- 1College of Agronomy, Sichuan Agricultural University, Chengdu, China
- 2Sichuan Engineering Research Center for Crop Strip Intercropping System, Sichuan Agricultural University, Chengdu, China
- 3Key Laboratory of Crop Ecophysiology and Farming System in Southwest China – Ministry of Agriculture, Sichuan Agricultural University, Chengdu, China
- 4College of Resources, Sichuan Agricultural University, Chengdu, China
Plants use shade avoidance strategy to escape the canopy shade when grown under natural conditions. Previous studies showed that the Arabidopsis receptor-like kinase ERECTA (ER) is involved in shade avoidance syndrome. However, the mechanisms of ER in modulating SAR by promoting hypocotyl elongation are unknown yet. Here, we report that ER regulated hypocotyl elongation in shade avoidance requires auxin and gibberellins (GAs). The T-DNA insertional ER mutant er-3 shows a less hypocotyl length than that in Col-0 wild type. Promoter::GUS staining analysis shows that ER and its paralogous genes ERECTA-LIKE1 (ERL1) and ERECTA-LIKE2 (ERL2) are differentially expressed in the seedlings, of which only ER is most obviously upregulated in the hypocotyl by shade treatment. Exogenous feeding assay by using media-application with vertical-grown of Arabidopsis seedlings showed that the hypocotyl length of er-3 is partially promoted by indol-3-acetic acid (IAA), while it is relatively insensitive of er-3 to various concentrations of IAA than that of Col-0. Hypocotyl elongation of er-3 is promoted similar to that of Col-0 by high temperature in the white light condition, but the elongation was not significantly affected by the treatment of the auxin transport inhibitor 1-N-naphthylphthalamic acid (NPA). Exogenous GA3 increased the hypocotyl elongation of both er-3 and the wild type in the shade condition, and the GA3 biosynthesis inhibitor paclobutrazol (PAC) severely inhibits the hypocotyl elongation of Col-0 and er-3. Further analysis showed that auxin biosynthesis inhibitors yucasin and L-kynurenine remarkably inhibited the hypocotyl elongation of er-3 while yucasin shows a more severe inhibition to er-3 than Col-0. Relative expression of genes regulating auxin homeostasis and signaling, and GA homeostasis is less in er-3 than that in Col-0. Furthermore, genetic evidences show that ER regulated hypocotyl elongation is dependent of PHYTOCHROME B (PHYB). Overall, we propose that ER regulated shade avoidance by promoting hypocotyl elongation is PHYB-dependent and requires auxin and GAs.
Introduction
Light is one of the most important factors for plant survival and production. In a natural environment, plants always grow closely to one another. Under these conditions, red light wavelengths is been absorbed while the far red light wavelengths is reflected by the leaves of the neighboring plants, resulting in reduction of red:far red (R:FR) light ratio and light intensity, referred to as shade condition. Plants have evolved sophisticated mechanisms which involves architecture and physiological process including increase of the hypocotyl and stem length, hyponasty, early flowering and yield reduction, which is referred to as shade avoidance syndrome (SAR) (Casal, 2013; Wit et al., 2016). In the shade, plants must accelerate their growth in order to maintain their height at least as tall as their neighboring plants to succeed in light sensing competition conditions (Ballaré, 1999). In this process, plants have to expend more energy to support their elongation growth at the expense of leaf development, seed number and yield reduction. Arabidopsis and most crops show typical phenotypes of SAR, while some other plants display shade tolerance phenotype mimicking the phenotypes of the plants in the white light condition (Valladares and Niinemets, 2008; Carriedo et al., 2016).
Upon sensing the canopy shade by the neighboring plants, phytochromes in plant cells would perceive the changes of R:FR light condition and rapidly evoke cascades of actions. Of all the five phytochromes, phytochrome A (PHYA), phytochrome B (PHYB), phytochrome C (PHYC), phytochrome D (PHYD), and phytochrome E (PHYE) in Arabidopsis, PHYB was found to play dominant roles in shade avoidance (Ballaré, 1999; Ruberti et al., 2012; Casal, 2013; Wit et al., 2016). When plants were under shade conditions, PHYB releases the binding of phytochrome interacting factor (PIF) transcription factors and facilitates their entry into the nucleus to bind the promoters of the target genes to trigger the expression of genes in regulating phytohormone levels and signaling pathways (Lorrain et al., 2008; Casal, 2013). Most phytohormones which are found to participate in several aspects of the shade avoidance signaling pathways, auxin and gibberellins (GAs) are best established to be essential for elongation-promoting of plant hypocotyls, stems and petioles (Wit et al., 2016; Yang and Li, 2017). In Arabidopsis and Brassica rapa seedlings, auxins are biosynthesized in the cotyledon when suffered to shade and cotyledon-synthesized auxins are then transported to promote the hypocotyl elongation (Tao et al., 2008; Procko et al., 2014). In these processes, genes related to plant growth and development, and adaptation are largely expressed.
Indol-3-acetic acid (IAA) is the predominant naturally occurring auxin in plants (Zhao, 2010, 2012). In higher plants, auxin biosynthesis is likely extremely complex in plants, which includes de novo auxin production and the release from auxin conjugates (Zhao, 2010, 2012, 2014). IAA exists in two forms, the free IAA and conjugated IAA, the free IAA can be converted from the conjugated IAA, which is considered as the storage forms or the intermediates for degradation (Woodward and Bartel, 2005; Ludwig-Müller, 2011; Zhao, 2014). Previous isotope-labeling experiment and genetic evidence demonstrated that auxin principally biosynthesized via tryptophan (Trp)-dependent and Trp-independent pathways to coordinately regulate plant growth and development (Wright et al., 1991; Normanly et al., 1993; Woodward and Bartel, 2005; Wang et al., 2015). More evidence showed that several Trp-dependent auxin biosynthesis pathways contribute predominantly to IAA levels referring to the indole-3-acetaldoxime (IAOx) pathway, indoleacetamine (IAM) pathway, and the indole-3-pyruvic acid (IPA) pathway, of which the IPA pathways is the well studied pathway up to date (Korasick et al., 2013; Tivendale et al., 2014; Zhao, 2014). IAA biosynthesized from the Trp by using the IPA as intermediate by a two-step pathway is the best completely established pathway (Zhao, 2012, 2014). In this pathway, Trp is first converted to IPA by TAA1/TARs and IPA is subsequently catalyzed by YUCCAs (YUCs) into IAA (Zhao et al., 2001; Tao et al., 2008). In recent years, more and more compelling evidence showed that, in addition to auxin biosynthesis, auxin transport and metabolism are also essential to hypocotyl elongation in shade avoidance (Pierik et al., 2009; Keuskamp et al., 2010; Zhao, 2010; Yang and Li, 2017). Several studies have demonstrated that auxin transport is important in hypocotyl elongation in etiolated growth, photomorphogenesis, and phototropism similar to shade avoidance response (Jensen et al., 1998; Wu et al., 2016).
When plants are exposed to the adverse environment, the external stimuli will activate the cell membrane-located receptor molecules and initiate the changes of conformation of the receptors. Receptor-like kinases (RLKs) are a set of single transmembrane proteins located on the plasma membrane which involve in sensing the environmental changes including cell-to-cell and cell-to-environment communications (Becraft, 2002; Li, 2010). A typical RLK contains an extracellular domain for signal perception, a transmembrane domain for membrane anchoring and an intercellular Ser/Thr/Tyr kinase domain for signal transduction via phosphorylation (Walker and Zhang, 1990; Shiu and Li, 2004; Oh et al., 2009; Li, 2010; Oh et al., 2010). The first plant RLK was identified from maize by using degenerate PCR primers to the protein kinase domain (Walker and Zhang, 1990). More than 610 RLKs have been found in Arabidopsis in recent years (Shiu and Bleecker, 2001; Shiu et al., 2004). Up to date, more and more RLKs have been found to function in many aspects of plant growth and development, cell death and defense (Li and Chory, 1997; Li et al., 2002; Nam and Li, 2002; Zipfel et al., 2006; Chinchilla et al., 2007; He et al., 2007; Du et al., 2012). For instance, the BRI1 was found as a receptor of brassinosteroids (BRs) (Li and Chory, 1997), BAK1 is a co-receptor of BRI1 in BR signaling pathways in regulating plant growth and development (Li et al., 2002; Nam and Li, 2002). Furthermore, BAK1 was also found as a co-receptor of FLS2 and EFR in pathogen perception and defense pathways (Zipfel et al., 2006; Chinchilla et al., 2007). ERECTA (ER) was firstly found in 1957 by using X-ray irradiated Arabidopsis Landsberg ecotype (Rédei et al., 1992). ER and its functional paralogs ERL1 and ERL2 not only control multiple aspects of plant morphology, but also regulate plant responses to environmental changes (Shpak et al., 2004). Genetic analysis has shown that the er mutant displays compact inflorescence and short blunt silique phenotypes due to the decrease in cell proliferation and growth (Shpak et al., 2003). In addition, ERL1 and ERL2 were found to play a redundant role in cell proliferation of organ growth and patterning (Shpak et al., 2004). Furthermore, ER was found to regulate transpiration efficiency in Arabidopsis (Masle et al., 2005). Overexpression of truncated Arabidopsis ER in tomato decreased water loss and enhanced drought tolerance (Villagarcia et al., 2012). Overexpression of Arabidopsis ER in Arabidopsis, rice and tomato increased plant biomass and improved thermal tolerance independent of water content (Shen et al., 2015). Single nucleotide polymorphism (SNP) analysis has shown that an ER homologous gene might be associated with drought adaptation between wild and common bean (Blair et al., 2016).
In recent studies, Patel et al. demonstrated that ER regulates petiole angle and elongation in the shade particularly at cool temperatures in Landsberg ecotype (Patel et al., 2013). It is possible that ER stimulate petiole elongation by promoting the cell expansion in the petioles. Another study also showed that ER makes an important contribution to the shade avoidance syndrome in some stages during plant development against light fluctuations (Kasulin et al., 2013). However, the mechanisms of ER in modulating the SAR are not clear yet, which needs further investigation.
Here, we report our identification of ER in regulating Arabidopsis hypocotyl elongation in the shade in Col-0 background. The results show that loss-of-function of ER displays a shorter hypocotyl length than that of Col-0 wild type in the shade condition. Promoter::GUS analyses show that the expression of ER is remarkably induced in the hypocotyl by shade treatment. Further investigation show that ER regulated hypocotyl elongation is probably via actions of auxin and GAs, and is dependent of PHYB. Our data supplied a new identification of ER in shade avoidance and detailed a possible mechanism of ER regulated shade avoidance underlying the involvement of auxin homeostasis and signaling pathways, as well as GA homeostasis, which provides new evidence and mechanisms for ER regulated shade avoidance.
Materials and Methods
Plant Materials and Growth Conditions
All the Arabidopsis seeds used in these studies were Col-0 ecotype. Seeds of er-3 (SALK_044110) (Durbak and Tax, 2011), phyB (SALK_022035C) were ordered from Arabidopsis Biological Resource Center (ABRC). Plants were grown at 22°C in a long-day growth condition (16 h of light and 8 h of dark) in a greenhouse except those for special treatments.
