- 1Key Laboratory for Quality and Safety Control of Subtropical Fruits and Vegetables, Collaborative Innovation Center for Efficient and Green Production of Agriculture in Mountainous Areas of Zhejiang Province, Ministry of Agriculture and Rural Affairs, College of Horticulture Science, Zhejiang Agriculture and Forestry University, Hangzhou, China
- 2Ministry of Agriculture Key Laboratory of Biology and Genetic Resource Utilization of Rubber Tree, State Key Laboratory Breeding Base of Cultivation and Physiology for Tropical Crops, Rubber Research Institute, Chinese Academy of Tropical Agricultural Sciences, Danzhou, China
- 3College of Jiyang, Zhejiang Agriculture and Forestry University, Zhuji, China
- 4Institute of Ecological Civilization, Zhejiang Agriculture and Forestry University, Hangzhou, China
Cucumber (Cucumis sativus L.) is an important economic vegetable crop worldwide that is susceptible to various common pathogens, including powdery mildew (PM), downy mildew (DM), and Fusarium wilt (FM). In cucumber breeding programs, identifying disease resistance and related molecular markers is generally a top priority. PM, DM, and FW are the major diseases of cucumber in China that cause severe yield losses and the genetic-based cucumber resistance against these diseases has been developed over the last decade. Still, the molecular mechanisms of cucumber disease resistance remain unclear. In this review, we summarize recent findings on the inheritance, molecular markers, and quantitative trait locus mapping of cucumber PM, DM, and FM resistance. In addition, several candidate genes, such as PM, DM, and FM resistance genes, with or without functional verification are reviewed. The data help to reveal the molecular mechanisms of cucumber disease resistance and provide exciting new opportunities for further resistance breeding.
Introduction
Cucumber (Cucumis sativus L.) is a popular vegetable grown on a large scale worldwide. It has an edible fruit with refreshing tastes and is enriched with vitamin E. In the recent years, with the increasing cultivation area of cucumber, it has gradually moved towards a large-scale planting model. However, because cucumber is susceptible to horticultural diseases, including powdery mildew (PM), downy mildew (DM), Fusarium wilt (FW), Verticillium wilt, Cladosporium cucumerinum, Corynespora leaf spot, green mottle mosaic virus, and bacterial soft rot, it does not help for industrialized production, which results in substantial economic losses to cucumber producers. Among the diseases, PM, DM, and FW are the serious main fungal diseases of cucumber that result in severe production and quality losses (Block and Reitsma, 2005; Zhang et al., 2016; Vakalounakis and Lamprou, 2018). Several effective approaches have been widely used to control these diseases, such as various fungicides, biofungicides, and grafting. However, the variable adaptability of pathogens, fungicides residues on plants and in the environment, and higher production costs associated with these approaches indicate that better methods are required (Mahmood et al., 2016; Chen et al., 2021). Therefore, breeding more resistant cultivars is an efficient approach to control cucumber diseases and understanding the genetic and molecular mechanisms of cucumber disease resistance is a crucial focus of cucumber breeding programs.
There is no conclusive genetic data on cucumber disease resistance at present. Some studies have shown that PM, DM, and FW resistance are quantitative traits controlled by multiple genes, respectively. For instance, the resistance to PM is controlled by a recessive single gene, and susceptibility is controlled by partial dominant genes (Nie et al., 2015a). In cucumber, the DM resistance was controlled by multiple recessive genes and has the duplicate recessive epistasis and the additive effects data confirmed the detected 14 quantitative trait loci (QTLs) for DM resistance (Innark et al., 2020). Dong et al. (2019) found that the inheritance of FW resistance in cucumber is a quantitative resistance trait controlled by multiple genes, including two pairs of additive dominance-epistatic major genes and an additive-dominance polygene. However, resistance to PM, DM, and FW is also controlled by a single gene. For example, a single recessive gene, pm, for PM resistance in leaves, has been mapped to an approximately 468 kb region on chromosome 5 in IL52 (Zhang et al., 2018). A recessive resistance gene, dm-1, has been identified in many DM-resistant plant introduction (PI) lines, including PI 197087, Gy4, Chipper, and the Market more series (Barnes and Epps, 1954; Wehner and Shetty, 1997; Call et al., 2012a). Foc has been incorporated in the Dutch-type cucumber hybrids and has widely controlled FW of cucumber for 40 years (Vakalounakis and Fragkiadakis, 2003). Variety is a major factor in the inheritance of cucumber disease resistance. At present, the mapping population of cucumber PM-resistance genes has been mainly constructed using PI 197088, S06, WI 2757, H136, K8, and IL52. In the constructed segregation population, 19 possible PM resistance QTLs were identified (Wang et al., 2020). In the population generated using the high-resistance variety PI 197088, pm1.1, pm1.3, pm4.3, pm5.1, pm5.3, pm5.4, pm6.2, pm6.3, and