- 1DISTAL – Department of Agricultural and Food Sciences, Alma Mater Studiorum – Università di Bologna, Bologna, Italy
- 2María de Maeztu Unit of Excellence, Institut de Ciència i Tecnologia Ambientals (ICTA-UAB), Universitat Autònoma de Barcelona, Barcelona, Spain
- 3Chemical, Biological and Environmental Engineering Department, Universitat Autònoma de Barcelona, Barcelona, Spain
Intensive growing systems used for greenhouse tomato production, together with light interception by cladding materials or other devices, may induce intracanopy mutual shading and create suboptimal environmental conditions for plant growth. There are a large number of published peer-reviewed studies assessing the effects of supplemental light-emitting diode (LED) lighting on improving light distribution in plant canopies, increasing crop yields and producing qualitative traits. However, the research results are often contradictory, as the lighting parameters (e.g., photoperiod, intensity, and quality) and environmental conditions vary among conducted experiments. This research presents a global overview of supplemental LED lighting applications for greenhouse tomato production deepened by a meta-analysis aimed at answering the following research question: does supplemental LED lighting enhance the yield and qualitative traits of greenhouse truss tomato production? The meta-analysis was based on the differences among independent groups by comparing a control value (featuring either background solar light or solar + HPS light) with a treatment value (solar + supplemental LED light or solar + HPS + supplemental LED light, respectively) and included 31 published papers and 100 total observations. The meta-analysis results revealed the statistically significant positive effects (p-value < 0.001) of supplemental LED lighting on enhancing the yield (+40%), soluble solid (+6%) and ascorbic acid (+11%) contents, leaf chlorophyll content (+31%), photosynthetic capacity (+50%), and leaf area (+9%) compared to the control conditions. In contrast, supplemental LED lighting did not show a statistically significant effect on the leaf stomatal conductance (p-value = 0.171). In conclusion, in addition to some partial inconsistencies among the considered studies, the present research enables us to assert that supplemental LED lighting ameliorates the quantitative and qualitative aspects of greenhouse tomato production.
Introduction
In greenhouse tomato (Solanum lycopersicum) production, photosynthesis and carbon sequestration may be hindered by cloud cover, shading systems, and variable solar radiation, as well as by plant mutual shading (e.g., when high vertical stem training or increased crop densities are used) (Zhang et al., 2015; Tewolde et al., 2018). Considering the non-uniform distribution of solar radiation around the world, limitations may also occur in cases of high-latitude countries such as Canada, Japan, Norway, as well as in the northern areas of China and the United States, where long winters and low DLIs (daily light integrals) may affect greenhouse production (Garland et al., 2010; Deram et al., 2014; Sun et al., 2015; Tewolde et al., 2016; Paponov et al., 2019). Supplemental artificial lighting can be applied to increase greenhouse yields and ensure stable year-round production regardless of environmental conditions (Ohashi-Kaneko et al., 2007), even in regions with high DLIs, such as the Mediterranean and Jordan Valley (Israel) (Joshi et al., 2019; Paucek et al., 2020). Today, light-emitting diode (LED) lamps represent the most advantageous artificial lighting systems in terms of energy use efficiency, with foreseen expectations for further reducing investments and running costs in the near future (Olle and Viršile, 2013). Additional advantages also involve the functional aspects of LEDs that make the technology suitable for cultivation, particularly thanks to their possible miniaturization, light weight, and limited radiant heat emissions (Ibaraki, 2017). Accordingly, LED lamps can be used in proximity to plant canopies without excessively increasing the leaf temperature (Morrow, 2008), enabling inter-lighting applications that reduce intracanopy shading conditions in high-stem-density plants (Jokinen et al., 2012, Gómez and Mitchell, 2016a; Kumar et al., 2016; Hao et al., 2017).
LED application can enable the fine tuning of combinations between light spectral compositions and light intensities, with direct consequences not only on yield but also on structural and physiological plant aspects (Ouzounis et al., 2015; Hao et al., 2017; Ibaraki, 2017). In fact, the responses of plants to light characteristics are regulated by photoreceptors that reading specific wavelengths, intensities or photoperiods can trigger signals that modify plant metabolism (Christie et al., 2015). Accordingly, light environmental management can lead to interesting commercial results. For instance, red light can promote flower development (Liao et al., 2014), while the blue-violet spectrum can increase plant protection from diseases (Tokuno et al., 2012; Hui et al., 2017), preserving postharvest conservation and food safety through the inactivation of pathogen action (D'Souza et al., 2015). Moreover, specific light spectra can improve the qualitative and nutraceutical aspects of plants (Mempel and Wittmann, 2019), such as enhancing antioxidant compound biosynthesis (e.g., flavonoids, ascorbic acid) in various species (e.g., lettuce, basil, tomato) (Ebisawa et al., 2008; Carvalho et al., 2016; Jiang et al., 2017; Pennisi et al., 2019a,b).
Stomatal conductance is a specific physiological response that is guided by light. The wavelength mainly involved in this process is blue light (450 and 495 n), which is also implicated in other mechanisms, such as phototropism, chloroplast migration, photomorphogenesis, and chlorophyll production (O'Carrigan et al., 2014b). Cryptochromes and phototropins are the main photoreceptors stimulated by blue light (Christie et al., 2015); these photoreceptors go through a phosphorylation process and bind protein to trigger proton extrusion and K+ uptake in stomatal guard cells, with the consequent cell turgidity and stomatal opening enabling gas exchange (Roelfsema and Hedrich, 2005: Shimazaki et al., 2007). Apparently, green and red light may also play roles in gas exchange by inducing stomatal closure, as green light may stop soluble uptake in guard cells (Talbott et al., 2002), while red light may lead to K+ and solute losses (Zeiger, 1990). In tomato plants, studies that have applied blue, red, and green lighting in closed chambers seem to confirm such observations (O'Carrigan et al., 2014b; Bian et al., 2019), opening the possibility of integrating green LED lighting to reduce drought stress in tomato plants (Bian et al., 2019). However, it is important to consider that what is observed in growing chamber experiments is not always transferable to the processes occurring in productive systems, where different environmental factors may affect plant responses.
Greenhouse tomatoes represent one of the most relevant horticultural crops worldwide (Deram et al., 2014; FAO, 2018). In intensive greenhouse tomato production, high-wire single-truss training systems are normally applied to enable labor reductions, multiple harvests and possible automation (Giniger et al., 1988; Okano et al., 2001). Nonetheless, the high plant density required for these systems can limit light penetration within canopies with consequences on fruit quality and yield (Wada et al., 2006). In this context, several studies have already reported the usefulness of LED lighting system applications for qualitative and quantitative improvements in greenhouse truss tomato production (Tewolde et al., 2016; Dzakovich et al., 2017; Jiang et al., 2017; Kim et al., 2019). However, inconsistencies among studies are also present; non-significant effects of supplemental LED lighting, especially on qualitative parameters (e.g., soluble solids, ascorbic acid) (Lu et al., 2012b; Hao et al., 2016), have been found. In most studies to date, researchers have integrated supplemental LED lighting technologies either in greenhouses where no supplemental lighting was formerly present or as additional lighting sources in greenhouses where top artificial lights (e.g., high-pressure sodium lights, HPSs, lamps) were already installed and in operation. Accordingly, this study aims to offer an overview of the recent topic of supplemental LED lighting for greenhouse tomato production through the use of a meta-analysis as a statistical tool to summarize the results of published studies and understand the effectiveness of supplementary LED lighting in influencing the qualitative-quantitative aspects of truss tomatoes. Consequently, the meta-analysis aims to answer the following research question: does supplemental LED lighting enhance the yield and qualitative traits of greenhouse truss tomato production?
Materials and Methods
Data Collection
Article collection was conducted during the first half of 2020 using online databases (e.g., Google Scholar and Scopus). The following search string was applied to identify publications: LED AND supplemental light* AND tomato* AND greenhouse. Only accessible published material in the English language was collected, including scientific articles, conference papers, book chapters, and thesis dissertations. The literature search results were then filtered to reduce heterogeneity in the studies and to include only Solanum lycopersicum species cultivated in greenhouses with supplemental LED lighting or supplemental LED lighting combined with HPS lamps. All cultivar types, growing systems and greenhouse typologies were considered. Given that the presence of solar radiation was a requisite of the research question (targeting the effect of supplemental LEDs in greenhouses), studies of indoor cultivation in which only artificial lighting sources (e.g., indoor farming) were applied were not considered in the research. Overhead, intracanopy and bottom lighting supplies were all included, as well as nighttime, end-of-the-day, and continuous lighting treatments. Only one case of night-break lighting supply was excluded from the research (Cao et al., 2016). Furthermore, studies reporting evaluations on seedlings or transplants with short treatment periods were also excluded; only mature and productive plants were considered to accomplish the upstream objective of evaluating the qualitative and quantitative effects of supplemental LED lighting on tomato production.