Arabidopsis seeds were surface-sterilized and grown in the soil or on the 1/2 Murashige and Skoog (MS) media (pH 5.7) supplemented with 1% sucrose and 0.8% agar. For shade treatment of soil-grown plants, 10-day-old Arabidopsis Col-0 and er-3 single mutant grown in a normal light condition (16 h of light and 8 h of dark) with PAR of 65 μmol m-2 s-1 and R/FR of 1.3) in a greenhouse and then transferred to a green filter (type No. 122 with a transmittance of 45.6%1, England) with PAR of 31 μmol m-2 s-1 and R/FR of 0.5 for another 15 days. For shade treatment of media-grown plants, plants were grown in normal light condition for 3 days and then treated with the green filter for another 5 days. Plants were then used for further analyses. Unless it is specially stated that, the plants were grown on slightly vertical 1/2 MS media.
Semi-quantitative Reverse Transcription (RT)-PCR and Quantitative PCR (qPCR) Analyses
Two micrograms of total RNA were extracted by using an RNAprep pure Plant Kit (Tiangen Biotech) used for reverse transcription with M-MLV (Invitrogen). The first strand of cDNAs was used for semi-quantitative reverse transcription PCR (RT-PCR) analyses with ExTaq DNA polymerase (TaKaRa) according to previous studies (Du et al., 2012). Real-time PCR was employed with SYBR® Premix Ex TaqTM II (TaKaRa) and relative expression of genes compared to ACT2 was calculated using ΔΔCt method. Primers used in this study are listed in Supplementary Table S1.
Promoter::GUS Construction of ER Family Genes and GUS Staining
Promoters of ER, ERL1 and ERL2 with 1.5 kb were amplified from genomic DNA and cloned with a Gateway® Cloning technology (Invitrogen). The genes were recombined into a binary vector pBASTA-GWR-GUS and the destination plasmids were then overexpressed in Col-0 with Agrobacterium-mediated transformation. Surface-sterilized seeds of homozygous transgenic plants harboring promoter-GUS were grown on 1/2 MS media. After stratification for 2 days at 4°C, the plates were grown vertically at 22°C in white light for 3 days, and then transferred to white light or shade conditions for another 5 days for GUS staining. For GUS staining, ER, ERL1, or ERL2 promoter-GUS transgenic seedlings were stained according to previous studies (Guo et al., 2010). The stained plants were observed and for photo-capture under a Leica M165C digital stereo microscope, the images were subsequently arranged by using the Adobe Photoshop CS6 software.
Treatment of Phytohormones and the Biosynthesis Inhibitors
Surface-sterilized seeds were grown on 1/2 MS plates supplemented with 1% sucrose, 0.8% agar and different concentrations of IAA (Sangon), GA3 (Sangon), the auxin biosynthesis inhibitors yucasin and L-kynurenine (kyn) (Sigma-Aldrich), and the GA biosynthesis inhibitor paclobutrazol (PAC) (Sangon). The seedlings were grown at a slight angle with the hypocotyl touching the media. Stock solutions of the phytohormones and the inhibitors were dissolved as follows: 20 mM of IAA were dissolved in ethanol, 20 mM of GA3 in methanol, 500 mM of yucasin in DMSO, 100 mM of kyn in 0.5 M HCl and 5 mM PAC in methanol, respectively. ddH2O was used for dilution for the working concentrations. The hypocotyl length was measured with ImageJ 1.6 and analyzed with Graphpad 5.0.
Results
Loss-of-Function of ER Is Shade Insensitive in Hypocotyl Elongation
To understand whether ER regulates hypocotyl elongation in shade response, er-3 (Durbak and Tax, 2011), a T-DNA insertional loss-of-function mutant of ER, was used to assess the shade response. After 3-day white-light growth, Col-0 and er-3 were then moved to the shade condition for another 5 days. The results showed that the hypocotyl length of er-3 were significantly less sensitive to shade treatment than that of Col-0 in our shade condition (Figures 1A,B). To further confirm the shade response phenotypes of er mutant, we grew the Col-0 and er-3 seeds in the soil and covered a green filter for shade treatment. In the shade condition, both soil-grown Col-0 and er showed a typical shade avoidance response including petiole elongation (Figures 1C,D). Nevertheless, the petiole elongation was shorter in er-3 single mutant than that in Col-0 in the shade, indicating that petiole elongation of er-3 is relatively insensitive to shade, which is consistent with other er alleles in previous studies (Patel et al., 2013). These results suggest that ER in Col-0 ecotype makes a contribution to shade avoidance syndrome.
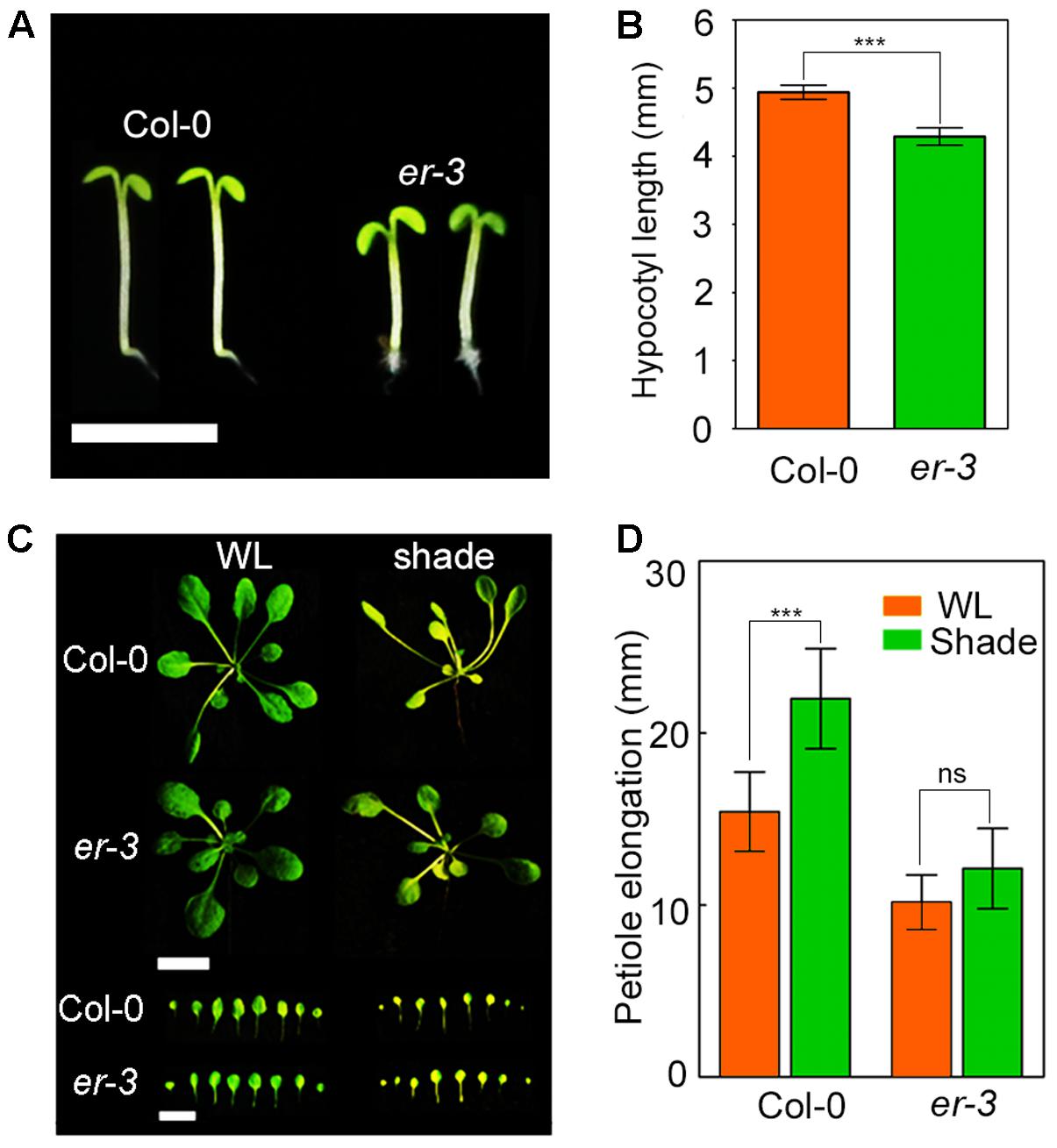
FIGURE 1. Phenotypes of Col-0 and er-3 single mutant in shade avoidance. (A) Phenotypes of Col-0 and er-3 grown on MS media under the shade conditions. Scale bar represents 5 mm. (B) Statistical data of hypocotyl length of Col-0 and er-3 under white light and shade conditions. At least 30 seedlings were measured for each genotype. Error bars represent SE. (C) Phenotypes of Col-0 and er-3 grown in the soil under white light and shade conditions. Scale bars represent 10 mm. (D) Statistical data of petiole length of Col-0 and er-3 grown in the soil under white light and shade conditions. At least 30 seedlings were measured for each genotype. Error bars represent SE. Student’s t-test indicated the differences are statistically significant (∗∗∗P < 0.001).
Expression of AtER in Hypocotyl Is Upregulated by Shade
To examine whether the transcript level of ER in the hypocotyl of Arabidopsis responds to shade, we performed RT-PCR analysis to check gene expression by the time-course shade treatment. The results showed that the shade inducible gene PIL1 was upregulatedly expressed in the hypocotyls of Col-0 after 30 min by shade treatment and increased as time rises (Supplementary Figure S1), indicating that our shade condition is reliable for study on the shade avoidance syndrome. Under this shade condition, we further found that the expression of ER was upregulated in the hypocotyls of Col-0 after 30 min and reached at a highest level after 2 h of shade treatment (Supplementary Figure S1). These results indicate that ER is responsive to shade at the transcription level, suggesting that ER might function in shade avoidance pathways in the hypocotyl of Arabidopsis.
ER Family Genes Are Differentially Responsive to Shade
There are three ER family genes, ER and its paralogous genes ERL1 and ERL2 in the Arabidopsis genome. To investigate whether ER family members are responsive to shade stress, we constructed expression vectors harboring a GUS reporter gene driven by the native promoters of ER family genes. As shown in Figure 2, ER is principally expressed in the young tissues and the hypocotyls when grown in the white light, whereas slightly expressed in the cotyledons. However, ER shows a higher expression level in the cotyledon, petiole and hypocotyl in the shade condition. ERL1 is slightly expressed on the leaf margin, petiole and meristem, and its expression is remarkably induced in the meristem by shade but only slightly induced in the hypocotyl. The expression of ERL2 is mainly distributed on the leaf margin and the meristem of the seedling, whereas downregulated by shade in the leaves and obviously upregulated in the meristem. These results indicate that ER family genes differentially respond to shade stress and only the expression of ER is most obviously increased in the hypocotyls, suggesting that ER might contribute more to shade avoidance rather than ERL1 and ERL2 in the hypocotyls.