pm7.1 are the main resistance QTLs and pm4.1 and pm6.3 are two major QTLs in the population constructed using high-resistance variety S06. Additionally, pm5.3 is the most important QTL for PM resistance in the population constructed using the high-resistance cultivar IL52 (Sakata et al., 2006; Liu et al., 2008; Fukino et al., 2013; Yoshioka et al., 2014; Zhang et al., 2018). Most PM-related genes are closely linked to DM-related genes; therefore, they may also play equally important roles in DM resistance (Wang et al., 2018; Zhang et al., 2018). The materials used for mapping genes associated with PM are also used for selecting genes associated with DM. At present, the DM-resistance gene mapping population has mainly been constructed using PI 197085, PI 197088, WI 7120 (PI 330628), WI 2757, S94, TH118FLM, IL52, and K8. In total, 16, 5, and 2 QTLs have been identified in PI 197085, PI 330628, and WI 2757, respectively (Wang et al., 2020). For example, Wang et al. (2018) developed 55 microsatellite markers and found that dm5.1, dm5.2, and dm5.3 are the main resistance QTLs in the highly resistant variety PI 197088 and dm1.1, dm2.1, and dm6.2 are the main sensitivity-related QTLs in the highly susceptible variety Coolgreen. Li et al. (2018) used 141 simple sequence repeat (SSR) markers to identify 5 QTLs, namely, dm1.1, dm3.1, dm4.1, and dm5.1/dm5.2, among which, dm4.1 is a major resistance QTL in the cross-population derived from PI 197088 and Changchunmici. The development of resistance mechanisms against FW occurred more slowly than against PM and DM and few materials resistant to FW have been identified. Dong et al. (2019) detected a major effect QTL, fw2.1, in a 1.91-Mb region on chromosome 2 using F2 segregating populations derived from Superina (P1) and Rijiecheng (P2). Additionally, different identification and evaluation methods, mapping population, infection site, and pathogen races also influence the inheritance of cucumber disease resistance (Vakalounakis and Lamprou, 2018; Innark et al., 2020; Wang et al., 2020). The epigenetic variations also play important roles in crop disease resistance and are affected by environmental factors (Zhi and Chang, 2021), but the epigenetic regulation in cucumber disease resistance has not been found yet.
Many candidate genes for PM, DM, and FW disease resistance in cucumber have been identified using genetic mapping as well as transcriptomic and proteomic analyses and several genes have been cloned for functional verification. The candidate genes for these diseases are all involved in plant hormone signal transduction, cell redox homeostasis, and transcriptional regulation. In PM disease, the Mildew Resistance Locus O (MLO)-like genes, including CsaMLO1–13, but especially CsMLO1, −8, and −11, are the most studied PM genes in cucumber (Schouten et al., 2014; Nie et al., 2015b; Berg et al., 2017) and MLO-based PM resistance caused by the formation of cell wall depositions (papillae) by the plant cell directly beneath the site of PM penetration (Wolter et al., 1993), but the function is not yet unraveled. The candidate genes for DM resistance are involved in various metabolic pathways. For example, STAYGREEN (CsSGR), which is involved in the chlorophyll degradation pathway, plays important roles in DM disease resistance (Wang et al., 2019) and the transient expression of CsLRK10L2, which is a Damage-associated Molecular Pattern Molecule (DAMP) oligogalacturonan receptor and is involved in the breakdown of pectin, in Nicotiana benthamiana (N. benthamiana) leaves causes necrosis and results in high DM resistance (Berg et al., 2020). Several candidate genes of both DM and PM resistance have also been identified. The gene Csa5M622830.1, a GATA transcriptional factor gene, may prevent supplement nutrition from reaching DM and PM pathogens (Zhang et al., 2018). Compared with PM and DM, fewer candidate genes resist to FW have been identified. However, resistance to FW is enhanced in transgenic cucumber harboring Ginkbilobin2-1 (GNK2-1) (Liu et al., 2010). Additionally, a great number of candidate microRNAs (miRNAs), long non-coding RNAs (lncRNAs), proteins, and metabolites related to DM, PM, and FW in cucumber have also been identified (Li et al., 2011; Xu et al., 2019, 2021; Nie et al., 2021; Sun et al., 2021).
Although a number of molecular markers, QTLs, and candidate genes have been identified, the genetic mechanisms of cucumber disease resistance are not well understood. Here, we independently review the genetic mechanisms of cucumber resistance to PM, FW, and DM and also provide new insights into future management strategies.
Inheritance, Quantitative Trait Loci Mapping, and Candidate Genes of Cucumber Resistance to Powdery Mildew
Powdery mildew mainly invades cotyledons, leaves, and stems, resulting in yellow, crisp dry leaves in which photosynthesis is seriously affected, thereby reducing cucumber yield. PM in cucumber is commonly caused by Podosphaera xanthii (Sphaerotheca fuliginea) and Golovinomyces cichoracearum (Erysiphe cichoracearum) (Block and Reitsma, 2005), which share the characteristics of frequent infection, short incubation period, and strong transmission. They also can occur annually during cucumber production.