The collected data included both general information related to trial conditions and more specific data used in the meta-analysis. In particular, the general data were represented by intrinsic or environmental trial features (cultivar, location, maximum and minimum temperatures, relative humidity, nutrient solution electrical conductivity (EC) and pH, plant density, greenhouse typology, and growing system), as well as by the LED treatment characteristics (light spectrum, intensity and photoperiod, treatment duration, and other eventual specific experimental conditions, e.g., nighttime treatments). All these general data were used in the descriptive statistical analysis and to identify factors of heterogeneity among different experiments during the meta-analysis. The natural lighting amount (e.g., DLI) was not considered due to the scarcity of articles reporting this information. The specific data referred to precise information that was needed for the development of the meta-analysis, including the treatment and control mean outcomes as well as the sample size (or replicate number, in cases in which the sample size was not available). Studies not reporting specific data were not used for the meta-analysis development. The outcomes, also called the effect sizes or response ratios [R] (Hedges et al., 1999), used in the meta-analysis consisted of the fresh fruit mass yield (yield, expressed as kg plant−1 of fresh fruit mass), soluble solid content (TSS, expressed as °Brix), ascorbic acid content (Asc, expressed as mg Asc 100 g−1 of fruit fresh weight), chlorophyll content (Chl, expressed as Chl index), net photosynthesis (PN, expressed as μmol CO2 m−2 s−1), stomatal conductance (gs, expressed as mmol H2O m−2 s−1), and leaf area (LA, expressed as m2 per plant). Only physiological and vegetative outcomes directly influencing tomato productivity and quality were considered, while other information (e.g., stem diameter, internode length) was not investigated. Outcome values were extracted from both tables and graphs, integrating textual information in cases of general descriptive data relative to the trials. Figure 1 shows the flow diagram applied for the data selection and evaluation.
Meta-Analysis
The response ratio [R] considered in the meta-analysis was represented by the influence of supplemental LED lighting on the Yield, TSS, Asc, Chl, PN, gs, and LA of greenhouse-grown tomato plants. Since each study accounted for more than one treatment, each trial was examined as a separate observation (k). Accordingly, each study could have more than one observation. For instance, if an article compared two different supplemental LED lighting treatments, one with a red spectrum and the other with a blue spectrum, each treatment was considered an individual observation. Each treatment value was compared with a control value from the same article to perform a meta-analysis based on the differences among independent groups. In the current study, the applied control treatment may be of two types: solar light only or solar light + HPS light. In the first case, the control was compared with solar light + supplemental LED light, while in the second case, the comparison involved solar light + HPS light + supplemental LED light. Only observations reporting a control, either with solar or solar + HPS light, were used for the meta-analysis after a second selection phase. In one case (Deram et al., 2014), the comparison between the control and treatment showed extremely high values compared to other results. In this case, the data were considered outliers and were excluded from the meta-analysis.
The [R] of each observation was calculated as follows:
where mt and mc represent the mean outcomes of the treatment and control, respectively (Hedges et al., 1999; Borenstein et al., 2009). Since most of the considered publications did not display standard error (SE), variance (Var), or standard deviation (S) values, an unweighted meta-analysis was applied to equally weight each observation (McDaniel et al., 2014; Qin et al., 2015). The data were analyzed using the online available software Meta-Essential (Suurmond et al., 2017). A random effect model was chosen for each response value (Yield, TSS, Asc, Chl, PN, gs, and LA). The heterogeneity value (I2) was used to evaluate the percentage of variation among studies (Hak et al., 2016). Cases reporting I2 values higher than 25% were further investigated by applying a subgroup analysis (Borenstein et al., 2009; Hak et al., 2016). The subgroup analysis divided the observations into six categories: solar light or solar light + HPS used as a control; pure supplemental LED light or supplemental LED light + HPS; artificial light supply (e.g., DLI ≥10 or <10 mol m−2 d−1); seasonality (whether the hours of natural light were increasing, e.g., during spring in the Northern Hemisphere, or decreasing, e.g., during fall in Northern Hemisphere, along the experiment); photoperiod ≥16 or <16 h d−1; lighting supplied as intracanopy or others. In the last case, “others” were intended to include overhead, bottom or combined lighting supplies, which were grouped together due to the low number of singular categories. Hedges' g was applied as the measurement of the effect size in the meta-analysis model. The [R] value was accepted as significant with a p-value < 0.05, considering a confidence interval (CI) of 95%. Since the results showed high heterogeneity, no publication bias analysis was performed, assuming its absence (Hak et al., 2016). A graphic representation of the study distribution per year and country was realized using Gephi software (Bastian et al., 2009) (Figure 2).
Results
The literature search results are included in Supplementary Material 1, attached as an Excel file to the present manuscript. The preliminary literature search resulted in 45 studies following the selection criteria. These publications were used for the descriptive statistical analysis. The results showed that the majority of trials took place in North America, with 38% of the total cases (USA n = 9, Canada n = 8), while Europe (Netherlands n = 8, Norway n = 3, Poland n = 2, Belgium n = 1, Germany n = 1, and Italy n = 1) and Asia (Japan n = 6, China n = 4, and South Korea n = 2) reported frequencies of 35 and 27%, respectively. No cases were registered in other continents. No collected publication was released before 2011, and the collected studies showed the highest frequencies in 2019 (29%) and 2016 (18%) (Figure 2).
Although not always stated, most experiments were conducted in technologically advanced greenhouses applying soilless growing methods, and the studies often mentioned controlled environmental systems. When reported, the highest-frequency growing methods reported were substrate cultivation on slabs (61% of 36 cases reported this growing method). Slab materials were mainly represented by rockwool, although two cases of coir use were also reported. Pot employment was also registered, occurring for 22% of cited cases with sand, perlite, vermiculite or peat applied as growing substrates. The use of bags filled with substrate (peat, vermiculite or perlite) was also identified in 3 out of 36 cases. Finally, only two soil-based cultivation cases and one nutrient film technique (NFT) case were reported.
Concerning the planting density, the 45 studies showed a mean of ~5 plants m−2, a mode of 2.7 plants m−2 and a median of 2.7 plants m−2, with values ranging from 1.5 to 16.6 plants m−2. The average maximum and minimum temperatures were 23 and 19°C, respectively, while the average relative humidity was 69%. The applied nutrient solutions had a mean EC value of 2.4 dS m−1 and a mean pH value of 6. Different cultivars of truss tomato were used in the trials (33), and the highest recurrence was observed for Solanum lycopersicum cv Komeett (De Ruiter, Amstelveen, The Netherlands), which was mentioned in 10 publications.
In total, 161 supplemental LED lighting treatments were observed within the 45 collected publications. Of those treatments, 57% applied intracanopy LED lighting, 8% applied bottom lighting, 17% applied overhead lamps, 13% applied a combination of supply methods (e.g., intracanopy + overhead lighting), and 6% of cases did not clearly report the type of lighting supply. Furthermore, 20% of revised observations applied a combination of LED and HPS lighting as the supplemental treatment. Regarding the daily lighting duration, the mean photoperiod used was 15 h d−1, while the mode and median durations were both 16 h d−1. Within the collected literature, two extreme cases of 24 h of continuous lighting and 2 h of end-of-the-day lighting were also found. The average photosynthetic photon flux density (PPFD) and DLI supplied through lighting were also registered, showing values of 165 μmol m−2 s−1 and 9.5 mol m−2 d−1, respectively, while the mode and median were 160 and 165 μmol m−2 s−1 for PPFD and 11.5 and 9.8 mol m−2 d−1 for DLI, respectively. Spectral compositions occurred in numerous combinations and ratios depending on the trial. The absolute frequencies of the red, blue, white, far-red, and UV spectral components were registered separately, and each component was counted each time it appeared in a treatment independently from the combinations. The count resulted in red light supplies recurring in 128 cases, while light in the blue, white and far-red spectra were adopted 115, 24 and 20 times, respectively. UV application was only used 3 times, while green light occurred once. Both UV and green light were always applied in combination with other light spectral components. Furthermore, 68% of the reviewed observations used a combination of red and blue diodes, while monochromatic red or blue diodes were applied in 6 and 2% of total observations, respectively. The average duration of treatments was 5 months, with the durations ranging from 2 to 8 months.
After a second selection phase, 10 studies not reporting any control, as well as one outlier case concerning the reported yield values (Deram et al., 2014), were excluded from the meta-analysis. This selection resulted in 31 studies, and 100 total observations were used for the further analyses. The results revealed the generally positive effects of supplemental LED lighting, although different tendencies and significances were observed depending on the evaluated [R] (Figure 3). PN (k = 45) and Yield (k = 68) were the parameters most affected by supplemental LED treatments, reporting the highest standardized mean differences (Hedges' g) of 2.70 and 1.75, respectively. Both response ratios were significantly influenced by supplemental lighting, with one-tailed p-values < 0.001. Conversely, gs (k = 26) showed a standardized mean difference of 0.83, although no significant effect was reported (p = 0.171). Asc (k = 20) and TSS (k = 38) presented standardized mean differences of 0.81 and 0.34, with significant p-values of 0.001 and <0.001, respectively. Finally, Chl (k = 40) and LA (k = 38) recorded similar values, showing Hedges' g values of 0.74 and 0.75, respectively, while the p-values were <0.001 for both cases. Figure 3 displays a summary of the combined effect sizes.