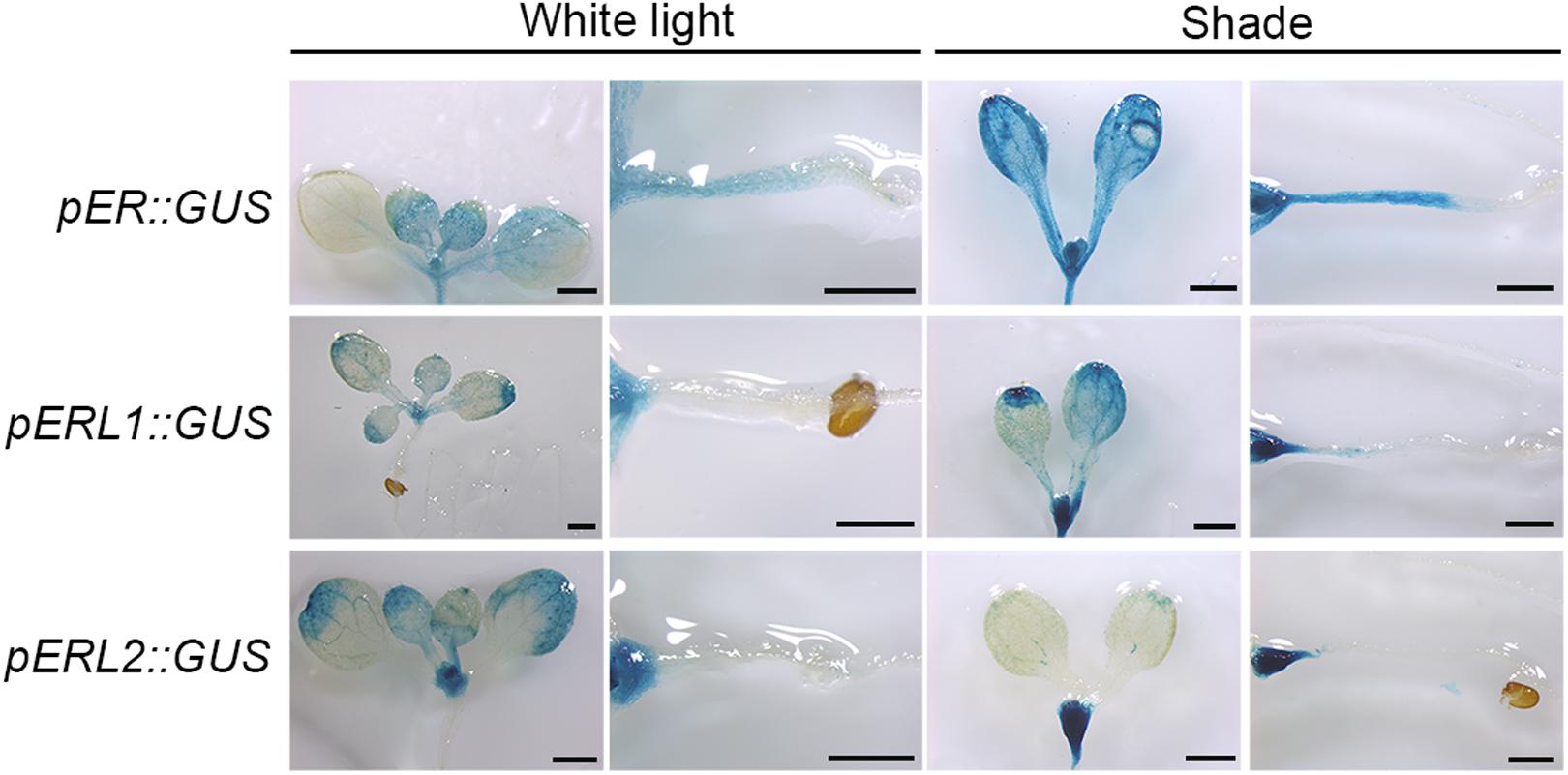
FIGURE 2. Spatiotemporal expression pattern of ER family genes in the white light and shade. Scale bars represent 0.5 mm. pER::GUS staining of 5-day shade treated seedlings show differential expression of ER, ERL1 and ERL2 under white and shade conditions.
Exogenous Auxin Promoted the Hypocotyl Length of er-3 in Shade Response
To examine whether er-3 inhibited hypocotyl elongation in the shade is impaired in auxin biosynthesis, we determined the response of er-3 to indole-3-acetic acid (IAA), the main natural auxin. As shown in Figure 3, 0.1 and 0.5 μM of IAA remarkably promoted the hypocotyl elongation of both Col-0 and er-3, and 0.5 μM of IAA fully rescued the hypocotyl length of er-3 than that of Col-0 without IAA treatment. The results indicate that IAA is essential for ER regulated hypocotyl elongation and IAA biosynthesis might be impaired in er-3 in the shade. Further, higher concentrations of IAA inhibit the elongation of both Col-0 and er-3, but er-3 shows a shorter hypocotyl phenotype than that of Col-0 by the same concentration of IAA treatment (Figures 3A,B), implying that auxin signaling is also diminished in the er-3 mutant. These results suggested that both auxin biosynthesis and signaling pathways might be impaired in the er-3 mutant in the shade condition.
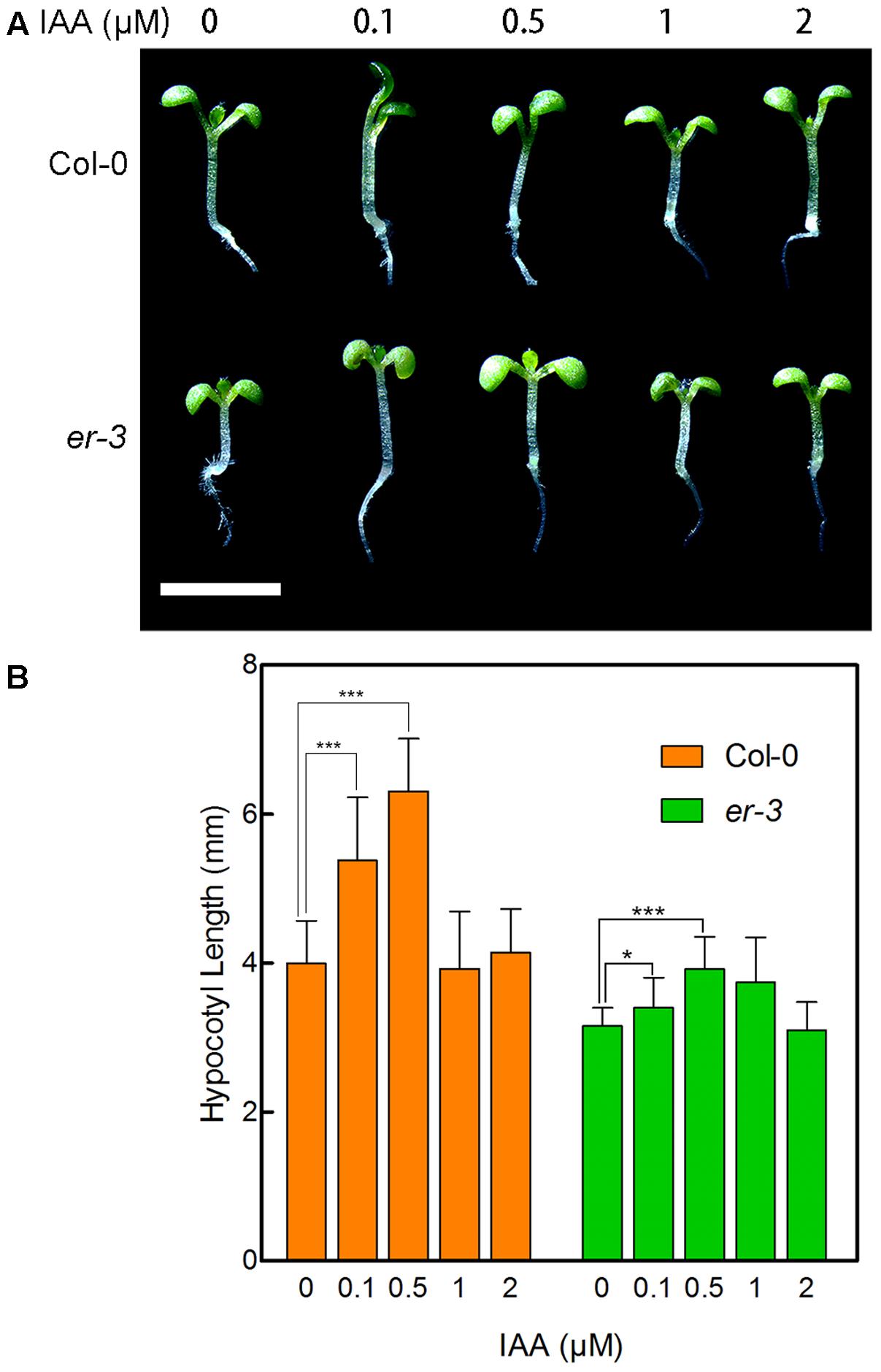
FIGURE 3. Hypocotyl phenotypes of Col-0 and er-3 in response to IAA. (A) Hypocotyl phenotypes Col-0 and er-3 seedlings with different concentrations of IAA treatment. (B) Statistical data of hypocotyl length of Col-0 and er-3 by IAA treatment under shade conditions. Student’s t-test indicated the differences are statistically significant (∗P < 0.05; ∗∗∗P < 0.001). At least 10 seedlings were measured for each genotype. Error bars represent SE. Scale bars represent 0.5 cm.
Genetic and physiological evidences show that IAA is principally biosynthesized from the precursor tryptophan via TRYPTOPHAN AMINOTRANSFERASE of ARABIDOPSIS/SHADE AVOIDANCE 3 (TAA1/SAV3), which catalyzes the production of IPA from L-tryptophan (L-Trp), then the IPA is as the substrate of YUC proteins to produce IAA (Zhao et al., 2001; Tao et al., 2008; Dai et al., 2013). Chemical library screening assay showed that kyn is a Trp analog as an effective competitive inhibitor of TAA1/TARs (TRYPTOPHAN AMINOTRANSFERASE RELATEDs) in Arabidopsis, which can effectively block the steps of Trp to IPA (He et al., 2011). In IPA to IAA steps, yucasin was recently found as a potent inhibitor of YUC enzymes in the major IAA biosynthesis pathways (Nishimura et al., 2014). To further understand whether ER regulated shade avoidance is deficient in auxin biosynthesis and in which step of auxin biosynthesis pathways, the effects of kyn and yucasin on Col-0 and er-3 single mutant were investigated. In the shade condition, the results showed that the hypocotyl elongation of both vertically grown Col-0 and er-3 at a slight angle are inhibited by both kyn and yucasin treatment (Supplementary Figures S2A,B). However, elongation of er-3 shows less sensitive to kyn and yucasin than that of the wild type (Supplementary Figures S2A,B), suggesting that ER regulated hypocotyl elongation might depend on conversion of both Trp to IPA and IPA to IAA. It is reported that in some growth conditions, the plant appears thigmotropic response (Meng et al., 2012). To clarify that whether the inhibition of hypocotyl elongation by kyn and yucasin is resulted from the thigmotropism, we also test the horizontal growth of the plants on agar plates (0.5% agar) feeding by IAA biosynthesis inhibitors in the shade condition. Plants showed a similar inhibition of the hypocotyl elongation of Col-0 and er-3 by both kyn and yucasin to that grown vertically at a slight angle (Supplementary Figures S2A,C). The inhibition of the hypocotyl elongation of Col-0 and er-3 by IAA biosynthesis inhibitor therefore seems to be independent of thigmotropism. These results indicate that auxin biosynthesis is essential for the hypocotyl elongation of Col-0 and er-3 stimulated by shading.