Inheritance of Powdery Mildew Resistance in Cucumber
A classical genetic analysis demonstrated that cucumber PM resistance is a quantitative trait controlled by multiple recessive genes in different germplasms (Smith, 1948; Kooistra, 1968; Morishita et al., 2003; He et al., 2013). Early in 1948, Smith (1948) suggested that PM resistance in Puerto Rico 37 was controlled by recessive genes and then, associated recessive genes were identified in the PI 2008151 and Natsufushinari varieties (Kooistra, 1968). The two recessively inherited genes linked to the QTL in chromosome 5 are responsible for PM in WI2757 (He et al., 2013). Additionally, studies have shown that PM resistance in cucumber is controlled by a single recessive gene. A single recessive gene pm for PM resistance in leaves has been mapped to an approximately 468 kb region on chromosome 5 in IL52 (Zhang et al., 2018). The resistance to PM in the stem of NCG-12 is also controlled by a single recessive nuclear gene (pm-s) (Liu et al., 2017). The recessive inheritance of PM is not convenient to use in cucumber breeding (Xu X. et al., 2016). The temperature-dependent PM resistance in PI 197088-5 is due to one recessive gene and another incompletely dominant gene (Morishita et al., 2003). Shen et al. (2011) found that cucumber PM traits are determined by the interaction of major genes and polygenes in the JIN 5-508 variety and the inheritance of major genes dominates. Xu X. et al. (2016) first reported the dominantly inherited major-effect QTL (Pm1.1) for PM in the Jin5-508-derived SSSL0.7 line. However, quantitative resistance under polygenic control is generally more durable than that conferred by a single dominant gene (Kelly and Vallejo, 2006). The inheritance of cucumber disease resistance is dependent on the variety and material (Wang et al., 2020) and the genetic laws governing cucumber PM resistance are still not well understood.
Molecular Markers and Quantitative Trait Loci of Powdery Mildew Resistance in Cucumber
Effective molecular markers and QTLs controlling resistance to PM in cucumber have also been reported in recent years (de Ruiter et al., 2008; Fukino et al., 2013; He et al., 2013; Nie et al., 2015a; Wang et al., 2019). Various molecular markers have been used for mapping PM-associated loci in different cucumber species. In total, 140 PM-associated Specific-locus Amplified Fragment Sequencing (SLAFs) and two hot regions (pm5.3 and pm6.1) have been identified on chromosomes 1 and 6 using an F2 segregating population derived from H136 as the susceptible parent and BK2 as the resistance donor (Zhang et al., 2015). In total, 17 SSR markers have been discovered to be linked to the pm-s gene, which maps to chromosome 5 between the pmSSR27 and pmSSR17 markers (Liu et al., 2017). The introgression of the 6.8-Mb segment that contains 3,016 single nucleotide polymorphisms (SNPs) causes the phenotypic variation in PM resistance between SSL508-28 and D8 (Xu et al., 2017) and this region, pm5.1, is consistent with major loci for PM resistance found in many studies (Nie et al., 2015a; Xu Q. et al., 2016; Wang et al., 2018). In total, 113 SNP and InDel markers significantly associated with PM resistance have been identified on chromosomes 4 and 5 using a genome-wide association analysis (GWAS) (Tan, 2021). Additionally, four QTLs (pm1.1, pm2.1, pm5.1, and pm6.1) have been identified on chromosomes 1, 2, 5, and 6 using the recombinant inbred line (RIL) population derived from a cross between PI 197088 and the susceptible line Coolgreen. Among them, pm5.1 is the major-effect QTL, explaining 32.4% phenotypic variance, whereas the minor-effect QTL, pm6.1, contributed to disease susceptibility (Wang et al., 2018). Recently, pm5.2 (30% R2 at LOD 11) and pm6.1 (11% R2 at LOD 3.2) conferred PM resistance in an F2 population derived from a cross between PM-R (resistant) and PM-S (susceptible) (Zhang C. et al., 2021). After further studies on the segregation populations constructed from PI 197088, S06, WI 2757, H136, K8, and IL52, 19 possible QTLs for PM resistance were mapped (Wang et al., 2020). Moreover, PM resistance QTLs are also organ-dependent in cucumber. The disease indices of the hypocotyl, cotyledon, and true leaf of WI2757 were analyzed by multiple QTL mapping. pm5.1 was the major QTL for cotyledon resistance, pm5.2 controlled hypocotyl resistance, pm1.1 and pm1.2 controlled leaf resistance and both the minor QTLs, pm3.1 and pm4.1, caused leaves or hypocotyls to have an increased PM susceptibility (He et al., 2013). Liu et al. (2017) showed that pm-s, located on chromosome 5, controls PM resistance in cucumber stem and the gene Csa5G623470, encoding an MLO protein, is closely related to the PM resistance of stem. Environmental factors also play important roles in the resistance to PM. Sakata et al. (2006) constructed a cucumber RIL using the PI197088-1 variety, resistant to PM, and the Santou variety, susceptible to PM, under both the high and low temperatures. Only one QTL played a role at high (26°C) and low (20°C) temperatures, which suggested that resistance was related to temperature. This was the first study on the QTL mapping of PM resistance genes at different temperatures. Like PM, DM is also an important disease in cucumber production. Many PM QTLs or genes are closely linked to DM QTLs or genes; consequently, they may also play equally important roles in DM resistance (Wang et al., 2018; Zhang et al., 2018). For example, pm2.1, pm5.1, and pm6.1 associated with PM QTLs are colocalized with the DM QTLs dm2.1, dm5.2, and dm6.1, respectively (Wang et al., 2018). These studies showed inconsistent results regarding the number and locations of QTLs underlying PM and this may be due to differences in the germplasms, genetic maps, analysis methods, and environmental conditions.