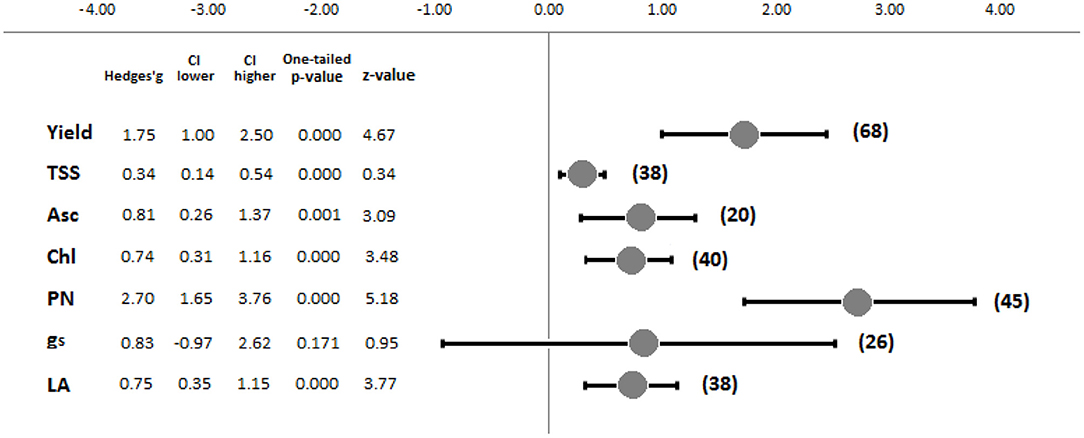
Figure 3. Forest plot showing the combined effect sizes and main meta-analysis parameters of the investigated response ratios (Yield, Yield; TSS, soluble solid content; Asc, ascorbic acid content; Chl, chlorophyll content; PN, photosynthetic capacity; gs, stomatal conductance; LA, leaf area). Numbers within brackets refer to k response ratios. The meta-analysis parameters are the effect size value (Hedges'g), low and high confidence intervals (CI), and tests of the null hypothesis (one-tailed p-value and z-value) (Hak et al., 2016).
The I2 value, which describes the percentage of variation among studies, was the main investigated factor used to understand the heterogeneity in the results (Hak et al., 2016). In particular, fruit yield (Yield) showed a heterogeneity of 89.18%. The qualitative effects, measured as TSS and Asc, reported I2 values of 29.27 and 75.97%, respectively. The physiological parameters showed I2 values equal to 91.66% for gs, 91.15% for PN, and 73.23% for Chl. Finally, the LA heterogeneity was 80.04%. The other parameters explaining heterogeneity are reported in Table 1.
All response ratios showed high heterogeneity, with I2 values >25%. Accordingly, a subgroup analysis was performed for each outcome. Low heterogeneity was observed for Yield in cases of light supplies different from intracanopy supplies (I2 = 18.7%) and cases using solar + HPS lighting as controls (I2 = 12.7%); for TSS in cases with increased natural lighting (I2 = 21.2%) and lighting supplies other than intracanopy supplies (I2 = 0.0%); and for Chl in cases with decreased natural lighting (I2 = 0.0%). Table 2 shows the I2 heterogeneity values identified for each [R] value and subgroup, as well as the percentages of each subgroup both relative to the single response ratios and to the total number of meta-analysis observations. Cases not reporting a sufficient number of observations (k ≥ 5) within each subgroup division were not reported.
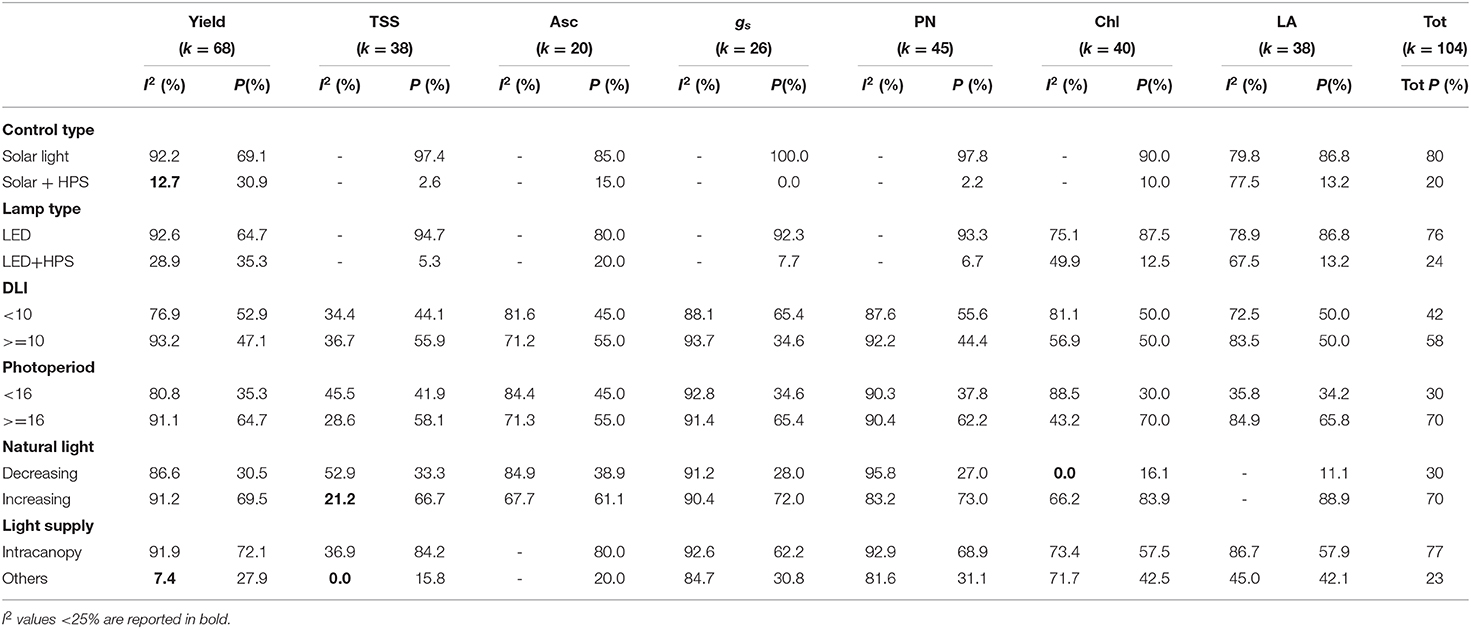
Table 2. Subgroup analysis reporting heterogeneity (I2) and percentage (P) by response ratio (Yield, Yield; TSS, soluble solid content; Asc, ascorbic acid content; Chl, chlorophyll content; PN, photosynthetic capacity; gs, stomatal conductance; LA, leaf area) and total percentage (Tot P) of subgroups considering 104 total observations used in the meta-analysis.
Discussion
The worldwide distribution of the 45 identified studies showed a prevalence of trials in countries of the boreal hemisphere occurring at latitudes above 43°N, falling within the temperate climatic zone (Fischer et al., 2012). Geographical latitude is one of the main factors constraining daily solar radiation during the year, thus affecting the minimal light requirements of most horticultural crops (~2.34 kWh m−2 d−1, which translates to ~8.5 MJ m−2 day−1) and, consequently, affecting climatic suitability for greenhouse cultivation (Castilla and Baeza, 2013). Accordingly, supplemental lighting can be particularly appropriate to guarantee better light distributions and longer cultivation spans in high-tech greenhouses in northern countries, although useful applications were also observed within the Mediterranean area (Paucek et al., 2020). Although Mediterranean greenhouse cultivation is mainly characterized by applications of low-tech solutions (Fernández et al., 2018), some examples of technologically advanced high-density greenhouse farms also exist in this region (Meneses and Castilla, 2009; Tuzel and Oztekin, 2014). In these cases, supplemental lighting may be applied to improve off-season production. Indeed, research on the application of supplemental LED lighting in the Mediterranean region has already demonstrated the capability of this technology to improve yields and anticipate the ripening of truss tomatoes during spring and summer (Paucek et al., 2020), although further research should be conducted in the fall season. Furthermore, it is important to consider that Mediterranean greenhouse cultivation can suffer from excessive sun radiation and temperatures during summertime, making external shading a necessary technique to ensure good internal growing conditions (Castilla et al., 2002). However, sunlight screening may also reduce plant photosynthesis, especially in cases of low-cost permanent solutions (e.g., whitewashing) (Garcia et al., 2011). Tewolde et al. (2018) demonstrated the feasibility of supplemental LED inter-lighting on tomato production in cases of shading cover applications, obtaining the same qualitative-quantitative performances as those observed in the naturally lighted control. Although LED use was identified as an effective artificial lighting source for horticultural purposes (Heuvelink and Gonzalez-Real, 2007; Gupta and Agarwal, 2017), research on greenhouse-grown tomato production using supplemental LED lighting seems to be relatively recent, as evidenced by the higher number of studies published during the last 5 years (Figure 2). Nevertheless, earlier studies on seedlings and transplants are also present (Brazaityte et al., 2009; Suzuki et al., 2009), though they were not considered within this research.
High-tech solutions characterized by the use of soilless cultivation systems, controlled climates and high plant densities were mainly adopted in the evaluated trials. Although not always mentioned, some studies reported high-wire growing methods based on plant lowering, allowing for production throughout several seasons (Kubota et al., 2018). This training system, in association with advanced protected growing technologies, can ensure increased productivity despite flourishing vegetation causing inner canopy shading (Hamamoto and Yamazaki, 2009) and light quality modifications that occur due to both greenhouse cladding materials and shading items (Kittas et al., 1999; Petropoulos et al., 2019). An economic analysis demonstrated that these highly productive systems, together with efficient lighting technologies, can make supplemental lighting more effective for greenhouse tomato production than for the production of other species (Kubota et al., 2016). With reference to both the technical and environmental aspects of trial management, the analysis of the results showed that most supplemental LED lighting studies followed the optimal growth conditions suggested for intensive greenhouse tomato production (Schwarz et al., 2014; Kubota et al., 2018). For instance, rockwool was found to be the most-applied growing substrate, as is commonly observed in greenhouse tomato soilless cultivation systems (Kubota et al., 2018). The environmental growth conditions also followed the recommendations for the fruit production phase, suggesting a mean temperature of 21–18°C, with nutrient solutions featuring ECs of 2.7–4.0 dS m−1 and a pH value of 5.8 (OMAFRA, 2001). When a supplemental LED lighting system is adopted, temperature management becomes a key factor. Dueck et al. (2011) observed that tomato plants grown under LED lighting receive less radiative energy than when other lamp typologies (such as HPS lamps) are used, thus requiring more thermal heat during cold seasons to maintain an optimal temperature within the greenhouse. On the other hand, Verheula et al. (2019) pointed out that the addition of supplemental LED inter-lighting to HPS lamps can increase the temperature by 1–2°C, leading to increased ventilation requirements for greenhouse production during summer. Furthermore, considering that the lifespans of LED lamps are halved when the working temperature increases by 10°C, a cooling system may also be necessary (Nelson and Bugbee, 2014; Hinov et al., 2019). The average planting density value adopted in the considered studies was higher than the suggested greenhouse standards (2.5 plants m−2 for northern Europe) (Kubota et al., 2018), even reaching 16.6 plants m−2 in some studies (Song et al., 2016; Johkan et al., 2017). Elevated planting densities may negatively affect light absorption in tomato plants (Sarlikioti et al., 2011), but the use of supplemental lighting can compensate for the lower light availability caused by an increased planting density, also enabling higher annual production compared to systems with lower planting densities (Dorais et al., 1991).