High Temperature Increases Shade-Stimulated Hypocotyl Elongation of er-3
Previous studies demonstrated that high temperature can increase the endogenous free auxin levels to promote the hypocotyl elongation (Gray et al., 1998). To test whether er-3 regulated hypocotyl elongation in the shade is dependent on endogenous auxin levels, we tested the hypocotyl elongation response of er-3 to a high temperature of 30°C. The results showed that, similar to shade avoidance syndrome, hypocotyl elongation of both Col-0 and er-3 is enhanced by high temperature even in the white light condition, and hypocotyl length of er-3 is rescued similar to that of Col-0 by the same high temperature treatment, indicating that endogenous auxin level is essential for ER-modulated hypocotyl elongation. To further investigate that whether auxin transport is also essential for promoting the hypocotyl elongation of er-3 by endogenous IAA increase, we use a polar auxin transport inhibitor 1-N-naphthylphthalamic acid (NPA) treatment at 30°C. As shown in Supplementary Figure S3, NPA could slightly inhibit the hypocotyl length of both Col-0 and er-3 that increased by high temperature in the white light, while the hypocotyl length shows no significance between Col-0 and er-3 by 5 μM of NPA treatment. These results suggest that ER-mediated hypocotyl elongation is dependent on endogenous auxin levels but not remarkably depends on auxin transport.
Exogenous GA3 Increased the Hypocotyl Length of er in the Shade
Gibberellins are another important group of phytohormones required to regulate the hypocotyl elongation in shade avoidance. To understand whether ER modulated hypocotyl elongation is deficient in GA biosynthesis, the effects of various concentrations of GA3 on the hypocotyl elongation of er-3 and Col-0 were investigated (Figure 4). The results showed that lower concentrations of GA3 can promote the hypocotyl elongation of both Col-0 and er-3, and the promotion of the hypocotyl length in er-3 is less than that in Col-0 by concentrations of 0.5 and 1 μM of GA3 (Figures 4A,B). However, 2 μM of GA3 can stimulate the hypocotyl elongation of er-3 similar to that of Col-0 (Figures 4A,B), indicating that the ER regulated hypocotyl elongation is partially dependent of GAs. Furthermore, the hypocotyl elongation of both Col-0 and er-3 are impaired to the similar length by the GA biosynthesis inhibitor PAC (Figures 4A,B), indicating that GAs are important, but not only specific, for ER-mediated shade avoidance. These results suggest that GA level is an important regulator for hypocotyl growth for both the wild type and er-3 mutant in the shade.
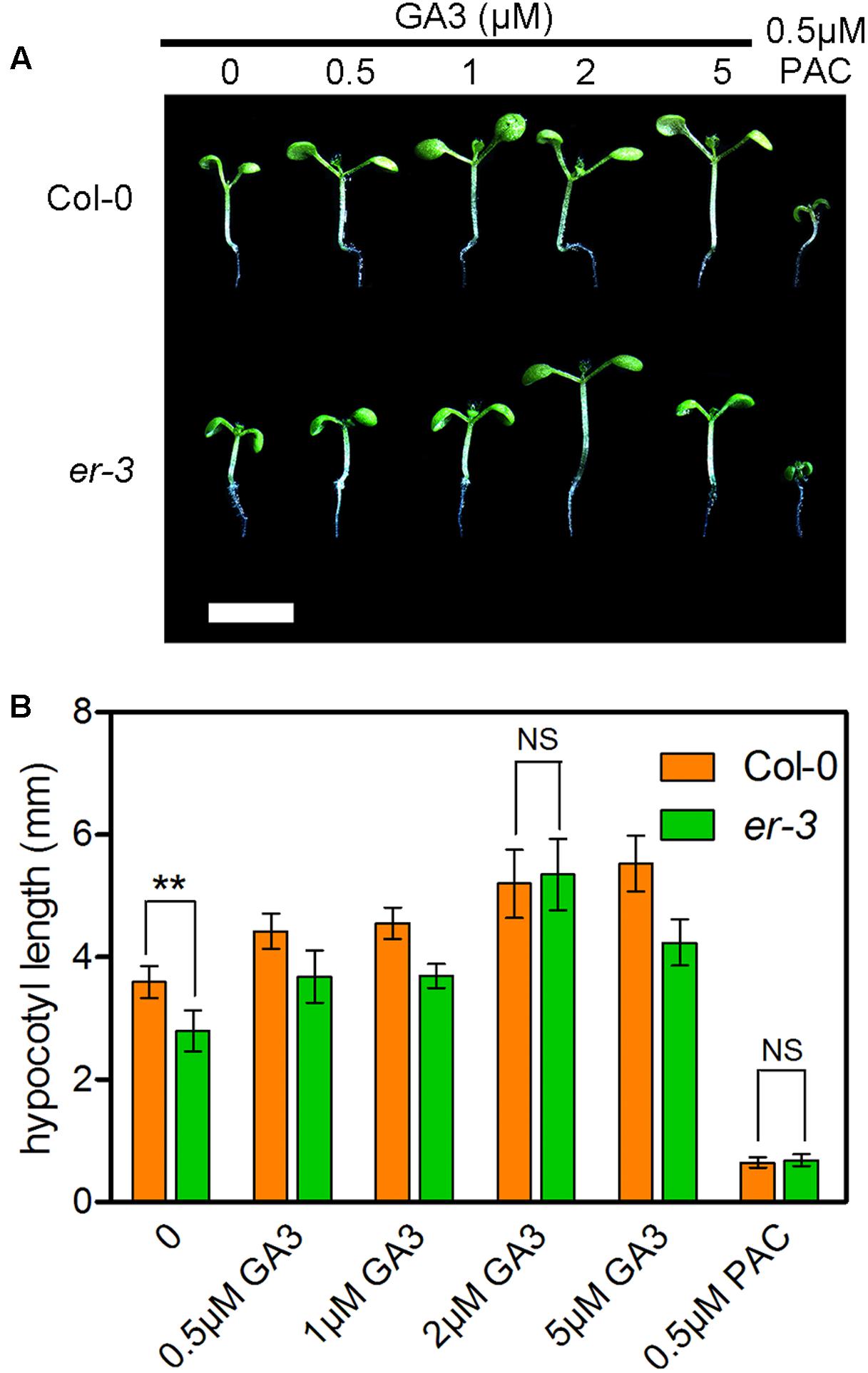
FIGURE 4. Exogenous feeding of GA3 enhanced the hypocotyl elongation of both Col-0 and er-3 in the shade. (A) Hypocotyl phenotypes of Col-0 and er-3 by different concentrations of GA3 and PAC treatment. Scale bar represents 0.5 cm. (B) Statistical data of hypocotyl length of Col-0 and er-3 by treatment of GA3 and PAC under shade conditions. Student’s t-test indicated the differences are statistically significant (∗∗P < 0.01). At least 10 seedlings were measured for each genotype. Error bars represent SE.
Auxin- and GA-Related Genes Are Differentially Regulated by ER in the Shade
To further elucidate the molecular mechanisms of ER modulated hypocotyl growth, genes related to auxin and GA biosynthesis and signaling pathways were examinated. Auxin biosynthesis gene YUC9 and auxin-responsive genes, IAA29 and SAUR68, whose expression is also rapidly upregulated by shade (Tao et al., 2008; Galstyan et al., 2011), are upregulated in both Col-0 and er-3 under shade condition, and that the expression level is higher in Col-0 than that in er-3, suggesting that ER mutation impairs the expression level of auxin biosynthesis gene YUC9 and auxin response might be impaired in er-3 in the shade. Previous studies revealed that VAS2, encoding an IAA-amido synthetase Gretchen Hagen 3 (GH3).17, is expressed predominantly in the hypocotyl and plays important role in conversion of free IAA to IAA-Glu (IAA-glutamate) independent of IPA-mediated IAA biosynthesis (Zheng et al., 2016). To test whether ER regulated hypocotyl elongation in the shade is dependent on VAS2, we checked the expression level of VAS2 in Col-0 and er-3. The results showed that relative expression of VAS2 is decreased in Col-0 by shading compared to that in the white light condition. While VAS2 is significantly upregulated in shade-treated er-3 compared to that in er-3 grown in the white light, and is much higher in expression than that of Col-0 in the shade condition. These results indicate that VAS2 might also contribute to ER-regulated hypocotyl elongation in the shade.
The GA biosynthetic genes GA20OX1, GA3OX1, and GA1 were induced in the shade treated both Col-0 and er-3, respectively. While expression of GA3OX1 is less in er-3 than that in Col-0, indicating that GA biosynthesis might be diminished in the conversion of GA9/GA20 to GA4/GA1. Expression of GA1 is induced in both Col-0 and er-3 by shade, whereas the expression level is lower in er-3 than that in Col-0 (Figure 5). However, the expression of the GA catabolic gene, GA2OX1 is sharply decreased in both shade treated Col-0 and er-3 than that in white light-treated plants. GA2OX1 exhibit a slightly less expression in shade treated er-3 than that in shade-treated Col-0, suggesting that conversion of copalyl diphosphate (CPP) from geranylgeranyl diphosphate (GGPP) in GA biosynthesis might be impaired in er-3. However, significant changes in expression of GA biosynthesis and catabolic genes were not more remarkable than that of genes in auxin homeostasis and signaling pathways, implying that auxin might contribute more to ER-regulated hypocotyl elongation than GA in the shade.