Candidate Genes or Proteins Involved in the Powdery Mildew Resistance of Cucumber
In the recent years, candidate genes or proteins associated with PM resistance have been identified using transcriptomic and proteomic analyses and genetic mapping. Differentially expressed genes (DEGs) have been identified between PM-resistant species and susceptible species, such as SSL508-28 and D8, XY09-118 and Q10, BK2 and H136, and NILs of S1003 and Near Iso-genic Lines (NIL) (pm5.1), using transcriptomes (Xu et al., 2017; Nie et al., 2021; Zhang P. et al., 2021; Zheng et al., 2021). These DEGs function in plant hormone signal transduction, phenylpropanoid biosynthesis, phenylalanine metabolism, ubiquinone and other terpenoid-quinone biosynthesis, endocytosis, plant–pathogen interaction, and Mitogen-activated Protein Kinases (MAPKS). In particular, genes encoding the transcriptome factors (WRKY, NAC, and TCP), peroxidase, nucleotide-binding site (NBS), glucanase, and chitinase have been analyzed (Zhang P. et al., 2021; Zheng et al., 2021). The miRNAs Csa-miR172c-3p and Csa-miR395a-3p are upregulated in PM-resistant D8 and Csa-miR395d-3p and Csa-miR398b-3p are downregulated in PM-susceptible SSSL508-28, suggesting that their target genes AP2, bHLH, Dof, UGT, and LASPO may play important roles in PM-inoculated cucumber leaves (Xu et al., 2020). Nie et al. (2021) showed that 49 differentially expressed lncRNAs may function as target mimics for 106 miRNAs during cucumber_PM interaction, including miR156, miR159, miR164, miR166, miR169, miR171, miR172, miR6173, miR319, miR390, miR393, miR396, and miR5658. Moreover, differentially regulated processes, proteins, and accumulated metabolites between different PM-resistant materials have also been detected, including flavonoid, hormones, fatty acid, diterpenoid metabolism, tetrapyrrole biosynthetic process, sulfur metabolic process, and cell redox homeostasis (Xu et al., 2019; Zhang P. et al., 2021).
A larger number of potential genes related to PM in cucumber have been identified using genetic mapping. Nie et al. (2015a) delimited the recessive major QTL pm5.1 for PM resistance in an approximately 1.7-kb region between markers UW065021 and UW065094 and they identified an MLO-like gene CsMLO1, which encodes a cell membrane protein, as a candidate gene for PM resistance (Nie et al., 2015b). Schouten et al. (2014) obtained 13 MLO homologs, CsaMLO1-13, in cucumber. Among them, the ectopic expression of CsMLO1 in the PM-resistant Atmlo2-Atmlo12 double-mutant results in PM sensitivity recovery. The overexpression of CsaMLO1 or CsaMLO8 completely restores PM susceptibility in a tomato mlo mutant, whereas the overexpression of CsaMLO11 only partially restores PM susceptibility (Nie et al., 2015b; Berg et al., 2017). To date, only MLO genes in cucumber have been functionally verified as being involved in PM resistance. In addition to MLO genes, other candidate PM resistance genes have been identified. Csa1M064780 and Csa1M064790, encoding a cysteine-rich receptor-like protein kinase, are the most likely candidate PM resistance genes (Xu Q. et al., 2016). The single recessive gene Csa5M622830, which encodes a GATA transcriptional factor, is likely the gene for the complete PM resistance introgressed from Cucumis hystrix (Zhang et al., 2018). CsGy5G015660, which encodes a putative leucine-rich repeat receptor-like serine/threonine-protein kinase, is currently considered a strong candidate gene for PM resistance in cucumber (Liu et al., 2021; Zhang C. et al., 2021). Moreover, proteins related to PM resistance have also been identified and functionally verified. Two NBS-Leucine-rich Repeat (LRR) proteins (CsRSF1 and CsRSF2), closely correlated with Abscisic Acid (ABA) and Gibberellin (GA) signals in cucumber, are predicted to have a similar domain sequence with the Arabidopsis PM-resistance protein RESISTANCE TO POWDERY MILDEW8 (RPW8) (Xiao et al., 2001). The transient silencing of CsRSF1 and CsRSF2 reduces the resistance of cucumber to PM, whereas the transient overexpression of CsRSF1 and CsRSF2 improves the resistance of cucumber to PM (Wang et al., 2021). Transcription factors, such as GRAS, DNA-binding with One Finger (DoF), Eukaryotic Initiation Factor 2 (eIF2α), Polygalacturonase (PG), UDP-Glycosyltransferase (UGT), and Serine/threonine Protein Kinases (STPKs) and their target genes, are also differentially expressed after PM inoculation (Zhong, 2020). Translationally Controlled Tumor Protein (TCTP) is a highly conserved and multifunctional protein and CsTCTP1 may regulate the defense responses of cucumber or ABA signaling to control PM disease in cucumber. CsTCTP2 may regulate the Target of Rapamycin (TOR) signal in response to PM stress (Meng et al., 2018). These studies provide new insights into cucumber responses to PM and the potential genes related to PM will be highly helpful in breeding cucumber varieties with enhanced PM resistance.
Inheritance, Quantitative Trait Loci Mapping, and Candidate Genes of Cucumber Resistance to Downy Mildew
Downy mildew of cucumber is caused by the obligate biotrophic oomycete Pseudoperonospora cubensis. It mainly infects leaves, but can also harm stems and inflorescences. It can occur from seedling to adult stage, but is particularly prevalent when cucumber enters the harvest stage. During the period of seedling infection, irregular chlorotic and withered yellow spots are produced on the reverse sides of cotyledons. A gray-black mold layer is produced when the plant becomes wet and cotyledons die when the infection is serious. During the adult stage, the disease gradually spreads upward from the lower leaves. At the beginning of the disease, light green water-immersion spots appear on the backs of the leaves. At the middle stage of the disease, the leaf spots fade from green to light yellow and the leaf backs become yellowish-brown. At the later stage, the disease spots converge and shrink upward from the leaf edges and finally, the whole leaf withers. In serious cases, all the leaves on the plant die (Zhang et al., 2016).