The lighting distribution is a fundamental factor associated with optimizing the effectiveness of supplemental lighting systems. Traditionally, overhead lamps were used in greenhouse production systems, resulting in increased upper leaf interception and intracanopy shading (Gomez et al., 2013). Although an overhead lighting supply may be preferred by growers due to both its easy installation in greenhouses and reduced labor requirements for crop management (Gunnlaugsson and Adalsteinsson, 2005), intracanopy lighting can increase light interception within a canopy, enhance light use efficiency thanks to better lighting distribution and maintain the photosynthetic capacities of lower leaves (Trouwborst et al., 2011). The efficacy of intracanopy lighting on tomato production has already been ascertained by using HPS and fluorescent lamps (Gunnlaugsson and Adalsteinsson, 2005; Lu et al., 2012a), although its feasibility for technological uptake emerged only after the introduction of low-surface-temperature LEDs (Hao et al., 2012; Guo et al., 2016). In our research, the majority of considered trials applied intracanopy LED lighting alone, sometimes combined with overhead HPS lamps. However, few cases of overhead LED lighting alone against a control were also registered. Although not statistically significant differences could be observed, intracanopy lighting tended to have a larger impact on yield than overhead lighting alone when compared to the controls. Finally, LEDs can also be applied as below-canopy lighting. Supplemental lighting strategies have been shown to increase photosynthesis both below and within the canopy. However, two studies comparing intracanopy light with below-canopy lighting found that the latter technology can promote CO2 assimilation and stomatal conductance by providing stable light penetration even at low canopy levels (Song et al., 2016; Johkan et al., 2017).
Deram et al. (2014) observed that the responses of plants to supplemental lighting also depended on the spectral components of the lighting system adopted. Red and blue wavelengths, alone or combined in different ratios, were mainly used in the studies evaluated in our research. In general, red light was mostly efficient in enhancing photosynthesis (McCree, 1971; Kaiser et al., 2019a), while blue light was shown to play an important role in controlling plant morphology, biomass accumulation and stomatal conductance (Ménard et al., 2005; Johkan et al., 2010; Ieperen et al., 2012). Monochromatic lighting may be less effective than a combination of red and blue light, since combined blue light can mitigate the so-called “red light syndrome” (seen with monochromatic red lighting), which manifests itself in reduced leaf growth and decreased stomatal conductance and photosynthetic capacity (Miao et al., 2019). Lu et al. (2012b) observed the effects of monochromatic supplemental lighting on greenhouse truss tomato plants, showing higher yields in cases of red light application compared to pure blue light application. However, good results were also obtained by using white light containing both red and blue spectral regions in addition to an abundant presence of green light, which may favor light penetration within a canopy and be particularly suitable for single-truss growing systems (Lu et al., 2012b). Deram et al. (2014) and Kaiser et al. (2019a) highlighted the effectiveness of red and blue combinations for tomato production, suggesting red:blue = 4 and red:blue = 1.2–2.4 as optimal ratios for yield improvement, respectively. Kaiser et al. (2019b) also evaluated the partial replacement of red:blue LED lights with different percentages of green light (7, 20, and 39%) in cases of greenhouse tomato production with supplemental artificial lighting. The results showed that the highest studied green percentage (39%), which was similar to the sunlight spectrum, showed the best effects on plant biomass and yield, suggesting that plants may use sunlight-combined wavelengths more efficiently for growth than other wavelength combinations (Kaiser et al., 2019b). The far-red wavelength was also investigated by several studies on greenhouse tomato supplemental LED lighting (Pepin et al., 2014; Hao et al., 2015, 2016; Gómez and Mitchell, 2016b; Song et al., 2016; Dzakovich et al., 2017; Fanwoua et al., 2019; Ji et al., 2019; Kalaitzoglou et al., 2019; Kim et al., 2019, 2020; Zhang et al., 2019). The far-red ratio, particularly the red:far-red ratio, influences phytochrome regulation and has effects on plant architectural development, flower induction, germination, photosynthetic capacity, and nutrition (Demotes-Mainard et al., 2016). Zhang et al. (2019) evaluated the effects of different durations of the far-red lighting supply (namely, 0.5, 1.5, or 12 h day−1) on greenhouse tomato cultivation, concluding that even when adopting the lowest supply time, plant stem elongation was stimulated, thus enhancing light penetration within the canopy. Kalaitzoglou et al. (2019) also highlighted similar far-red-induced morphological and productive effects on tomato plants, although pointing out the necessity for long-term far-red supplies during the day to obtain optimal performances. Furthermore, far-red light may also improve the hedonic perception of tomato fruit (Kim et al., 2020), despite the potential reduction of resistance to Botrytis cinerea (Ji et al., 2019). Finally, Hao et al. (2018) investigated the effects of UV light on tomato yield and did not confirm any significant increase compared to other wavelengths. It should be noted that UV light is traditionally not considered within photosynthetic active radiation (PAR), although recent studies have also attributed the capacity of UV light to foster photosynthesis and growth in plants, e.g., in basil (Dou et al., 2019). Moreover, Tokuno et al. (2012) demonstrated the effectiveness of supplemental UV LED radiation in reducing phytopathological diseases in greenhouse tomato plants. Further research should also specifically target the effect of UV radiation on inducing secondary metabolite production in greenhouse-grown tomato plants, as already observed in several crops (Schreiner et al., 2014).
In addition to lighting quality, the intensity and photoperiod of lighting are also fundamental aspects. Deram et al. (2014) investigated different supplemental LED lighting intensities (135, 115, and 100 μmol m−2 s−1), although no statistically significant differences in plant productivity were observed. However, studies on light intensity are still limited, and further investigations of the optimization of plant photosynthetic responses while minimizing energy costs are needed (Weaver et al., 2019). Concerning the photoperiod, the tomato is a photosensitive species with an optimal photoperiod identified at ~14 h d−1 (Dorais et al., 1996; Demers et al., 1998; Demers and Gosselin, 2000). Continuous lighting (24 h d−1) for 5–7 weeks may improve tomato plant growth and tomato yield, while a longer supply period can have negative effects, likely caused by accumulations of sucrose and starch affecting the maximum quantum efficiency of photosystem II (PSII) with consequent leaf chlorosis (Demers et al., 1998; Demers and Gosselin, 2000; Velez-Ramirez et al., 2017). However, the alternation of red and blue continuous LED lighting was reported to reduce plant injuries, with potential applications for long-term yield improvements (Lanoue et al., 2019). Additionally, the period of the day (daytime or nighttime) in which additional lighting is supplied may affect plant production. Particularly, Tewolde et al. (2016) confronted daytime vs. nighttime (applying light from 4:00 am to 4:00 pm in the case of daytime supply and from 10:00 am to 10:00 pm for nighttime treatment) supplemental LED lighting applications, reporting a significant increase in yield, as well as of soluble solids and ascorbic acid, during wintertime in the case of nighttime supply and also observing better cost-effectiveness of nighttime supply compared to diurnal applications.
The meta-analysis results showed that the application of supplemental LED lighting on greenhouse tomato plants has a statistically significant tendency toward enhancing Yield, TSS, Asc, Chl, PN, and LA, while no significant results were observed for gs (Figure 3). With reference to Yield, although the tendency revealed a global positive effect (with an average yield increase of +40% from the control conditions), some studies reported negative or equal output values compared with their control treatments. These inconsistencies may be attributed to different trial management aspects and should be considered to obtain the best tomato cultivation performance using supplemental LED lighting. Tewolde et al. (2016) observed that daytime LED inter-lighting during summer may reduce tomato yield compared to a solar light control, probably due to the excessive temperature and radiation around the mid-canopy area caused by lamps. A similar effect was also observed by Verheula et al. (2019), equally pointing out the need for ventilation during summer, although with lower energy savings. Additionally, Gómez et al. (2016) reinforced these observations, concluding that supplemental LED lighting may not be a feasible solution during summer even when a root cooling system is used.
Looking at qualitative parameters, while most of the analyzed studies associated supplemental lighting with positive effects (increasing TSS by 6% and Asc by 11%), some inconsistent results were also found. Accordingly, Dzakovich et al. (2015, 2017) reported that supplemental lighting did not increase the TSS values. Similarly, supplemental lighting on tomato plants was not associated with increased TSS values or Asc contents (Lu et al., 2012b) or with the sugar or acid contents, according to Gautier et al. (2005). However, it is important to consider that in addition to light access, other factors may affect the qualitative parameters of tomato fruits (e.g., genotype, environmental conditions, nutrient solution EC) (Kubota et al., 2012; Dzakovich et al., 2015; Ouzounis et al., 2016). Furthermore, it must be acknowledged that the parameters used for the purpose of this research (e.g., TSS and Asc) do not entirely describe tomato fruit quality from a sensorial or nutraceutical standpoint. For instance, due to the scarcity of studies, some qualitative aspects (e.g., antioxidant content) were not evaluated in the present research. Further research on the antioxidant response to supplemental LED lighting is therefore needed, also considering that a potential increase in carotenoids induced by using far-red light has already been reported (Hao et al., 2016).