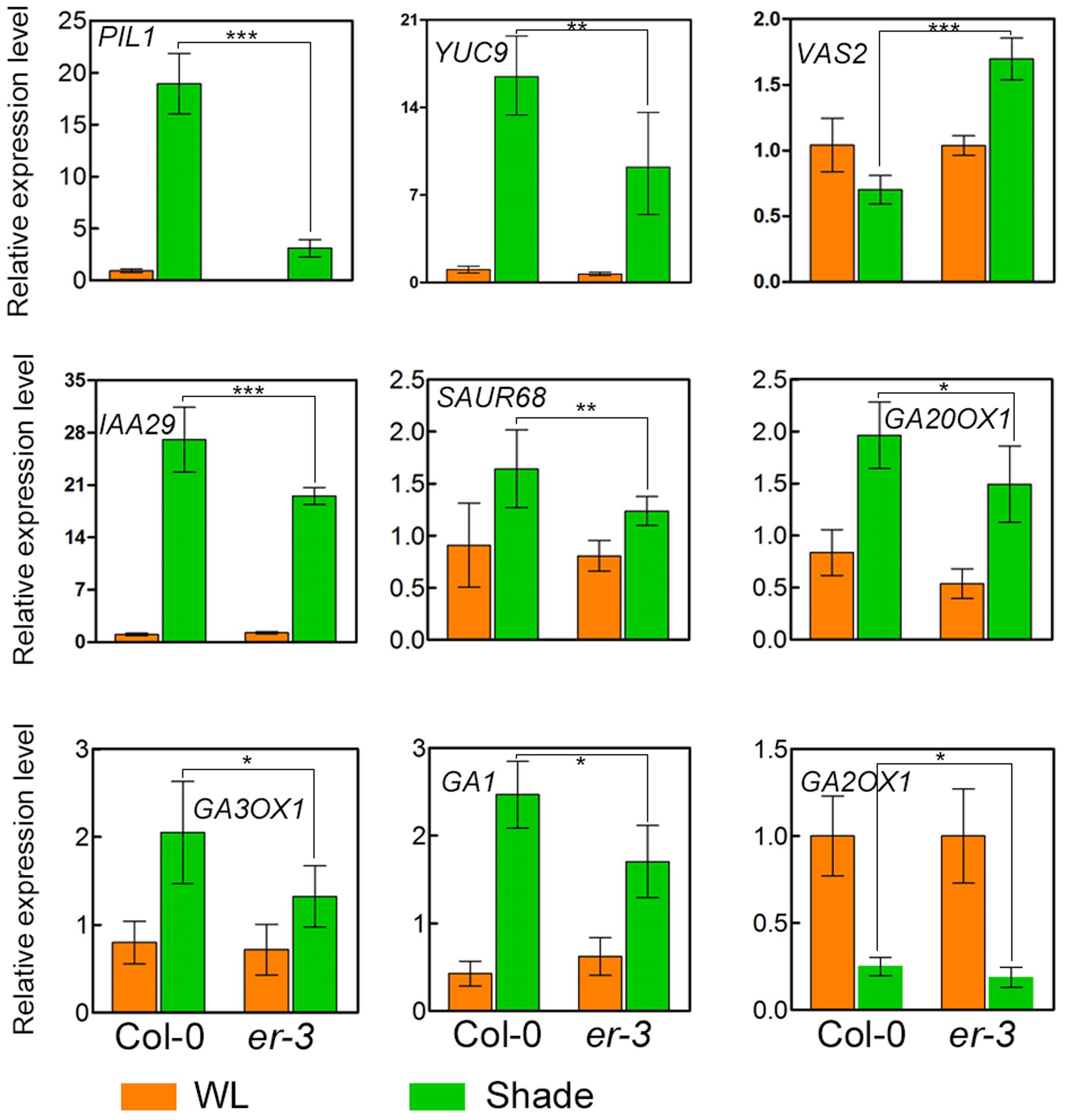
FIGURE 5. Relative expression of genes in Col-0 and er-3 under white light and shade conditions. Expression of the shade-inducible gene PIL1, auxin biosynthesis gene YUC9, auxin homeostasis gene VAS2, auxin signaling gene IAA29 and SAUR68, GA biosynthesis gene GA20OX1, GA3OX1 and GA1 and GA catabolism gene GA2OX1 were examined by using real time PCR analysis. Similar results from two biological repeats were obtained and at least three technical repeats in each biological assay were performed for the gene expression analysis and a presentative one is shown. Student’s t-test indicated the differences are statistically significant (∗P < 0.05; ∗∗P < 0.01; ∗∗∗P < 0.001).
ER Regulated Hypocotyl Elongation in Response to Shade Is Dependent of PHYB
Pants use red and far-red light-absorbing phytochromes A and B to sense the changes of R:FR ratio, of which phytochrome B (PHYB) play dominant role in shade avoidance inhibition. To determine whether ER-controlled hypocotyl elongation is via PHYB, we crossed er-3 with a T-DNA insertional mutant phyB to generate an er-3 phyB double mutant (Figure 6A). In the white light condition, Col-0 and er-3 single mutant show a hypocotyl inhibition phenotype, and phyB single mutant shows a hypocotyl elongation phenotype compared with that of Col-0 and er-3 single mutant. However, er-3 phyB double mutant displays a hypocotyl elongation phenotype mimicking phyB single mutant but ER mutation significantly reduced hypocotyl elongation of phyB (Figures 6A,B). These results indicate that PHYB inhibited hypocotyl elongation in the white light is ER dependent. In the shade condition, er-3 single mutant shows a shade avoidance phenotype in the hypocotyl, but elongation of the hypocotyl of er-3 was significantly insensitive to shade compared with Col-0. Hypocotyls of both phyB and er phyB mutants also elongated in the shade condition. However, er-3 phyB is less sensitive to shade compared to the phyB single mutant, but er-3 phyB double mutant shows more sensitive in hypocotyl elongation compared to that of er single mutant, indicating that ER promoted hypocotyl elongation depends on PHYB (Figures 6A,B).
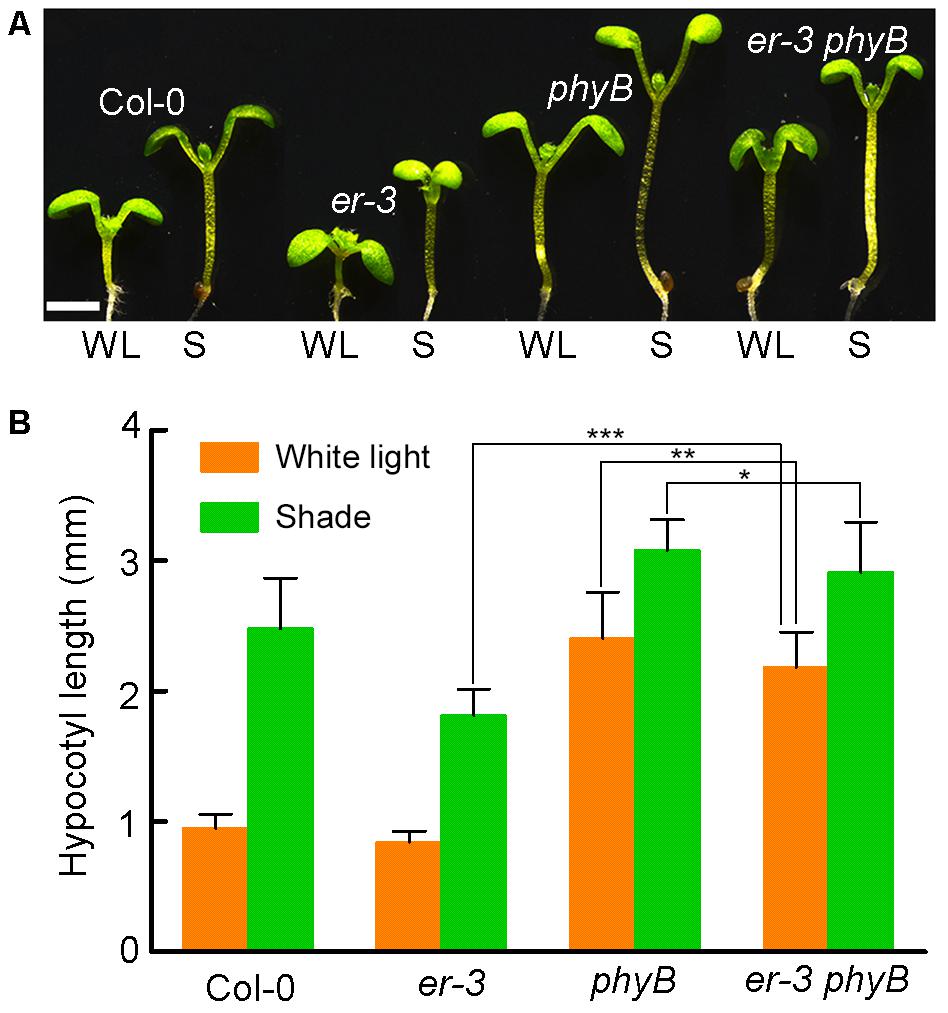
FIGURE 6. ER promoted hypocotyl elongation is dependent of PHYB in the shade. (A) Phenotypes of Col-0, single and double mutants of er-3 and phyB grown in white light and shade conditions. Scale bar represents 1 mm. (B) Statistical data of hypocotyl length of Col-0, er-3 and phyB mutants in white light and shade conditions. Student’s t-test indicated the differences are statistically significant (∗P < 0.05; ∗∗P < 0.01; ∗∗∗P < 0.001). At least 10 seedlings were measured for each genotype. Error bars represent SE.
Discussion
Hypocotyl growth is stimulated by various factors from internal signals and surrounding environment and controlled by complicated signaling networks. Plant receptor-like kinases play critical roles in perception of environmental signals. However, researches on RLK-mediated shade avoidance are seldom reported. Previous studies demonstrated that the leucine-rich repeat receptor-like kinase ER not only regulates Arabidopsis growth and development, but also plays a role in response to environmental stimuli (Torii et al., 1996; van Zanten et al., 2009; Shpak, 2013). Our study demonstrated that a T-DNA insertional mutation of Arabidopsis ER in Clo-0 background inhibits hypocotyl elongation in shade avoidance. Previous studies showed that Arabidopsis ER regulates petiole elongation and leaf hyponasty response in shade avoidance in a temperature-dependent manner in the Arabidopsis Landsberg ecotype (Patel et al., 2013). Another study from an independent group showed that ER contributes to hypocotyl, petiole and lamina elongation, hyponastic growth and flowering time in response to end-of-day far-red (R/FR) light in a genetic background-dependent manner (Kasulin et al., 2013). However, mechanisms of ER controlled shade avoidance are unknown yet.