Inheritance of Downy Mildew Resistance in Cucumber
Researchers have studied the inheritance of cucumber DM resistance. However, due to different resistance germplasms and inconsistent identification methods, there is no consensus on the genetic laws governing cucumber DM resistance. As early as 1942, DM-resistant lines were screened and DM resistance is controlled by a recessive resistance gene, dm-1, in many resistant PI lines, including PI 197087, Gy4, Chipper, and the Marketmore series (Jenkins, 1942; Barnes and Epps, 1954; Wehner and Shetty, 1997; Call et al., 2012a). Simultaneously, multiple recessive genes are also involved in the regulation of cucumber DM resistance in resistant germplasms, including cucumber varieties WI4783, Wisconsin SMR18, K8 and K18, PI19708, CSL0067, and CSL0139 (Doruchowski and Lakowska-Ryk, 1992; Zhang et al., 2013; Szczechura et al., 2015; Wang et al., 2016). Call et al. (2012b) identified three highly DM-resistant materials, PI 197088, PI 330628, and PI 605996, from 1,300 cucumber collections. Among them, PI 197088 is the most studied for DM resistance, with multiple genes being controlled in breeding programs (Li et al., 2018; Liu et al., 2021). PI 197088 also has high resistance to PM. There are different genetic bases of DM-resistant germplasms. Therefore, the identification of DM-associated molecular markers and QTLs in various resistant materials may help to increase the inheritance of DM through breeding programs.
Molecular Markers and Quantitative Trait Loci of Downy Mildew Resistance in Cucumber
A variety of DM-associated QTLs has been identified in different varieties using Sequence Characterized Amplified Regions (SCAR), SSR, and SNP markers in recent years. The genetic linkage map was constructed using 66 polymorphic SSR markers and using this linkage map, 14 QTLs have been detected by evaluating DM in cotyledons as well as first and second true leaves after inoculation. LG5.1, located between the SSR03943 and SSR19172 markers, was detected at all the leaf stages (Innark et al., 2020). Based on the linkage map having 328 SSR and SNP markers, dm4.1, and dm5.1, compared with dm2.1 and dm6.1, were determined to be the major effect of QTL (R2 = 15–30%) with additive effects and this has been reproducibly detected in four environments (US2013, US2014, IT2013, and NL2013) (Wang et al., 2016). In total, five QTLs associated with DM resistance have been identified on chromosomes 1, 3, 4, and 5 in seven independent experiments and dm4.1, explaining 27% of the phenotypic variance, has been reliably detected in all the indoor experiments (Li et al., 2018). The DM candidate QTLs related to DM have been detected using diverse evaluation methods that consist of different plant organs (cotyledons and true leaves), developmental stages (seedlings and adult plants), and evaluation criteria (lesion expansion and sporulation extent) and the dm1.1 QTL has the largest effect on resistance among the nine QTLs detected (Yoshioka et al., 2014). In addition to QTL mapping methods, bulked segregant analyses (BSAs), next-generation sequencing (NGS), and GWASs have been the most rapid and effective ways of studying the genetic inheritance of DM resistant in cucumber. In total, five QTLs (dm2.2, dm4.1, dm5.1, dm5.2, and dm6.1) have been identified and dm2.2 has the largest effect on DM resistance as assessed by combining BSA and NGS methods based on SNP markers (Win et al., 2017). Additionally, 18 QTLs have been detected through the GWAS of a core database of 97 cucumber lines, but only six QTLs (dmG1.4, dmG4.1, dmG4.3, dmG5.2, dmG7.1, and dmG7.2) are associated with stable DM resistance (Liu et al., 2021). To date, PI 197085, PI 197088, WI 7120 (PI 330628), WI 2757, S94, TH118FLM, IL52, and K8 have been used for mapping QTLs associated with DM resistance. Different cucumber germplasm resources may show stable genetic bases and QTLs for DM. For example, dm5.1 and dm5.2 have been detected in five resistance sources (Wang et al., 2020). New QTLs have also been detected in commonly used disease-resistant materials. In PI 197087, Berg et al. (2020) focused on a QTL on chromosome 4-DM4.1 in the NILs produced by PI 197087 and a susceptible cucumber line (HS279) and this contained three sub-QTLs: DM4.1.1 that affects pathogen-induced necrosis, DM4.1.2 that has additive effects on sporulation, and DM4.1.3 that has recessive effects on chlorosis and sporulation. In general, the DM-associated QTLs varied depending on the germplasm and plant tissue as well as the developmental stage used in these analyses.
