. A. (2021). Assessment of nematicidal potential of Cannabis sativa and Azadirachta indica in the management of root-knot nematode (Meloidogyne javanica) on peach. Pak. J. Agric. Sci. 58, 1555–1561. doi: 10.21162/PAKJAS/21.1282
Shahid, M., Gowen, S. R., Burhan, M., Niaz, M. Z., Anwar-ul-Haq, M., Mehmood, K. (2024). Differential responses of Meloidogyne spp. to Pasteuria isolates over crop cycles. Plant Prot. 8, 257–267. doi: 10.33804/pp.008.02.5192
Shahzaman, S., Inam-ul-Haq, M., Mukhtar, T., Naeem, M. (2015). Isolation, identification of antagonistic rhizobacterial strains obtained from chickpea (Cicer arietinum L.) field and their in-vitro evaluation against fungal root pathogens. Pak. J. Bot. 47, 1553–1558.
Song, W., Dai, M., Gao, S., Mi, Y., Zhang, S., Wei, J., et al. (2024). Volatile organic compounds produced by Paenibacillus polymyxa J2-4 exhibit toxic activity against Meloidogyne incognita. Pest Manage. Sci. 80, 1289–1299. doi: 10.1002/ps.7932
Taha, Z. R., Altaai, A. F., Mohammad, T. H., Khajeek, T. R. (2024). Efficacy of silver nanoparticles from Fusarium solani and mycorrhizal inoculation for biological control of Fusarium wilt in tomato. Plant Prot. 8, 635–648. doi: 10.33804/pp.008.04.5343
Tait, E., Perry, J. D., Stanforth, S. P., Dean, J. R. (2014). Identification of volatile organic compounds produced by bacteria using HS-SPME-GC–MS. J. Chromatogr. Sci. 52, 363–373. doi: 10.1093/chromsci/bmt042
Tariq-Khan, M., Mukhtar, T., Munir, A., Hallmann, H., Heuer, H. (2017). Distribution of root-knot nematode species and their virulence on vegetables in northern temperate agro-ecosystems of the Pakistani-administered territories of Azad Jammu and Kashmir. J. Plant Dis. Prot. 124, 201–212. doi: 10.1007/s41348-016-0045-9
Tariq-Khan, M., Mukhtar, T., Munir, A., Hallmann, H., Heuer, H. (2020). Comprehensive report on the prevalence of root-knot nematodes in the Poonch division of Azad Jammu and Kashmir, Pakistan. J. Phytopathol. 168, 322–336. doi: 10.1111/jph.12913
Tariq-Khan, M., Rehman, T. U., Mukhtar, T., Mahmood, M., Rahman, A. U., Ahmed, R. (2025). Population dynamics and virulence patterns of root-knot nematodes (Meloidogyne spp.) on tomato in Poonch Highlands, Azad Jammu and Kashmir, Pakistan. J. Phytopathol. 173, e70060. doi: 10.1111/jph.70060
Taylor, D. P., Netscher, C. (1974). An improved technique for preparing perineal patterns of Meloidogyne spp. Nematologica 20, 268–269. doi: 10.1163/187529274X00285
Vagelas, I. (2024). Math in plant pathology: is the early blight of tomato one more example of the golden ratio? Plant Prot. 8, 691–700. doi: 10.33804/pp.008.04.5435
Yaseen, A., Hájos, M. T. (2020). Study on moringa tree (Moringa oleifera Lam.) leaf extract in organic vegetable production: A review. Res. Crops 21, 402–414.
Yaseen, I., Mukhtar, T. (2024). Impact of sequential and concurrent inoculations of Meloidogyne incognita and Fusarium oxysporum f.sp. vasinfectum on the growth performance of diverse okra cultivars. Plant Prot. 8, 303–313. doi: 10.33804/pp.008.02.5186
Yaseen, I., Mukhtar, T., Kim, H. T., Arshad, B. (2023). Quantification of resistance to Meloidogyne incognita in okra cultivars using linear and nonlinear analyses of growth parameters and nematode infestations. Bragantia 82, e20230114. doi: 10.1590/1678-4499.20230114
Yaseen, I., Mukhtar, T., Kim, H.-T., Arshad, B. (2024). Interactive effects of Meloidogyne incognita and Fusarium oxysporum f.sp. vasinfectum on okra cultivars. Bragantia 83, e20230266. doi: 10.1590/1678-4499.20230266
Yaseen, I., Mukhtar, T., Mubarik, A., Arshad, B., Sahu, N., Somaddar, U. (2025). Management of Ralstonia solanacearum-Meloidogyne incognita complex with Trichoderma harzianum in tomato. Bragantia 84, e20240060. doi: 10.1590/1678-4499.20240060
Keywords: root-knot nematodes, biological control, Meloidogyne incognita, Moringa oleifera, rhizobacteria, bacterial VOCs, FTIR, GC-MS
Citation: Azeem W, Mukhtar T, Inam-ul-Haq M, Khan MA, Ibrahim MS, Hassan A, Regmi H and Duncan LW (2025) The nematicidal potential of Moringa oleifera extracts and rhizobacteria against Meloidogyne incognita in tomato. Front. Plant Sci. 16:1562074. doi: 10.3389/fpls.2025.1562074
Received: 16 January 2025; Accepted: 07 April 2025;
Published: 13 May 2025.
Edited by:
Eustachio Tarasco, Faculty of Agricultural Science, University of Bari Aldo Moro, ItalyReviewed by:
Alberto Troccoli, National Research Council (CNR), ItalyAlessio Vovlas, National Research Council (CNR), Italy
Copyright © 2025 Azeem, Mukhtar, Inam-ul-Haq, Khan, Ibrahim, Hassan, Regmi and Duncan. This is an open-access article distributed under the terms of the Creative Commons Attribution License (CC BY). The use, distribution or reproduction in other forums is permitted, provided the original author(s) and the copyright owner(s) are credited and that the original publication in this journal is cited, in accordance with accepted academic practice. No use, distribution or reproduction is permitted which does not comply with these terms.
*Correspondence: Wajahat Azeem, d2FqYWhhdDM2QGdtYWlsLmNvbQ==; Tariq Mukhtar, ZHJ0bXVraHRhckB1YWFyLmVkdS5waw==