Our physiological evidence revealed that ER regulates hypocotyl elongation in shade avoidance probably via auxin homeostasis and signaling pathways, and GA homeostasis pathways. Firstly, expression pattern analyses showed the expression of ER, but not its homologs ERL1 and ERL2, which is specifically induced by shade in the Arabidopsis hypocotyl, suggesting that ER might play roles in hypocotyls in shade avoidance distinct from ERL1 and ERL2. Moreover, a cis-acting regulatory element prediction of the ER promoter region by PlantCARE showed that 14 light responsive elements are found in the promoter of ER (Supplementary Figure S4), providing a clue that ER might involve in light responsiveness. Secondly, exogenous IAA feeding promoted the hypocotyl elongation of both Col-0 and er-3 in the shade, while exogenous IAA only partially promoted the hypocotyl elongation of er-3 comparing to that of the wild type, suggesting that ER-mediated hypocotyl elongation might be partially dependent of auxin biosynthesis. Nevertheless, er-3 was relatively insensitive to IAA treatment than the wild type in the same concentration of IAA in the shade (Figure 3), implying that the auxin signaling pathways might be also impaired in the er-3 mutant compared to the wild type in the shade. Previous studies showed that indole-3-pyruvic acid (IPyA) pathway is a major IAA biosynthesis pathway from tryptophan in Arabidopsis, in which the tryptophan aminotransferase SAV3/TAA1 and YUC enzymes play principal roles (Zhao et al., 2001; Tao et al., 2008; Dai et al., 2013). By using a chemical biology approach, Nishimura et al. discovered a potent IAA biosynthesis inhibitor, 5-(4-chlorophenyl)-4H-1,2,4-triazole-3-thiol (yucasin), which can effectively inhibit the activities of YUC thus impaired IAA production in planta (Nishimura et al., 2014). Another study showed that kyn is a tryptophan analog which can effectively inhibit Arabidopsis SAV3/TAA1/TARs identified from a chemical library screen (He et al., 2011). Our results showed that both yucasin and kyn can effectively inhibit the hypocotyl elongation of Col-0 and er-3, suggesting that both yucasin and kyn are potent inhibitors for Arabidopsis in the shade. Thirdly, it is known that high temperature can increase the endogenous auxin levels to promote the hypocotyl elongation of Arabidopsis and the expression of auxin biosynthesis gene YUC9 is also induced by shade (Gray et al., 1998; Müller-Moulé et al., 2016), our results revealed that high temperature can enhance the hypocotyl elongation of er-3 similar to that of Col-0 in the white light condition (Supplementary Figure S3) and auxin biosynthesis gene YUC9 is less induced by shade in er-3 than that in Col-0 (Figure 5), indicating that endogenous auxin homeostasis makes an important contribution to ER-mediated hypocotyl elongation in the shade. This conclusion also supported by another recent study which revealed that auxin biosynthesis is essential for ER regulated cell elongation in the hypocotyl under white light (Qu et al., 2017). It is reported that VAS2 controls the endogenous free IAA levels by conversion from IAA-Glu in the hypocotyl epidermis to promote the hypocotyl elongation (Zheng et al., 2016). Our qPCR results showed that relative expression of VAS2 is down-regulated in Col-0 but upregulated in er-3 by shading (Figure 5), suggesting that auxin metabolism might also contribute to ER-mediate shade avoidance in hypocotyl elongation. Auxin transport also plays roles in plant hypocotyl elongation in the white light condition (Jensen et al., 1998; Wu et al., 2016), our results indicated that auxin transport might be not essential for ER-regulated hypocotyl elongation, in that NPA treatment cannot cause the significant reduce in hypocotyl elongation of er-3 compared to that of Col-0 in white light with high temperature conditions. These results suggested that auxin transport seems to be less important than auxin homeostasis in ER-mediated hypocotyl elongation in shade avoidance.
To date, the mechanisms of actions for auxin transport inhibitors have remained poorly understood (Teale and Palme, 2017). Although our physiological assays suggested that auxin transport inhibitors did not directly significantly affect the hypocotyl growth of er-3 than that of Col-0, previous studies reported that long-term application of high concentrations of auxin to the roots leads to changes of auxin transport probably through auxin-dependent transcriptional control (Sieberer et al., 2000; Vieten et al., 2005; Vanneste and Friml, 2009). Moreover, high temperature is able to impact on membrane fluidity vesicular trafficking, and other hormonal responses (Kim and Portis, 2005; Asensi-Fabado et al., 2013; Hanzawa et al., 2013). For this reason, we speculate that it is also probably that high temperature induced auxin maxima in the roots or other processes and thus resulted in changes of auxin transport. In addition, several evidences has revealed that NPA probably binds either directly to the auxin efflux carrier including PIN and ABC-transporters (Rubery, 1979; Sussman and Goldsmith, 1981) or to auxin efflux-related regulatory proteins and cytoskeleton (Cox and Muday, 1994; Bailly et al., 2008) to inhibit auxin transport, but it has also been proposed that NPA may have other effects independent of auxin transport (Hössel et al., 2005). Altogether, we could not rule out that auxin balance in the hypocotyl of er-3 might be neither affected by a loss or accentuation of an auxin maxima in the root, leading to alteration of auxin transport, nor affected by other biological processes, which might finally result in changes of ER-mediated hypocotyl elongation. In the future, more molecular and physiological evidences are required to uncover whether and how auxin transportation involving auxin transporters contributes in ER-regulated hypocotyl growth in shade avoidance.
Our exogenous GA3 feeding increased the hypocotyl length of er-3 to the length of the wild type in the shade, suggesting that GA3 biosynthesis might be diminished in er-3 under shade condition. Relative expression of genes in GA biosynthesis and catabolism pathways in er-3 are less than that in the wild type, suggesting that ER modulates hypocotyl elongation might depend on GA homeostasis. Nevertheless, more genetic and biochemical evidences need to be done to elucidate the detailed mechanisms underlying the crosstalk between auxin and GA in ER regulated shade avoidance in the future. PHYB is the main photoreceptor in perception of changes of light quality of red to far-red light wave length. Previous studies show that ER modulates low light intensity induced petiole elongation independent of PHYB (van Zanten et al., 2010). Our genetic evidence shows that loss-of-function of PHYB can promote the hypocotyl elongation of er-3, whereas the hypocotyl length of the double mutant of er-3 phyB is shorter than that of phyB single mutant, suggesting that ER regulated hypocotyl elongation depends on PHYB, and phyB-mediated promotion of hypocotyl elongation is partially dependent of ER. However, the detailed molecular mechanisms of how does PHYB function in ER-mediated shade avoidance pathways needs further investigation. Interestingly, protein–protein interaction analyses by Search Tool for the Retrieval of Interacting Genes/Proteins (STRING2) show that ER and PHYB are in the same protein complex (Supplementary Figure S5), thus we speculate that it is probably ER and PHYB function together in hypocotyl elongation in a same protein complex. Furthermore, extensive evidence showed that RLKs play roles in plant growth and development, and defense with their co-receptors as receptor complex in previous studies. For instance, the BR receptor BRASSINOSTEROID INSENSITIVE 1 (BRI1) interacts with its co-receptors SOMATIC EMBRYOGENESIS RECEPTOR KINASEs (SERKs) to perceive and transduce BR signals to regulate plant growth and development (Li et al., 2002; Nam and Li, 2002; Gou et al., 2012). EF-TU RECEPTOR (EFR) and FLAGELLIN-SENSING 2 (FLS2) interacts with SERKs to sense the flagellin22 in plant defense signaling pathways (Zipfel et al., 2006; Chinchilla et al., 2007; Roux et al., 2011; Stegmann et al., 2017). ER family receptors interact with a receptor-like protein TOO MANY MOUTH (TMM) to form a homo- and heterodimer receptor complex to regulate stomatal development (Shpak et al., 2005). Receptor-like kinases FERONIA and THESEUS1 were found to control the shoot elongation of Geranium pyrenaicum identified by transcriptome analyses (Gommers et al., 2017). We therefore hypothesize that ER may function with unknown co-receptors to regulate hypocotyl elongation in shade avoidance by regulating the auxin homeostasis and signaling and GA homeostasis related networks. Further studies will focus on disclosing the detailed molecular mechanisms, including the crosstalk of auxin and GA in ER regulated hypocotyl elongation in shade avoidance.
Author Contributions
JD and WY designed the experiments. JD, HJ, XS, YaL, MS, YiL, ZF, QC, LF, JS, KS, JL, WL, FY, TY, XW, SY, LY, and CL performed the experiments. JD, HJ, XS, and YaL analyzed the data. JD, HJ, XS, and YaL wrote the manuscript.
Funding
This work was supported by the National Natural Science Foundation of China grants 31401308, 31671445, and 31371555.
Conflict of Interest Statement
The authors declare that the research was conducted in the absence of any commercial or financial relationships that could be construed as a potential conflict of interest.
Acknowledgments
The authors thank Dr. Tomokazu Koshiba at Tokyo Metropolitan University for generously providing yucasin and Mr. George Bawa and Muhmmad Ahsan Asghar for reading and commenting the manuscript. They are also grateful to the Arabidopsis Biological Resource Center (ABRC) for providing some of the T-DNA insertional mutant seeds.
Supplementary Material
The Supplementary Material for this article can be found online at: https://www.frontiersin.org/articles/10.3389/fpls.2018.00124/full#supplementary-material
FIGURE S1 | Time course of gene expression in Col-0 induced by shade. ER and the shade inducible genes PIL1 and XTR7 were upregulated in the shade. ACT2 was amplified for 23 cycles as an internal control.
FIGURE S2 | Exogenous feeding of kyn and yucasin can inhibit shade stimulated hypocotyl elongation. (A) Phenotypes of Col-0 and er-3 seedlings vertically with a slight angle or horizontally grown with treatment of different concentrations of kyn and yucasin in the shade. Scale bar represents 1 cm. (B) Statistical data of hypocotyl length of vertically grown Col-0 and er-3 with a slight angle treated by kyn and yucasin under shade condition. (C) Statistical data of hypocotyl length of horizontally grown Col-0 and er-3 treated by kyn and yucasin under shade condition. Student’s t-test indicated the differences are statistically significant (∗∗∗P < 0.001). At least 15 seedlings were measured for each genotype. Error bars represent SE.
FIGURE S3 | High temperature promoted hypocotyl elongation of both Col-0 and er-3. (A) Phenotype of Col-0 and er-3 treated with 22°C and 30°C in the while light or shade conditions. 5 μM of NPA feeding were used at 30°C in the shade. (B) Statistical data for Col-0 er-3 with/without NPA treatment grown at 22°C and 30°C in the while light or shade conditions.
FIGURE S4 | Motif prediction of the promoter of ER by PlantCARE (http://bioinformatics.psb.ugent.be/webtools/plantcare/html/). The yellow boxes show the motifs are light responsive elements.
FIGURE S5 | Prediction of interacting proteins of PHYB and ER in Arabidopsis by STRING (http://string-db.org/).
TABLE S1 | Primers used in this study.