In this study, the leaf response to supplemental LED lighting was evaluated in terms of Chl, PN, LA, and gs. As already presented within the results (Figure 3), the response ratios [R] for Chl, PN, and LA globally reported statistically significant increases when supplemental LED lighting was applied (on average, increasing Chl by 31%, PN by 50%, and LA by 9%). Additionally, for these parameters, however, inconsistencies were observed among studies. In particular, Kim et al. (2019) observed reductions in Chl and LA in plants treated with low red:far-red levels for long durations, which may be attributed to major biomass allocations in reproductive structures during plant growth and development. Other authors also observed non-statistically significant differences in both the chlorophyll content and total leaf area (Jiang et al., 2017) or in the leaf area only (Gómez and Mitchell, 2016b) when applying supplemental lighting treatments. No statistically significant effect of supplemental LED lighting application on PN was observed by Gajc-Wolska et al. (2013) or by Gómez et al. (2016) compared to the control conditions. From the meta-analysis, a non-significant effect of supplemental lighting on gs was observed, possibly suggesting that excessive light irradiance could also lead to stomatal closure (O'Carrigan et al., 2014a).
The evaluation of heterogeneity among the studies showed high values for each response ratio [R] (Table 1). Such results were, however, expected, considering not only the variability in trial management (e.g., diverse locations and technologies, light qualities, intensities, growing systems, etc.) but also the absence of common meta-data protocols for data collection and presentation. The last can be seen as one of the main issues hindering the development of agricultural meta-analyses, and this challenge should be overcome by always presenting all the statistical values needed for a meta-analysis evaluation (e.g., the standard error, standard deviation, variance, and sample size), as well as by applying common measurement methods (Eagle et al., 2017). The lower heterogeneity observed for TSS than for the other factors may be attributed to different measuring systems or units, while the other evaluated effect sizes utilized different measurement standards that required unit conversions in some cases. Concerning the subgroup analysis, most of the confronted group showed high heterogeneity, indicating the absence of influences determined by specific trial characteristics. However, low heterogeneity was observed for Yield in cases of lighting supplies different from intracanopy lighting or pure HPS lamps used as controls, for TSS in cases of increased natural light or other lighting supplies, and for Chl in cases of decreased natural lighting. Accordingly, the analysis revealed common trends of results in these specific subgroups. However, further targeted assumptions regarding the effect of specific LED lighting features (e.g., decreasing or increasing natural sunlight; intracanopy or other light supplies) on the combined effect sizes cannot be hypothesized due to the absence of homogeneity in the confronted group.
Conclusion
Despite some limitations commonly occurring in agricultural meta-analyses, the research conducted herein revealed that supplemental LED lighting may be effective in improving the quantitative and qualitative aspects of greenhouse-grown truss tomato production. Significant positive results were observed for both direct qualitative-quantitative parameters (Yield, TSS, Asc) and crop photosynthetic properties (Chl, PN, LA), while only stomatal conductance (gs) was not significantly affected by supplemental LED lighting. Further research is needed regarding product quality, particularly focusing on the unexplored effects of LED lighting on nutraceutical properties and organoleptic features. Moreover, most studies considered herein applied red and blue spectra, although preliminary studies have also introduced promising results by applying UV or green light. Finally, the collected studies were mainly concentrated in the northern part of the boreal hemisphere, where the presence of technologically advanced greenhouses, as well as some favorable environmental conditions due to lower temperatures and sun radiation, have induced the wide uptake of horticultural LED technology. However, interesting applications may also be hypothesized for milder climates such as those of the Mediterranean area, in which supplemental LED lighting could improve the quantitative and qualitative aspects of greenhouse tomato plants both during the off-season and when extremely hot summers occur and intensive shading is needed.
Data Availability Statement
The authors confirm that the data supporting the findings of this study are available within the article and its Supplementary Materials.
Author Contributions
EA designed and performed the data collection and meta data analysis, and drafted the manuscript. GP and FO contributed to the research design and the drafting of the manuscript. IP revised the manuscript. XG and GG supervised the research and critically revised the manuscript. All authors contributed to the article and approved the submitted version.
Funding
The research leading to this publication has received funding from the European Union's Horizon 2020 research and innovation programme under grant agreement No 862663.
Disclaimer
The publication reflects the author's views. The Research Executive Agency (REA) is not liable for any use that may be made of the information contained therein.
Conflict of Interest
The authors declare that the research was conducted in the absence of any commercial or financial relationships that could be construed as a potential conflict of interest.
Supplementary Material
The Supplementary Material for this article can be found online at: https://www.frontiersin.org/articles/10.3389/fpls.2021.596927/full#supplementary-material
References
Bastian, M., Heymann, S., and Jacomy, M. (2009). “Gephi: an open source software for exploring and manipulating networks,” in International AAAI Conference on Weblogs and Social Media (San Jose, CA).
Bian, Z., Zhang, X., Wang, Y., and Lu, C. (2019). Improving drought tolerance by altering the photosynthetic rate and stomatal aperture via green light in tomato (Solanum lycopersicum L.) seedlings under drought conditions. Environ. Exp. Bot. 167:103844. doi: 10.1016/j.envexpbot.2019.103844
Borenstein, M., Hedges, L. V., Higgins, J. P. T., and Rothstein, H. R. (2009). Introduction to Meta-Analysis. Chichester: Wiley. doi: 10.1002/9780470743386
Brazaityte, A., Duchovskis, P., Urbonavičiute, A., Samuoliene, G., Jankauskiene, J., Kazenas, V., et al. (2009). After-effect of light-emitting diodes lighting on tomato growth and yield in greenhouse. Sodininkyste ir darŽininkyste 28, 115–126.
Cao, K., Cui, L., Ye, L., Zhou, X., Bao, E., Zhao, H., et al. (2016). Effects of red light night break treatment on growth and flowering of tomato plants. Front. Plant Sci. 7:527. doi: 10.3389/fpls.2016.00527
Carvalho, S. D., Schwieterman, M. L., Abrahan, C. E., Colquhoun, T. A., and Folta, K. M. (2016). Light quality dependent changes in morphology, antioxidant capacity, and volatile production in sweet basil (Ocimum basilicum). Front. Plant Sci. 7:1328. doi: 10.3389/fpls.2016.01328
Castilla, N., and Baeza, E. (2013). Greenhouse site selection. Good Agricultural Practices for greenhouse vegetable crops: principles for mediterranean climate areas. FAO plant production and protection paper, FAO, 21–34.
Castilla, N., Hernández, J., and Abou-Hadid, A. F. (2002). Strategic crop and greenhouse management in mild winter climate areas. Acta Hortic. 633, 183–196. doi: 10.17660/actahortic.2004.633.22
Christie, J. M., Blackwood, L., Petersen, J., and Sullivan, S. (2015). Plant flavoprotein photoreceptors. Plant Cell Physiol. 56, 401–413. doi: 10.1093/pcp/pcu196
Demers, D. A., Dorais, M., Wien, C. H., and Gosselin, A. (1998). Effects of supplemental light duration on greenhouse tomato (Lycopersicon esculentum Mill.) plants and fruit yields. Sci. Hortic. 74, 295–306. doi: 10.1016/s0304-4238(98)00097-1
Demers, D. A., and Gosselin, A. (2000). Growing greenhouse tomato and sweet pepper under supplemental lighting: optimal photoperiod, negative effects of long photoperiod and their causes. Acta Hortic. 580, 83–88. doi: 10.17660/actahortic.2002.580.9
Demotes-Mainard, S., Péron, T., Corot, A., Bertheloot, J., Le Gourrierec, J., Pelleschi-Travier, S., et al. (2016). Plant responses to red and far-red lights, applications in horticulture. Environ. Exp. Bot. 121, 4–21. doi: 10.1016/j.envexpbot.2015.05.010
Deram, P., Lefsrud, M. G., and Orsat, V. (2014). Supplemental lighting orientation and red-to-blue ratio of light-emitting diodes for greenhouse tomato production. HortScience 49, 448–452. doi: 10.21273/hortsci.49.4.448
Dorais, M., Gosselin, A., and Trudel, M. J. (1991). Annual greenhouse tomato production under a sequential intercropping system using supplemental light. Sci. Hortic. 45, 225–234. doi: 10.1016/0304-4238(91)90067-9
Dorais, M., Yelle, S., and Gosselin, A. (1996). Influence of extended photoperiod on photosynthate partitioning and export in tomato and pepper plants. N. Z. J. Crop Hort. 24, 29–37. doi: 10.1080/01140671.1996.9513932
Dou, H., Niu, G., and Gu, M. (2019). Pre-harvest UV-B radiation and photosynthetic photon flux density interactively affect plant photosynthesis, growth, and secondary metabolites accumulation in basil (Ocimum basilicum) plants. Agronomy 9:434. doi: 10.3390/agronomy9080434
D'Souza, C., Yuk, H. G., Khoo, G. H., and Zhou, W. (2015). Application of light-emitting diodes in food production, postharvest preservation, and microbiological food safety. Compr. Rev. Food Sci. F 14, 719–740. doi: 10.1111/1541-4337.12155
Dueck, T. A., Janse, J., Eveleens, B. A., Kempkes, F. L. K., and Marcelis, L. F. M. (2011). Growth of tomatoes under hybrid LED and HPS lighting. Acta Hortic. 952, 335–342. doi: 10.17660/actahortic.2012.952
Dzakovich, M. P., Gómez, C., Ferruzzi, M. G., and Mitchell, C. A. (2017). Chemical and sensory properties of greenhouse tomatoes remain unchanged in response to Red, Blue, and Far Red supplemental light from Light-Emitting Diodes. HortScience 52, 1734–1741. doi: 10.21273/hortsci12469-17
Dzakovich, M. P., Gómez, C., and Mitchell, C. A. (2015). Tomatoes grown with light-emitting diodes or high-pressure sodium supplemental lights have similar fruit-quality attributes. HortScience 50, 1498–1502. doi: 10.21273/hortsci.50.10.1498
Eagle, A. J., Christianson, L. E., Cook, R. L., Harmel, R. D., Miguez, F. E., Qian, S. S., et al. (2017). Meta-analysis constrained by data: recommendations to improve relevance of nutrient management research. J. Agron. 109, 2441–2449. doi: 10.2134/agronj2017.04.0215
Ebisawa, M., Shoji, K., Kato, M., Shimomura, K., Goto, F., and Yoshihara, T. (2008). Supplementary ultraviolet radiation B together with blue light at night increased quercetin content and flavonol synthase gene expression in leaf lettuce (Lactuca sativa L.). Environ. Control Biol. 46, 1–11. doi: 10.2525/ecb.46.1
Fanwoua, J., Vercambre, G., Buck-Sorlin, G., Dieleman, J. A., de Visser, P., and Génard, M. (2019). Supplemental LED lighting affects the dynamics of tomato fruit growth and composition. Sci. Hortic. 256:108571. doi: 10.1016/j.scienta.2019.108571
FAO (2018). FAOSTAT database collections. Rome: Food and Agriculture Organization of the United Nations. Available online at: http://faostat.fao.org (accessed June 24, 2020).