Candidate Genes or Proteins Involved in the Downy Mildew Resistance of Cucumber
A series of candidate genes or proteins related to DM resistance have been identified in cucumber through transcriptome profiling, proteomic analysis, and fine mapping. A large number of DEGs between DM-resistant and susceptible materials were identified by transcriptome analyses and these DEGs are involved in multiple defense response-related functions, including response: hormone signaling, regulation of nutrient supply, pathogen-associated molecular pattern recognition, signal transduction, reactive oxygen species and lignin accumulation, cell cycle, protein binding and metabolism, and transcriptional regulation (Li et al., 2011; Burkhardt and Day, 2016; Gao et al., 2021). For example, five genes play important roles in the cucumber DM defense pathway: Csa5G139760 encodes an acidic chitin endonuclease, Csa6G080320 encodes a kinase having an LRR domain and transmembrane domain, Csa5G471600 is a retroviral receptor-like protein, and Csa5G544050 and Csa5G564290 encode the RNA-dependent RNA polymerase gene (Gao et al., 2021). Consistently, differentially expressed proteins between the resistant and susceptible cucumber lines have also been identified and most of these proteins focus on cell rescue, defense, and energy metabolism (Sun et al., 2021). Zinc finger-homeodomain (ZHD) proteins encode a family of plant-specific transcription factors that are responsive to DM in cucumber, such as CsZHD1–3, CsZHD6, CsZHD8, and CsZHD10 (Lai et al., 2021). Many novel QTLs for DM resistance in different cucumber species have been detected, such as dm2.1, dm4.1, dm4.1.2, dm4.1.3, dmG2.1, and dmG7.1 (Win et al., 2017; Berg et al., 2020; Liu et al., 2021), and these precise molecular markers and QTLs for DM resistance are helpful for the consequent fine mapping and positional cloning of QTLs. Liu et al. (2021) identified seven DM-resistance candidate genes using GWAS, including Csa1G575030 for dmG1.4, Csa2G060360 for dmG2.1, Csa4G064680 for dmG4.1, Csa5G606470 for dmG5.2, and Csa7G004020 for dmG7.1. Among them, Csa5G606470 is a WRKY transcription factor and it was also identified within the DM-associated QTL dm5.2 using a Bulked Sergeant Analysis with Whole-genome Resequencing (BSA-seq) analysis (Zhang et al., 2018). Cucumber CsSGR encodes a magnesium dechelatase and plays critical regulatory roles in the chlorophyll degradation pathway and a loss-of-susceptibility mutation of CsSGR results in durable broad-spectrum DM disease resistance (Wang et al., 2019). CsLRK10L2 acts as a DAMP oligogalacturonan receptor and is involved in the breakdown of pectin, which is involved in the production of plant cell walls. This gene has been identified as a likely candidate for the sub-QTL DM4.1.2 because the transient expression of its loss-of-function mutation CsLRK10L2 from the DM-susceptible parent HS279 in N. benthamiana leaves causes necrosis (Berg et al., 2020). A series of DM- and PM-associated QTLs were also colocalized in typical Northern Chinese type cucumber K8, PI 197088, and PI 197088-derived line CS-PMR1. For example, dm2.1/pm2.1, dm5.3/pm5.1, and dm6.2/pm6.1 have been colocated in PI 197088 (Wang et al., 2018). Several candidate genes for both the DM and PM resistance have also been identified, including Csa5M622800.1, Csa5M622830.1, and Csa5M623490.1. The gene Csa5M622830.1 is a GATA transcriptional factor gene and it may prevent the nutrition from reaching DM and PM pathogens (Zhang et al., 2018). In addition, Cucumis sativus Irregular Vasculature Patterning (CsIVP)-RNA interference (RNAi) plants having higher salicylic acid levels show higher resistance to DM than wild type (WT) and it was proposed that CsIVP may interact with CsNIMIN1, which is a negative regulator in the salicylic acid-signaling pathway, to improve DM resistance in cucumber (Yan et al., 2020). At present, the candidate genes for DM resistance in cucumber identified by forward genetic analysis methods need to be verified by overexpression or knockout experiments in cucumber.
Inheritance, Quantitative Trait Loci Mapping, and Candidate Genes of Cucumber Resistance to Fusarium Wilt
Cucumber FW, caused by Fusarium oxysporum f. sp. cucumerinum Owen (FOC), is a systemic soil-borne fungal disease and the hyphae of this pathogen penetrate cucumber roots, which causes vascular wilt. The disease causes necrotic lesions on the stem bases, foliar wilting, and eventually whole-plant wilt and even death and it occurs throughout cucumber development (Vakalounakis and Lamprou, 2018). The main factor affecting the incidence of FW is the number of FOC in the soil, which is positively correlated.