Footnotes
References
Asensi-Fabado, M. A., Oliván, A., and Munné-Bosch, S. (2013). A comparative study of the hormonal response to high temperatures and stress reiteration in three Labiatae species. Environ. Exp. Bot 94(Suppl. C), 57–65. doi: 10.1016/j.envexpbot.2012.05.001
Bailly, A., Sovero, V., Vincenzetti, V., Santelia, D., Bartnik, D., Koenig, B., et al. (2008). Modulation of P-glycoproteins by auxin transport inhibitors is mediated by interaction with immunophilins. J. Biol. Chem. 283, 21817–21826. doi: 10.1074/jbc.M709655200
Ballaré, C. L. (1999). Keeping up with the neighbours: phytochrome sensing and other signalling mechanisms. Trends Plant Sci. 4, 97–102. doi: 10.1016/S1360-1385(99)01383-7
Becraft, P. W. (2002). Receptor kinase signaling in plant development. Annu. Rev. Cell Dev. Biol. 18, 163–192. doi: 10.1146/annurev.cellbio.18.012502.083431
Blair, M., Cortes, A., and This, D. (2016). Identification of an ERECTA gene and its drought adaptation associations with wild and cultivated common bean. Plant Sci. 242, 250–259. doi: 10.1016/j.plantsci.2015.08.004
Carriedo, L. G., Maloof, J. N., and Brady, S. M. (2016). Molecular control of crop shade avoidance. Curr. Opin. Plant Biol. 30, 151–158. doi: 10.1016/j.pbi.2016.03.005
Casal, J. J. (2013). Photoreceptor signaling networks in plant responses to shade. Annu. Rev. Plant Biol. 64, 403–427. doi: 10.1146/annurev-arplant-050312-120221
Chinchilla, D., Zipfel, C., Robatzek, S., Kemmerling, B., Nurnberger, T., and Jones, J. D. G. (2007). A flagellin-induced complex of the receptor FLS2 and BAK1 initiates plant defence. Nature 448, 497–500. doi: 10.1038/nature05999
Cox, D., and Muday, G. (1994). NPA binding activity is peripheral to the plasma membrane and is associated with the cytoskeleton. Plant Cell 6, 1941–1953. doi: 10.1105/tpc.6.12.1941
Dai, X., Mashiguchi, K., Chen, Q., Kasahara, H., Kamiya, Y., Ojha, S., et al. (2013). The biochemical mechanism of auxin biosynthesis by an Arabidopsis YUCCA Flavin-containing Monooxygenase. J. Biol. Chem. 288, 1448–1457. doi: 10.1074/jbc.M112.424077
Du, J., Yin, H., Zhang, S., Wei, Z., Zhao, B., Zhang, J., et al. (2012). Somatic embryogenesis receptor kinases control root development mainly via brassinosteroid-independent actions in Arabidopsis thaliana. J. Integr. Plant Biol. 54, 388–399. doi: 10.1111/j.1744-7909.2012.01124.x
Durbak, A. R., and Tax, F. E. (2011). CLAVATA signaling pathway receptors of Arabidopsis regulate cell proliferation in fruit organ formation as well as in meristems. Genetics 189, 177–194. doi: 10.1534/genetics.111.130930
Galstyan, A., Cifuentes-Esquivel, N., Bou-Torrent, J., and Martinez-Garcia, J. F. (2011). The shade avoidance syndrome in Arabidopsis: a fundamental role for atypical basic helix-loop-helix proteins as transcriptional cofactors. Plant J. 66, 258–267. doi: 10.1111/j.1365-313X.2011.04485.x
Gommers, C. M. M., Keuskamp, D. H., Buti, S., van Veen, H., Koevoets, I. T., Reinen, E., et al. (2017). Molecular profiles of contrasting shade response strategies in wild plants: differential control of immunity and shoot elongation. Plant Cell 29, 331–344. doi: 10.1105/tpc.16.00790
Gou, X., Yin, H., He, K., Du, J., Yi, J., Xu, S., et al. (2012). Genetic evidence for an indispensable role of somatic embryogenesis receptor kinases in brassinosteroid signaling. PLOS Genet. 8:e1002452. doi: 10.1371/journal.pgen.1002452
Gray, W. M., Östin, A., Sandberg, G., Romano, C. P., and Estelle, M. (1998). High temperature promotes auxin-mediated hypocotyl elongation in Arabidopsis. Proc. Natl. Acad. Sci. U.S.A. 95, 7197–7202. doi: 10.1073/pnas.95.12.7197
Guo, Z., Fujioka, S., Blancaflor, E. B., Miao, S., Gou, X., and Li, J. (2010). TCP1 modulates brassinosteroid biosynthesis by regulating the expression of the key biosynthetic gene DWARF4 in Arabidopsis thaliana. Plant Cell 22, 1161–1173. doi: 10.1105/tpc.109.069203
Hanzawa, T., Shibasaki, K., Numata, T., Kawamura, Y., Gaude, T., and Rahman, A. (2013). Cellular auxin homeostasis under high temperature is regulated through a sorting NEXIN1-dependent endosomal trafficking pathway. Plant Cell 25, 3424–3433. doi: 10.1105/tpc.113.115881
He, K., Gou, X., Yuan, T., Lin, H., Asami, T., Yoshida, S., et al. (2007). BAK1 and BKK1 regulate brassinosteroid-dependent growth and brassinosteroid-independent cell-death pathways. Curr. Biol. 17, 1109–1115. doi: 10.1016/j.cub.2007.05.036
He, W., Brumos, J., Li, H., Ji, Y., Ke, M., Gong, X., et al. (2011). A small-molecule screen identifies L-kynurenine as a competitive inhibitor of TAA1/TAR activity in ethylene-directed auxin biosynthesis and root growth in Arabidopsis. Plant Cell 23, 3944–3960. doi: 10.1105/tpc.111.089029
Hössel, D., Schmeiser, C., and Hertel, R. (2005). Specificity patterns indicate that auxin exporters and receptors are the same proteins. Plant Biol. 7, 41–48. doi: 10.1055/s-2004-830475
Jensen, P. J., Hangarter, R. P., and Estelle, M. (1998). Auxin Transport is required for hypocotyl elongation in light-grown but not dark-grown Arabidopsis. Plant Physiol. 116, 455–462. doi: 10.1104/pp.116.2.455
Kasulin, L., Agrofoglio, Y., and Botto, J. F. (2013). The receptor-like kinase ERECTA contributes to the shade-avoidance syndrome in a background-dependent manner. Ann. Bot. 111, 811–819. doi: 10.1093/aob/mct038
Keuskamp, D. H., Pollmann, S., Voesenek, L. A. C. J., Peeters, A. J. M., and Pierik, R. (2010). Auxin transport through PIN-FORMED 3 (PIN3) controls shade avoidance and fitness during competition. Proc. Natl. Acad. Sci. U.S.A. 107, 22740–22744. doi: 10.1073/pnas.1013457108
Kim, K., and Portis, J. A. R. (2005). Temperature dependence of photosynthesis in Arabidopsis plants with modifications in rubisco activase and membrane fluidity. Plant Cell Physiol. 46, 522–530. doi: 10.1093/pcp/pci052
Korasick, D. A., Enders, T. A., and Strader, L. C. (2013). Auxin biosynthesis and storage forms. J. Exp. Bot. 64, 2541–2555. doi: 10.1093/jxb/ert080
Li, J. (2010). Multi-tasking of somatic embryogenesis receptor-like protein kinases. Curr. Opin. Plant Biol. 13, 509–514. doi: 10.1016/j.pbi.2010.09.004
Li, J., and Chory, J. (1997). A putative leucine-rich repeat receptor kinase involved in brassinosteroid signal transduction. Cell 90, 929–938. doi: 10.1016/S0092-8674(00)80357-8
Li, J., Wen, J., Lease, K. A., Doke, J. T., Tax, F. E., and Walker, J. C. (2002). BAK1, an Arabidopsis LRR receptor-like protein kinase, interacts with BRI1 and modulates brassinosteroid signaling. Cell 110, 213–222. doi: 10.1016/S0092-8674(02)00812-7
Lorrain, S., Allen, T., Duek, P. D., Whitelam, G. C., and Fankhauser, C. (2008). Phytochrome-mediated inhibition of shade avoidance involves degradation of growth-promoting BHLH transcription factors. Plant J. 53, 312–323. doi: 10.1111/j.1365-313X.2007.03341.x
Ludwig-Müller, J. (2011). Auxin conjugates: their role for plant development and in the evolution of land plants. J. Exp. Bot. 62, 1757–1773. doi: 10.1093/jxb/erq412
Masle, J., Gilmore, S. R., and Farquhar, G. D. (2005). The ERECTA gene regulates plant transpiration efficiency in Arabidopsis. Nature 436, 866–870. doi: 10.1038/nature03835
Meng, L., Buchanan, B. B., Feldman, L. J., and Luan, S. (2012). CLE-like (CLEL) peptides control the pattern of root growth and lateral root development in Arabidopsis. Proc. Natl. Acad. Sci. U.S.A. 109, 1760–1765. doi: 10.1073/pnas.1119864109
Müller-Moulé, P., Nozue, K., Pytlak, M. L., Palmer, C. M., Covington, M. F., Wallace, A. D., et al. (2016). YUCCA auxin biosynthetic genes are required for Arabidopsis shade avoidance. PeerJ 4:e2574. doi: 10.7717/peerj.2574
Nam, K. H., and Li, J. (2002). BRI1/BAK1, a receptor kinase pair mediating brassinosteroid signaling. Cell 110, 203–212. doi: 10.1016/S0092-8674(02)00814-0
Nishimura, T., Hayashi, K. I., Suzuki, H., Gyohda, A., Takaoka, C., Sakaguchi, Y., et al. (2014). Yucasin is a potent inhibitor of YUCCA, a key enzyme in auxin biosynthesis. Plant J. 77, 352–366. doi: 10.1111/tpj.12399
Normanly, J., Cohen, J. D., and Fink, G. R. (1993). Arabidopsis thaliana auxotrophs reveal a tryptophan-independent biosynthetic pathway for indole-3-acetic acid. Proc. Natl. Acad. Sci. U.S.A. 90, 10355–10359. doi: 10.1073/pnas.90.21.10355
Oh, M.-H., Wang, X., Kota, U., Goshe, M. B., Clouse, S. D., and Huber, S. C. (2009). Tyrosine phosphorylation of the BRI1 receptor kinase emerges as a component of brassinosteroid signaling in Arabidopsis. Proc. Natl. Acad. Sci. U.S.A. 106, 658–663. doi: 10.1073/pnas.0810249106
Oh, M.-H., Wang, X., Wu, X., Zhao, Y., Clouse, S. D., and Huber, S. C. (2010). Autophosphorylation of Tyr-610 in the receptor kinase BAK1 plays a role in brassinosteroid signaling and basal defense gene expression. Proc. Natl. Acad. Sci. U.S.A. 107, 17827–17832. doi: 10.1073/pnas.0915064107
Patel, D., Basu, M., Hayes, S., Majláth, I., Hetherington, F. M., Tschaplinski, T. J., et al. (2013). Temperature-dependent shade avoidance involves the receptor-like kinase ERECTA. Plant J. 73, 980–992. doi: 10.1111/tpj.12088
Pierik, R., Djakovic-Petrovic, T., Keuskamp, D. H., de Wit, M., and Voesenek, L. A. C. J. (2009). Auxin and ethylene regulate elongation responses to neighbor proximity signals independent of gibberellin and DELLA proteins in Arabidopsis. Plant Physiol. 149, 1701–1712. doi: 10.1104/pp.108.133496