Fernández, J. A., Orsini, F., Baeza, E., Oztekin, G. B., Muñoz, P., Contreras, J., et al. (2018). Current trends in protected cultivation in Mediterranean climates. Eur. J. Hortic. Sci. 83, 294–305. doi: 10.17660/ejhs.2018/83.5.3
Fischer, G., Nachtergaele, F. O., Prieler, S., Teixeira, E., Tóth, G., Van Velthuizen, H., et al. (2012). Global agro-ecological zones (GAEZ v3. 0) - Model Documentation. IIASA, FAO, Rome, Italy.
Gajc-Wolska, J., Kowalczyk, K., Metera, A., Mazur, K., Bujalski, D., and Hemka, L. (2013). Effect of supplementary lighting on selected physiological parameters and yielding of tomato plants. Folia Horticult. 25, 153–159. doi: 10.2478/fhort-2013-0017
Garcia, M. L., Medrano, E., Sanchez-Guerrero, M. C., and Lorenzo, P. (2011). Climatic effects of two cooling systems in greenhouses in the Mediterranean area: external mobile shading and fog system. Biosyst. Eng. 108, 133–143. doi: 10.1016/j.biosystemseng.2010.11.006
Garland, K. F., Burnett, S. E., Stack, L. B., and Zhang, D. (2010). Minimum daily light integral for growing high-quality coleus. Horttechnology 20, 929–933. doi: 10.21273/HORTTECH.20.5.929
Gautier, H., Rocci, A., Buret, M., Grasselly, D., Dumas, Y., and Causse, M. (2005). Effect of photoselective filters on the physical and chemical traits of vine-ripened tomato fruits. Can. J. Plant Sci. 85, 439–446. doi: 10.4141/p03-163
Giniger, M. S., McAvoy, R. J., Giacomelli, G. A., and Janes, H. W. (1988). Computer simulation of a single truss tomato cropping system. Trans. ASAE 31, 1176–1179. doi: 10.13031/2013.30840
Gómez, C., Clark, M., and Mitchell, C. A. (2016). Effect of intracanopy lighting and/or root-zone temperature on high-wire tomato production under supra-optimal air temperature. Acta Hortic. 1134, 63–70. doi: 10.17660/ActaHortic.2016.1134.9
Gómez, C., and Mitchell, C. A. (2016a). Physiological and productivity responses of high-wire tomato as affected by supplemental light source and distribution within the canopy. J. Am. Soc. Hortic. Sci. 141, 196–208. doi: 10.21273/jashs.141.2.196
Gómez, C., and Mitchell, C. A. (2016b). In search of an optimized supplemental lighting spectrum for greenhouse tomato production with intracanopy lighting. Acta Hortic. 1134, 57–62. doi: 10.17660/actahortic.2016.1134.8
Gomez, C., Morrow, R. C., Bourget, C. M., Massa, G. D., and Mitchell, C. A. (2013). Comparison of intracanopy light-emitting diode towers and overhead high-pressure sodium lamps for supplemental lighting of greenhouse-grown tomatoes. Horttechnology 23, 93–98. doi: 10.21273/HORTTECH.23.1.93
Gunnlaugsson, B., and Adalsteinsson, S. (2005). Interlight and plant density in year-round production of tomato at northern latitudes. Acta Hortic. 711, 71–76. doi: 10.17660/actahortic.2006.711.6
Guo, X., Hao, X., Khosla, S., Kumar, K. G. S., Cao, R., and Bennett, N. (2016). Effect of LED interlighting combined with overhead HPS light on fruit yield and quality of year-round sweet pepper in commercial greenhouse. Acta Hortic. 1134, 71–78. doi: 10.17660/actahortic.2016.1134.10
Gupta, S. D., and Agarwal, A. (2017). “Artificial lighting system for plant growth and development: Chronological advancement, working principles, and comparative assessmen,” in Light Emitting Diodes for Agriculture, ed S. D. Gupta (Singapore: Springer), 1–25. doi: 10.1007/978-981-10-5807-3_1
Hak, T., Van Rhee, H. J., and Suurmond, R. (2016). How to Interpret Results of Meta-Analysis. (Version 1.3). Rotterdam: Erasmus Rotterdam Institute of Management. doi: 10.2139/ssrn.3241367
Hamamoto, H., and Yamazaki, K. (2009). Supplemental lighting inside the plant canopy increased the yield and quality of three-truss-ordered tomato. Acta Hortic. 907, 283–286. doi: 10.17660/actahortic.2011.907.45
Hao, X., Cao, R., Zheng, J., Little, C., Kholsa, S., and Yelton, M. (2018). Optimizing vertical light spectral distribution to improve yield and quality in greenhouse fruit vegetable production. Acta Hortic. 1271, 141–148. doi: 10.17660/actahortic.2020.1271.20
Hao, X., Guo, X., Lanoue, J., Zhang, Y., Cao, R., Zheng, J., et al. (2017). A review on smart application of supplemental lighting in greenhouse fruiting vegetable production. Acta Hortic. 1227, 499–506. doi: 10.17660/ActaHortic.2018.1227.63
Hao, X., Guo, X., Zheng, J., Celeste, L., Kholsa, S., and Chen, X. (2015). Response of greenhouse tomato to different vertical spectra of LED lighting under overhead high pressure sodium and plasma lighting. Acta Hortic. 1170, 1003–1110. doi: 10.17660/actahortic.2017.1170.129
Hao, X., Little, C., Zheng, J. M., and Cao, R. (2016). Far-red LEDs improve fruit production in greenhouse tomato grown under high-pressure sodium lighting. Acta Hortic. 1134, 95–102. doi: 10.17660/actahortic.2016.1134.13
Hao, X., Zheng, J., Little, C., and Khosla, S. (2012). LED inter-lighting in year-round greenhouse mini-cucumber production. Acta Hortic. 956, 335–340. doi: 10.17660/ActaHortic.2012.956.38
Hedges, L. V., Gurevitch, J., and Curtis, P. S. (1999). The meta-analysis of response ratios in experimental ecology. Ecology 80, 1150–1156. doi: 10.1890/0012-9658(1999)080[1150:TMAORR]2.0.CO;2
Heuvelink, E., and Gonzalez-Real, M. M. (2007). Innovation in plant-greenhouse interactions and crop management. Acta Hortic. 80, 63–74. doi: 10.17660/actahortic.2008.801.2
Hinov, N., Tsankov, P., and Ibrishimov, H. (2019). “Innovative LED lighting,” in 2019 International Conference on Creative Business for Smart and Sustainable Growth (CREBUS) (Sandanski), 1–5. doi: 10.1109/CREBUS.2019.8840095
Hui, X., Fu, Y. N., Li, T. L., and Rui, W. (2017). Effects of different LED light wavelengths on the resistance of tomato against Botrytis cinerea and the corresponding physiological mechanisms. J. Integr. Agric. 16, 106–114. doi: 10.1016/s2095-3119(16)61435-1
Ibaraki, Y. (2017). “LED supplementary lighting,” in Light Emitting Diodes for Agriculture, ed S. D. Gupta (Singapore: Springer), 27–36. doi: 10.1007/978-981-10-5807-3_2
Ieperen, W. V., Savvides, A., and Fanourakis, D. (2012). Red and blue light effects during growth on hydraulic and stomatal conductance in leaves of young cucumber plants. Acta Hortic. 956, 223–230. doi: 10.17660/actahortic.2012.956.24
Ji, Y., Ouzounis, T., Courbier, S., Kaiser, E., Nguyen, P. T., Schouten, H. J., et al. (2019). Far-red radiation increases dry mass partitioning to fruits but reduces Botrytis cinerea resistance in tomato. Environ. Exp. Bot. 168:103889. doi: 10.1016/j.envexpbot.2019.103889
Jiang, C., Johkan, M., Hohjo, M., Tsukagoshi, S., Ebihara, M., Nakaminami, A., et al. (2017). Photosynthesis, plant growth, and fruit production of single-truss tomato improves with supplemental lighting provided from underneath or within the inner canopy. Sci. Hort. 222, 221–229. doi: 10.1016/j.scienta.2017.04.026
Johkan, M., Hohjo, T., Tsukagoshi, S., Ebihara, M., Nakaminami, A., Maruo, M., et al. (2017). Supplemental lighting applied within or underneath the canopy enhances leaf photosynthesis, stomatal regulation and plant development of tomato under limiting light conditions. Acta Hortic. 1227, 645–652. doi: 10.17660/actahortic.2018.1227.82