Inheritance of Fusarium Wilt Resistance in Cucumber
To understand the genetic inheritance of FW resistance, it is important to develop resistance breeding resources and breed-resistant varieties. The inheritance of FW resistance in cucumber has been studied for a long time, but with different conclusions (Toshimitsu and Noguchi, 1975; Netzer et al., 1977; Vakalounakis, 1993, 1995; Zhang et al., 2014; Vakalounakis and Lamprou, 2018; Dong et al., 2019; Jaber et al., 2020). Dong et al. (2019) found that the inheritance of FW resistance in cucumber is a quantitative trait controlled by multiple genes using an F2 population derived from a cross between the susceptible line Superina and the resistant line Rijiecheng and several studies agreed with this inheritance of FW resistance in cucumber (Toshimitsu and Noguchi, 1975; Zhang et al., 2014). Other researchers have reported that the FW resistance in cucumber is a qualitative trait controlled by a single Foc gene (Netzer et al., 1977; Vakalounakis, 1993, 1995; Vakalounakis and Lamprou, 2018; Jaber et al., 2020). The Foc gene has been incorporated in the Dutch-type cucumber hybrids and has widely controlled FW in cucumber for 40 years (Vakalounakis and Fragkiadakis, 2003). The different patterns of FW inheritance in cucumber are also influenced by pathogen races, including races 1–3 from America, Israel, and Japan, respectively, and race 4 from China (Zhang et al., 2014). Vakalounakis and Lamprou (2018) found that Foc (syn.Fcu-1), which has been identified as a dominant FW resistance gene in the cultivars SMR-18 and WIS2757, controls FW resistance to races 1, 2, and 3, which indicates that FW resistance is not related to different pathogen races. Additionally, the Foc gene was found to be linked to the Ccu gene, which controls resistance to scab in cucumber inbred line 9110Gt, possible due to the FW and scab resistance in cucumber both being controlled by an NBS-type R gene (Vakalounakis, 1993; Mao et al., 2008). In the future, the availability of more natural FW-resistant resources aids in revealing the inheritance pattern of FW resistance in cucumber.
Molecular Markers and Quantitative Trait Loci of Fusarium Wilt Resistance in Cucumber
Compared with PM and DM, there are limited reports on molecular linkage markers and QTL mapping related to the inheritance of FW resistance in cucumber. Wang (2005) identified an Amplified Fragment Length Polymorphisms (AFLP) marker E25M70 and an SSR marker CSWCT06A linked to cucumber Foc2.1 at genetic distances of 8.12 and 5.98 cM, respectively. One major QTL, Foc2.1, has been screened from the F9 RILs derived from the cross between 9110Gt and 9930 and it is located between SSR03084 and SSR17631 on chromosome 2. The marker SSR17631 has been validated with an 87.88% accuracy among 46 cucumber germplasms (Zhang et al., 2014). Moreover, Zhou et al. (2015) mapped the QTL of Foc4 resistance to FW in the region of SSR17631 and SSR00684 on chromosome 2. Another major QTL, fw2.1, located on chromosome 2, has also been detected and fine-mapped, with a physical distance of 0.60 Mb (InDel1248093–InDel1817308) and it contains 80 candidate genes (Dong et al., 2019). One AFLP marker of FW resistance in cucumber has been identified at a distance of 6.0 cm from the Foc gene and it was converted into SCE12M50B and SCE12M50A codominant markers (Jaber et al., 2020). The SCE12M50B marker is located 7.0 cm away from SSR03084 and is linked to the Ccu locus that controls resistance to scab in cultivar SMR-18 (Mao et al., 2008; Jaber et al., 2020). Owing to the complexity of FW symptoms and the defects of related research techniques, the mechanisms and functions of these loci have not been determined and require further exploration.
Candidate Genes or Proteins Involved in the Fusarium Wilt Resistance of Cucumber
Some FW candidate proteins and genes in cucumber have been identified using proteomic and transcriptomic analyses in different FW-resistant varieties. A comparative proteomic analysis of root proteins isolated from infected highly susceptible 995 and highly resistant F9 revealed that 15 overaccumulated proteins are mainly involved in defense and stress responses, oxidation-reduction, metabolism, transport and other processes, and jasmonic acid and redox signaling components. LRR family- and stress-related proteins may be crucial in the defense responses to FW in cucumber (Zhang et al., 2016). Moreover, defense mechanisms against oxidation and detoxification as well as carbohydrate metabolism may also be necessary for FW resistance in cucumber (Du et al., 2016). Xu et al. (2021) identified 210 and 243 differentially regulated proteins in the FW resistance Rijiecheng and high-susceptibility Superina after Foc infection. Additionally, four genes, TMEM115 (CsaV3_5G025750), which encodes a transmembrane protein, TET8 (CsaV3_2G007840), which functions as a tetraspanin, TPS10 (CsaV3_2G017980), which encodes a terpene synthase, and MGT2 (CsaV3_7G006660), which encodes a glycosyltransferase, are remarkably upregulated in both the cultivars after Foc inoculation, but with higher expression levels in Superina. In total, 14 chitinase defense-related genes have higher expression levels in FW susceptible and resistant lines and CsChi23 may play an important role in activating a rapid immune reaction against FW (Bartholomew et al., 2019). Furthermore, other defense-related genes are activated to regulate the defense responses of cucumber to a Foc inoculation, including several genes related to ABA and ethylene (Zhou and Wu, 2009; Dong et al., 2020). miR319a-JRL3, miR6300-BEE1, miR6300-DAHP1, and miR6300-PERK2 also regulate cucumber defenses against FW (Xu et al., 2021). Dong et al. (2019) identified five candidate FW-resistance genes in fw2.1 by combining genetic mapping and a transcriptome analysis, Csa2G007990, which encodes calmodulin, Csa2G009430, which encodes a transmembrane protein, Csa2G009440, which encodes a serine-rich protein, and Csa2G008780 and Csa2G009330, which are novel genes. This is the only report of mapping FW candidate genes in cucumber, but the functions of the candidate genes have not been verified.