Procko, C., Crenshaw, C. M., Ljung, K., Noel, J. P., and Chory, J. (2014). Cotyledon-generated auxin is required for shade-induced hypocotyl growth in Brassica rapa. Plant Physiol. 165, 1285–1301. doi: 10.1104/pp.114.241844
Qu, X., Zhao, Z., and Tian, Z. (2017). ERECTA regulates cell elongation by activating auxin biosynthesis in Arabidopsis thaliana. Front. Plant Sci. 8:1688. doi: 10.3389/fpls.2017.01688
Rédei, G. P., Koncz, C., Chua, N. H., and Schell, J. (1992). A Heuristic Glance at the Past of Arabidopsis Genetics. Singapore: World Scientific. doi: 10.1142/9789814439701_0001
Roux, M., Schwessinger, B., Albrecht, C., Chinchilla, D., Jones, A., Holton, N., et al. (2011). The Arabidopsis leucine-rich repeat receptor–like kinases BAK1/SERK3 and BKK1/SERK4 are required for innate immunity to hemibiotrophic and biotrophic pathogens. Plant Cell 23, 2440–2455. doi: 10.1105/tpc.111.084301
Ruberti, I., Sessa, G., Ciolfi, A., Possenti, M., Carabelli, M., and Morelli, G. (2012). Plant adaptation to dynamically changing environment: the shade avoidance response. Biotechnol. Adv. 30, 1047–1058. doi: 10.1016/j.biotechadv.2011.08.014
Rubery, P. (1979). The effects of 2,4-dinitrophenol and chemical modifying reagents on auxin transport by suspension-cultured crown gall cells. Planta 144, 173–178. doi: 10.1007/BF00387267
Shen, H., Zhong, X., Zhao, F., Wang, Y., Yan, B., Li, Q., et al. (2015). Overexpression of receptor-like kinase ERECTA improves thermotolerance in rice and tomato. Nat. Biotechnol. 33, 996–1003. doi: 10.1038/nbt.3321
Shiu, S. H., and Bleecker, A. B. (2001). Plant receptor-like kinase gene family: diversity, function, and signaling. Sci. STKE 2001:re22. doi: 10.1126/stke.2001.113.re22
Shiu, S. H., Karlowski, W. M., Pan, R., Tzeng, Y. H., Mayer, K. F., and Li, W. H. (2004). Comparative analysis of the receptor-like kinase family in Arabidopsis and rice. Plant Cell 16, 1220–1234. doi: 10.1105/tpc.020834
Shiu, S. H., and Li, W. H. (2004). Origins, lineage-specific expansions, and multiple losses of tyrosine kinases in eukaryotes. Mol. Biol. Evol. 21, 828–840. doi: 10.1093/molbev/msh077
Shpak, E. D. (2013). Diverse roles of ERECTA family genes in plant development. J. Integr. Plant Biol. 55, 1238–1250. doi: 10.1111/jipb.12108
Shpak, E. D., Berthiaume, C. T., Hill, E. J., and Torii, K. U. (2004). Synergistic interaction of three ERECTA-family receptor-like kinases controls Arabidopsis organ growth and flower development by promoting cell proliferation. Development 131, 1491–1501. doi: 10.1242/dev.01028
Shpak, E. D., McAbee, J. M., Pillitteri, L. J., and Torii, K. U. (2005). Stomatal patterning and differentiation by synergistic interactions of receptor kinases. Science 309, 290–293. doi: 10.1126/science.1109710
Shpak, E. D., Lakeman, M. B., and Torii, K. U. (2003). Dominant-negative receptor uncovers redundancy in the arabidopsis ERECTA leucine-rich repeat receptor-like kinase signaling pathway that regulates organ shape. Plant Cell 15, 1095–1110. doi: 10.1105/tpc.010413
Sieberer, T., Seifert, G., Hauser, M., Grisafi, P., Fink, G., and Luschnig, C. (2000). Post-transcriptional control of the Arabidopsis auxin efflux carrier EIR1 requires AXR1. Curr. Biol. 10, 1595–1598. doi: 10.1016/S0960-9822(00)00861-7
Stegmann, M., Monaghan, J., Smakowska-Luzan, E., Rovenich, H., Lehner, A., Holton, N., et al. (2017). The receptor kinase FER is a RALF-regulated scaffold controlling plant immune signaling. Science 355, 287–289. doi: 10.1126/science.aal2541
Sussman, M., and Goldsmith, M. (1981). The action of specific inhibitors of auxin transport on uptake of auxin and binding of N-1-naphthylphthalamic acid to a membrane site in maize coleoptiles. Planta 152, 13–18. doi: 10.1007/BF00384978
Tao, Y., Ferrer, J.-L., Ljung, K., Pojer, F., Hong, F., Long, J. A., et al. (2008). Rapid synthesis of auxin via a new tryptophan-dependent pathway is required for shade avoidance in plants. Cell 133, 164–176. doi: 10.1016/j.cell.2008.01.049
Teale, W., and Palme, K. (2017). Naphthylphthalamic acid and the mechanism of polar auxin transport. J. Exp. Bot. 69, 303–312. doi: 10.1093/jxb/erx323
Tivendale, N. D., Ross, J. J., and Cohen, J. D. (2014). The shifting paradigms of auxin biosynthesis. Trends Plant Sci. 19, 44–51. doi: 10.1016/j.tplants.2013.09.012
Torii, K. U., Mitsukawa, N., Oosumi, T., Matsuura, Y., Yokoyama, R., and Whittier, R. F. (1996). The Arabidopsis ERECTA gene encodes a putative receptor protein kinase with extracellular leucine-rich repeats. Plant Cell 8, 735–746. doi: 10.1105/tpc.8.4.735
Valladares, F., and Niinemets, Ü (2008). Shade tolerance, a key plant feature of complex nature and consequences. Annu. Rev. Ecol. Evol. Syst. 39, 237–257. doi: 10.1146/annurev.ecolsys.39.110707.173506
van Zanten, M., Snoek, L. B., Proveniers, M. C., and Peeters, A. J. (2009). The many functions of ERECTA. Trends Plant Sci. 14, 214–218. doi: 10.1016/j.tplants.2009.01.010
van Zanten, M., Snoek, L. B., van Eck-Stouten, E., Proveniers, M. C. G., Torii, K. U., Voesenek, L. A. C. J., et al. (2010). ERECTA controls low light intensity-induced differential petiole growth independent of Phytochrome B and Cryptochrome 2 action in Arabidopsis thaliana. Plant Signal. Behav. 5, 284–286. doi: 10.4161/psb.5.3.10706
Vanneste, S., and Friml, J. (2009). Auxin: a trigger for change in plant development. Cell 136, 1005–1016. doi: 10.1016/j.cell.2009.03.001
Vieten, A., Vanneste, S., Wisniewska, J., Benková, E., Benjamins, R., Beeckman, T., et al. (2005). Functional redundancy of PIN proteins is accompanied by auxin-dependent cross-regulation of PIN expression. Development 132, 4521–4531. doi: 10.1242/dev.02027
Villagarcia, H., Morin, A.-C., Shpak, E., and Khodakovskaya, M. V. (2012). Modification of tomato growth by expression of truncated ERECTA protein from Arabidopsis thaliana. J. Exp. Bot. 63, 6493–6504. doi: 10.1093/jxb/ers305
Walker, J. C., and Zhang, R. (1990). Relationship of a putative receptor protein kinase from maize to the S-locus glycoproteins of Brassica. Nature 345, 743–746. doi: 10.1038/345743a0
Wang, B., Chu, J., Yu, T., Xu, Q., Sun, X., Yuan, J., et al. (2015). Tryptophan-independent auxin biosynthesis contributes to early embryogenesis in Arabidopsis. Proc. Natl. Acad. Sci. U.S.A. 112, 4821–4826. doi: 10.1073/pnas.1503998112
Wit, M. D., Galvão, V. C., and Fankhauser, C. (2016). Light-mediated hormonal regulation of plant growth and development. Annu. Rev. Plant Biol. 67, 513–537. doi: 10.1146/annurev-arplant-043015-112252
Woodward, A. W., and Bartel, B. (2005). Auxin: regulation. Action Interact. Ann. Bot. 95, 707–735. doi: 10.1093/aob/mci083
Wright, A. D., Sampson, M. B., Neuffer, M. G., Michalczuk, L., Slovin, J. P., and Cohen, J. D. (1991). Indole-3-acetic acid biosynthesis in the mutant maize orange pericarp, a tryptophan auxotroph. Science 254, 998–1000. doi: 10.1126/science.254.5034.998
Wu, G., Carville, J. S., and Spalding, E. P. (2016). ABCB19-mediated polar auxin transport modulates Arabidopsis hypocotyl elongation and the endoreplication variant of the cell cycle. Plant J. 85, 209–218. doi: 10.1111/tpj.13095
Yang, C., and Li, L. (2017). Hormonal regulation in shade avoidance. Front. Plant Sci. 8:1527. doi: 10.3389/fpls.2017.01527
Zhao, Y. (2010). Auxin biosynthesis and its role in plant development. Annu. Rev. Plant Biol. 61, 49–64. doi: 10.1146/annurev-arplant-042809-112308
Zhao, Y. (2012). Auxin biosynthesis: a simple two-step pathway converts tryptophan to indole-3-acetic acid in plants. Mol. Plant 5, 334–338. doi: 10.1093/mp/ssr104
Zhao, Y., Christensen, S. K., Fankhauser, C., Cashman, J. R., Cohen, J. D., Weigel, D., et al. (2001). A role for flavin monooxygenase-like enzymes in auxin biosynthesis. Science 291, 306–309. doi: 10.1126/science.291.5502.306
Zheng, Z., Guo, Y., Novák, O., Chen, W., Ljung, K., Noel, J. P., et al. (2016). Local auxin metabolism regulates environment-induced hypocotyl elongation. Nat. Plants 2:16025. doi: 10.1038/nplants.2016.25
Keywords: ERECTA, receptor-like kinase, shade avoidance, auxin, GA
Citation: Du J, Jiang H, Sun X, Li Y, Liu Y, Sun M, Fan Z, Cao Q, Feng L, Shang J, Shu K, Liu J, Yang F, Liu W, Yong T, Wang X, Yuan S, Yu L, Liu C and Yang W (2018) Auxin and Gibberellins Are Required for the Receptor-Like Kinase ERECTA Regulated Hypocotyl Elongation in Shade Avoidance in Arabidopsis. Front. Plant Sci. 9:124. doi: 10.3389/fpls.2018.00124
Received: 30 June 2017; Accepted: 23 January 2018;
Published: 07 February 2018.
Edited by:
Kai He, Lanzhou University, ChinaReviewed by:
Joshua Blakeslee, The Ohio State University, United StatesYingfang Zhu, Purdue University, United States
Copyright © 2018 Du, Jiang, Sun, Li, Liu, Sun, Fan, Cao, Feng, Shang, Shu, Liu, Yang, Liu, Yong, Wang, Yuan, Yu, Liu and Yang. This is an open-access article distributed under the terms of the Creative Commons Attribution License (CC BY). The use, distribution or reproduction in other forums is permitted, provided the original author(s) and the copyright owner are credited and that the original publication in this journal is cited, in accordance with accepted academic practice. No use, distribution or reproduction is permitted which does not comply with these terms.
*Correspondence: Junbo Du, junbodu@hotmail.com Wenyu Yang, mssiyangwy@sicau.edu.cn
† These authors have contributed equally to this work.