Johkan, M., Shoji, K., Goto, F., Hashida, S. N., and Yoshihara, T. (2010). Blue light-emitting diode light irradiation of seedlings improves seedling quality and growth after transplanting in red leaf lettuce. HortScience 45, 1809–1814. doi: 10.21273/hortsci.45.12.1809
Jokinen, K., Särkkä, L. E., and Näkkilä, J. (2012). Improving sweet pepper productivity by LED interlighting. Acta Hortic. 956, 59–66. doi: 10.17660/actahortic.2012.956.4
Joshi, N. C., Ratner, K., Eidelman, O., Bednarczyk, D., Zur, N., Many, Y., et al. (2019). Effects of daytime intra-canopy LED illumination on photosynthesis and productivity of bell pepper grown in protected cultivation. Sci. Hort. 250, 81–88. doi: 10.1016/j.scienta.2019.02.039
Kaiser, E., Ouzounis, T., Giday, H., Schipper, R., Heuvelink, E., and Marcelis, L. F. (2019a). Adding blue to red supplemental light increases biomass and yield of greenhouse-grown tomatoes, but only to an optimum. Front. Plant Sci. 9:2002. doi: 10.3389/fpls.2018.02002
Kaiser, E., Weerheim, K., Schipper, R., and Dieleman, J. A. (2019b). Partial replacement of red and blue by green light increases biomass and yield in tomato. Sci. Hort. 249, 271–279. doi: 10.1016/j.scienta.2019.02.005
Kalaitzoglou, P., Van Ieperen, W., Harbinson, J., van der Meer, M., Martinakos, S., Weerheim, K., et al. (2019). Effects of continuous or end-of-day far-red light on tomato plant growth, morphology, light absorption and fruit production. Front. Plant Sci. 10:322. doi: 10.3389/fpls.2019.00322
Kim, H. J., Lin, M. Y., and Mitchell, C. A. (2019). Light spectral and thermal properties govern biomass allocation in tomato through morphological and physiological changes. Environ. Exp. Bot. 157, 228–240. doi: 10.1016/j.envexpbot.2018.10.019
Kim, H. J., Yang, T., Choi, S., Wang, Y. J., Lin, M. Y., and Liceaga, A. M. (2020). Supplemental intracanopy far-red radiation to red LED light improves fruit quality attributes of greenhouse tomatoes. Sci. Hort. 261:108985. doi: 10.1016/j.scienta.2019.108985
Kittas, C., Baille, A., and Giaglaras, P. (1999). Influence of covering material and shading on the spectral distribution of light in greenhouses. J. Agric. Eng. Res. 73, 341–351. doi: 10.1006/jaer.1999.0420
Kubota, C., de Gelder, A., and Peet, M. M. (2018). “Greenhouse tomato production,” in Tomatoes, ed E. Heuvelink (Wageningen: CABI), 276–314. doi: 10.1079/9781780641935.0276
Kubota, C., Kroggel, M., Both, A. J., Burr, J. F., and Whalen, M. (2016). Does supplemental lighting make sense for my crop? – empirical evaluations. Acta Hortic. 1134, 403–412. doi: 10.17660/actahortic.2016.1134.52
Kubota, C., Kroggel, M., Torabi, M., Dietrich, K. A., Kim, H. J., Fonseca, J., et al. (2012). Changes in selected quality attributes of greenhouse tomato fruit as affected by pre-and postharvest environmental conditions in year-round production. HortScience 47, 1698–1704. doi: 10.21273/hortsci.47.12.1698
Kumar, K. G. S., Hao, X., Khosla, S., Guo, X., and Bennett, N. (2016). Comparison of HPS lighting and hybrid lighting with top HPS and intra-canopy LED lighting for high-wire mini-cucumber production. Acta Hortic. 1134, 111–118. doi: 10.17660/actahortic.2016.1134.15
Lanoue, J., Zheng, J., Little, C., Thibodeau, A., Grodzinski, B., and Hao, X. (2019). Alternating Red and Blue Light-Emitting Diodes allows for injury-free tomato production with continuous lighting. Front. Plant Sci. 10:1114. doi: 10.3389/fpls.2019.01114
Liao, Y., Suzuki, K., Yu, W., Zhuang, D., Takai, Y., Ogasawara, R., et al. (2014). Night break effect of LED light with different wavelengths on floral bud differentiation of Chrysanthemum morifolium ramat ‘Jimba’and Iwa no hakusen. Environ. Exp. Bot. 52, 45–50. doi: 10.2525/ecb.52.45
Lu, N., Maruo, T., Johkan, M., Hohjo, M., Tsukagoshi, S., Ito, Y., et al. (2012a). Effects of supplemental lighting within the canopy at different developing stages on tomato yield and quality of single-truss tomato plants grown at high density. Environ. Exp. Bot. 50, 1–11. doi: 10.2525/ecb.50.1
Lu, N., Maruo, T., Johkan, M., Hohjo, M., Tsukagoshi, S., Ito, Y., et al. (2012b). Effects of supplemental lighting with light-emitting diodes (LEDs) on tomato yield and quality of single-truss tomato plants grown at high planting density. Environ. Exp. Bot. 50, 63–74. doi: 10.2525/ecb.50.63
McCree, K. J. (1971). The action spectrum, absorptance and quantum yield of photosynthesis in crop plants. J. Agric. Meteorol. 9, 191–216. doi: 10.1016/0002-1571(71)90022-7
McDaniel, M. D., Tiemann, L. K., and Grandy, A. S. (2014). Does agricultural crop diversity enhance soil microbial biomass and organic matter dynamics? A meta-analysis. Ecol. Appl. 24, 560–570. doi: 10.1890/13-0616.1
Mempel, H., and Wittmann, S. (2019). Potential and use of artificial lighting in horticulture. Light Eng. 27, 31–41.
Ménard, C., Dorais, M., Hovi, T., and Gosselin, A. (2005). Developmental and physiological responses of tomato and cucumber to additional blue light. Acta Hortic. 711, 291–296. doi: 10.17660/actahortic.2006.711.39
Meneses, J. F., and Castilla, N. (2009). Protected cultivation in Iberian horticulture. Chron. Horticult. 49, 37–39.
Miao, Y., Chen, Q., Qu, M., Gao, L., and Hou, L. (2019). Blue light alleviates ‘red light syndrome’ by regulating chloroplast ultrastructure, photosynthetic traits and nutrient accumulation in cucumber plants. Sci. Hort. 257:108680. doi: 10.1016/j.scienta.2019.108680
Morrow, R. C. (2008). LED lighting in horticulture. HortScience 43, 1947–1950. doi: 10.21273/hortsci.43.7.1947
Nelson, J. A., and Bugbee, B. (2014). Economic analysis of greenhouse lighting: light emitting diodes vs. high intensity discharge fixtures. PLoS ONE 9:e99010. doi: 10.1371/journal.pone.0099010
O'Carrigan, A., Babla, M., Wang, F., Liu, X., Mak, M., Thomas, R., et al. (2014b). Analysis of gas exchange, stomatal behaviour and micronutrients uncovers dynamic response and adaptation of tomato plants to monochromatic light treatments. Plant Physiol. Biochem. 82, 105–115. doi: 10.1016/j.plaphy.2014.05.012
O'Carrigan, A., Hinde, E., Lu, N., Xu, X. Q., Duan, H., Huang, G., et al. (2014a). Effects of light irradiance on stomatal regulation and growth of tomato. Environ. Exp. Bot. 98, 65–73. doi: 10.1016/j.envexpbot.2013.10.007
Ohashi-Kaneko, K., Takase, M., Kon, N., Fujiwara, K., and Kurata, K. (2007). Effect of light quality on growth and vegetable quality in leaf lettuce, spinach and komatsuna. Envrion. Control Biol. 45, 189–198. doi: 10.2525/ecb.45.189
Okano, K., Nakano, Y., and Watanabe, S. I. (2001). Single-truss tomato system—a labor-saving management system for tomato production. Jpn. Agr. Res. Q. 35, 177–184. doi: 10.6090/jarq.35.177
Olle, M., and Viršile, A. (2013). The effects of light-emitting diode lighting on greenhouse plant growth and quality. Agr. Food Sci. 22, 223–234. doi: 10.23986/afsci.7897
OMAFRA (2001). Growing Greenhouse Vegetables. Publication 371. Ontario Ministry of Agriculture, Food and Rural Affairs, Toronto, Canada, 116.
Ouzounis, T., Heuvelink, E., Ji, Y., Schouten, H. J., Visser, R. G. F., and Marcelis, L. F. M. (2016). Blue and red LED lighting effects on plant biomass, stomatal conductance, and metabolite content in nine tomato genotypes. Acta Hortic. 1134, 251–258. doi: 10.17660/actahortic.2016.1134.34
Ouzounis, T., Rosenqvist, E., and Ottosen, C. O. (2015). Spectral effects of artificial light on plant physiology and secondary metabolism: a review. HortScience 50, 1128–1135. doi: 10.21273/hortsci.50.8.1128
Paponov, M., Kechasov, D., Lacek, J., Verheul, M., and Paponov, I. A. (2019). Supplemental LED inter-lighting increases tomato fruit growth through enhanced photosynthetic light use efficiency and modulated root activity. Front. Plant Sci. 10:1656. doi: 10.3389/fpls.2019.01656
Paucek, I., Pennisi, G., Pistillo, A., Appolloni, E., Crepaldi, A., Calegari, B., et al. (2020). Supplementary LED interlighting improves yield and precocity of greenhouse tomatoes in the mediterranean. Agron 10:1002. doi: 10.3390/agronomy10071002
Pennisi, G., Blasioli, S., Cellini, A., Maia, L., Crepaldi, A., Braschi, I., et al. (2019b). Unravelling the role of red:blue LED lights on resource use efficiency and nutritional properties of indoor grown sweet basil. Front. Plant Sci. 10:305. doi: 10.3389/fpls.2019.00305
Pennisi, G., Orsini, F., Blasioli, S., Cellini, A., Crepaldi, A., Braschi, I., et al. (2019a). Resource use efficiency of indoor lettuce (Lactuca sativa L.) cultivation as affected by red:blue ratio provided by LED lighting. Sci. Rep. 9:14127. doi: 10.1038/s41598-019-50783-z
Pepin, S., Fortier, E., Béchard-Dubé, S. A., Dorais, M., Ménard, C., and Bacon, R. (2014). Beneficial effects of using a 3-D LED interlighting system for organic greenhouse tomato grown in Canada under low natural light conditions. Acta Hortic. 1041, 239–246. doi: 10.17660/actahortic.2014.1041.28
Petropoulos, S. A., Fernandes, Â., Katsoulas, N., Barros, L., and Ferreira, I. C. (2019). The effect of covering material on the yield, quality and chemical composition of greenhouse-grown tomato fruit. J. Sci. Food Agric. 99, 3057–3068. doi: 10.1002/jsfa.9519
Qin, W., Hu, C., and Oenema, O. (2015). Soil mulching significantly enhances yields and water and nitrogen use efficiencies of maize and wheat: a meta-analysis. Sci. Rep. 5:16210. doi: 10.1038/srep16210
Roelfsema, M. R. G., and Hedrich, R. (2005). In the light of stomatal opening: new insights into ‘the Watergate’. New Phytol. 167, 665–691. doi: 10.1111/j.1469-8137.2005.01460.x
Sarlikioti, V., de Visser, P. H., Buck-Sorlin, G. H., and Marcelis, L. F. M. (2011). How plant architecture affects light absorption and photosynthesis in tomato: towards an ideotype for plant architecture using a functional–structural plant model. Ann. Bot. 108, 1065–1073. doi: 10.1093/aob/mcr221
Schreiner, M., Martínez-Abaigar, J., Glaab, J., and Jansen, M. (2014). UV-B induced secondary plant metabolites: potential benefits for plant and human health. Optik (Stuttg) 9, 34–37. doi: 10.1002/opph.201400048
Schwarz, D., Thompson, A. J., and Kläring, H. P. (2014). Guidelines to use tomato in experiments with a controlled environment. Front. Plant Sci. 5:625. doi: 10.3389/fpls.2014.00625
Shimazaki, K. I., Doi, M., Assmann, S. M., and Kinoshita, T. (2007). Light regulation of stomatal movement. Ann. Rev. Plant Biol. 58, 219–247. doi: 10.1146/annurev.arplant.57.032905.105434
Song, Y., Jiang, C., and Gao, L. (2016). Polychromatic supplemental lighting from underneath canopy is more effective to enhance tomato plant development by improving leaf photosynthesis and stomatal regulation. Front. Plant Sci. 7:1832. doi: 10.3389/fpls.2016.01832
Sun, Y., Sun, Y., Sun, Z., Li, P., and Li, C. (2015). “Mapping monthly distribution of daily light integrals across China,” in Annual Conference of ASHS (New Orleans).
Suurmond, R., van Rhee, H., and Hak, T. (2017). Introduction, comparison and validation of Meta-Essentials: a free and simple tool for meta-analysis. Res. Synth. Methods 8, 537–553. doi: 10.1002/jrsm.1260
Suzuki, K., Yasuba, K., Takaichi, M., Takahashi, T., and Hoshi, T. (2009). Effect of the supplemental lighting on the growth of young plants in second nursery in tomato. Acta Hortic. 907, 269–276. doi: 10.17660/actahortic.2011.907.43
Talbott, L. D., Nikolova, G., Ortiz, A., Shmayevich, I., and Zeiger, E. (2002). Green light reversal of blue-light-stimulated stomatal opening is found in a diversity of plant species. Am. J. Bot. 89, 366–368. doi: 10.3732/ajb.89.2.366
Tewolde, F. T., Lu, N., Shiina, K., Maruo, T., Takagaki, M., Kozai, T., et al. (2016). Nighttime supplemental LED inter-lighting improves growth and yield of single-truss tomatoes by enhancing photosynthesis in both winter and summer. Front. Plant Sci. 7:448. doi: 10.3389/fpls.2016.00448
Tewolde, F. T., Shiina, K., Maruo, T., Takagaki, M., Kozai, T., and Yamori, W. (2018). Supplemental LED inter-lighting compensates for a shortage of light for plant growth and yield under the lack of sunshine. PLoS ONE 13:e0206592. doi: 10.1371/journal.pone.0206592
Tokuno, A., Iaraki, Y., Ito, S. I., Araki, H., Yoshimura, K., and Osaki, K. (2012). Disease suppression in greenhouse tomato by supplementary lighting with 405 nm LED. Environ. Control. Biol. 50, 19–29. doi: 10.2525/ecb.50.19
Trouwborst, G., Sander, W. H., Harbinson, J., and Wim Van, I. (2011). The influence of light intensity and leaf age on the photosynthetic capacity of leaves within a tomato canopy. J. Hortic. Sci. Biotech. 86, 403–407. doi: 10.1080/14620316.2011.11512781
Tuzel, Y., and Oztekin, G. B. (2014). Recent developments of vegetables protected cultivation in Turkey. Acta Hortic. 1142, 435–442. doi: 10.17660/actahortic.2016.1142.66
Velez-Ramirez, A. I., Carreño-Quintero, N., Vreugdenhil, D., Millenaar, F. F., and van Ieperen, W. (2017). Sucrose and starch content negatively correlates with PSII maximum quantum efficiency in tomato (Solanum lycopersicum) exposed to abnormal light/dark cycles and continuous light. Plant Cell Physiol. 58, 1339–1349. doi: 10.1093/pcp/pcx068
Verheula, M. J., Maessen, H. F. R., Panosyan, A., Paponov, M., and Paponov, I. A. (2019). Effects of supplemental light and temperature on summer production of tomato in Norway. Available online at: https://vb.northsearegion.eu/public/files/repository/20190925153007_2019-10m%C3%B8de.pdf (accessed June 24, 2020).
Wada, T., Ikeda, H., Matsushita, K., Kambara, A., Hirai, H., and Abe, K. (2006). Effect of shading in summer on yield and quality of tomatoes grown on a single-truss system. J. Jpn. Soc. Hortic. Sci. 75, 51–58. doi: 10.2503/jjshs.75.51
Weaver, G. M., van Iersel, M. W., and Velni, J. M. (2019). A photochemistry-based method for optimising greenhouse supplemental light intensity. Biosyst. Eng. 182, 123–137. doi: 10.1016/j.biosystemseng.2019.03.008
Zeiger, E. (1990). Light perception in guard cells. Plant, Cell Environ. 13, 739–744. doi: 10.1111/j.1365-3040.1990.tb01088.x
Zhang, G., Shen, S., Takagaki, M., Kozai, T., and Yamori, W. (2015). Supplemental upward lighting from underneath to obtain higher marketable lettuce (Lactuca sativa) leaf fresh weight by retarding senescence of outer leaves. Front. Plant Sci. 6:1110. doi: 10.3389/fpls.2015.01110
Keywords: supplemental light, LED, greenhouse, Solanum lycopersicum, interlighting
Citation: Appolloni E, Orsini F, Pennisi G, Gabarrell Durany X, Paucek I and Gianquinto G (2021) Supplemental LED Lighting Effectively Enhances the Yield and Quality of Greenhouse Truss Tomato Production: Results of a Meta-Analysis. Front. Plant Sci. 12:596927. doi: 10.3389/fpls.2021.596927
Received: 20 August 2020; Accepted: 06 April 2021;
Published: 29 April 2021.
Edited by:
Bernard Grodzinski, University of Guelph, CanadaReviewed by:
Mark Lefsrud, McGill University, CanadaJason Lanoue, Agriculture and Agri-Food Canada (AAFC), Canada
Copyright © 2021 Appolloni, Orsini, Pennisi, Gabarrell Durany, Paucek and Gianquinto. This is an open-access article distributed under the terms of the Creative Commons Attribution License (CC BY). The use, distribution or reproduction in other forums is permitted, provided the original author(s) and the copyright owner(s) are credited and that the original publication in this journal is cited, in accordance with accepted academic practice. No use, distribution or reproduction is permitted which does not comply with these terms.
*Correspondence: Francesco Orsini, f.orsini@unibo.it