Future Prospects for Enhancing Cucumber Disease Resistance
In summary, the inheritance of PM, DM, and FW resistance in cucumber has been widely investigated and cucumber resistance traits are generally considered as quantitative traits controlled by more one gene. Because of the complicated inheritance of resistance to cucumber diseases, the results are not unified. Simultaneously, several molecular markers and QTLs for PM, DM, and FW resistance in cucumber have been identified (Figure 1 and Supplementary Table 1). Many factors affect cucumber resistance to these three diseases, including pathogen species, plant materials, pathogen invasion site, environment, and genetic linkage, resulting in a variety of effective molecular markers and QTLs for cucumber disease. Large numbers of candidate genes and immune proteins associated with DM, PM, and FW have been identified using mapping, GWAS, RNA sequencing (RNA-seq), and proteomic assay technology, but only a few have been functionally verified (Figure 1 and Supplementary Table 1). For example, only the functions of MLO-like genes that are important in PM resistance have been verified in cucumber. The transient silencing of the two NBS-LRR genes (CsRSF1 and CsRSF2) reduces cucumber resistance to PM. Among the DM-resistant candidate genes, CsSGR, CsLRK10L2, and CsIVP have been functionally verified through mutation, transient expression, or RNAi. Additionally, the resistance of GNK2-1 transgenic cucumber to FW is enhanced compared with WT (Liu et al., 2010). Because of a lack of cucumber FW-resistant germplasms in China, the susceptibility of most cultivars, and the relatively narrow genetic variation among cucumber FW, the breeding of cucumber FW-resistant cultivars has been restricted to a certain extent and research on the molecular mechanisms of FW has not progressed as far as research on PM and DW.
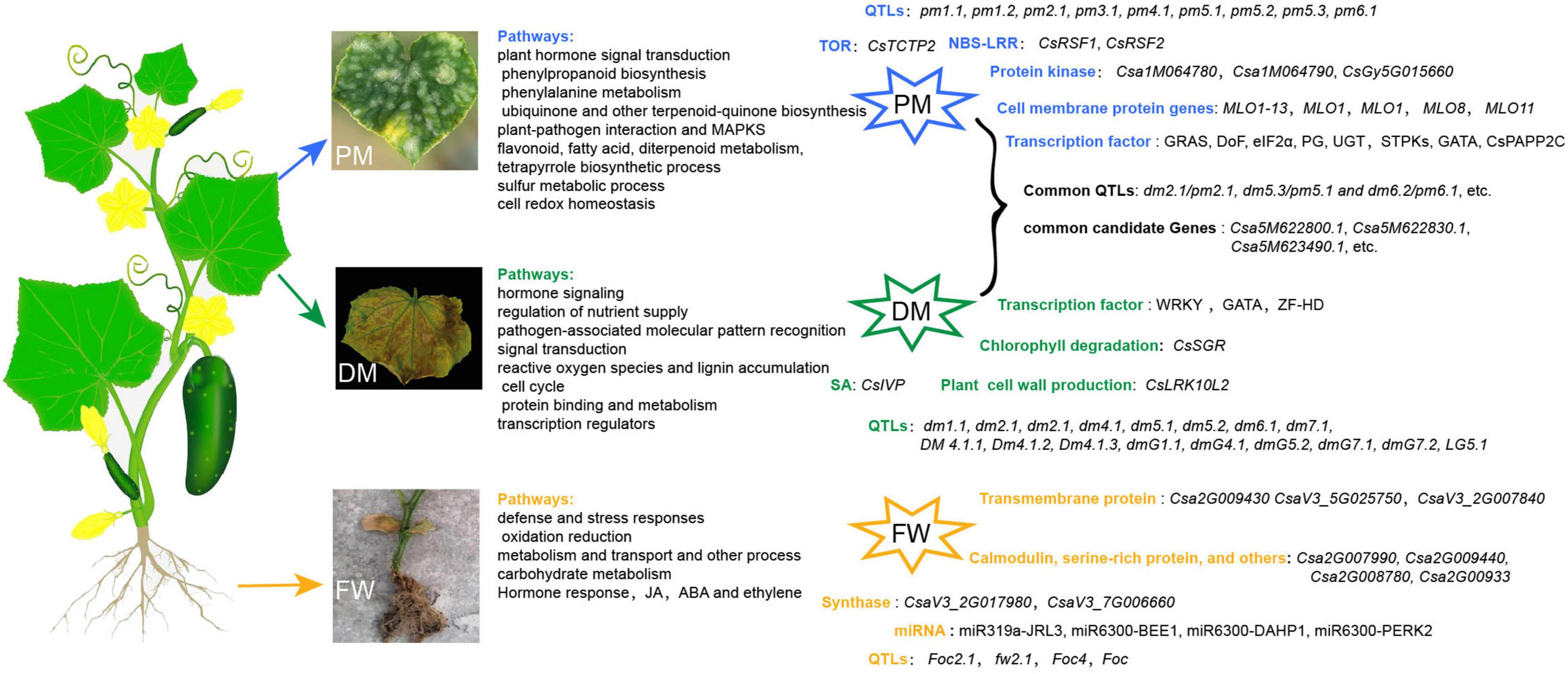
Figure 1. The symptoms, QTL mapping and candidate genes or proteins related to cucumber PM, DM, and FW, respectively.
To better effectively prevent cucumber diseases and explore the genetic and molecular mechanisms of cucumber resistance to PM, DM, and FW, respectively, we propose five aspects of work that need to be performed in the future: (1) collect more disease-resistant cucumber germplasms, especially materials that are resistant to multiple pathogens, including wild germplasm resources, cultivars, and mutants; (2) identify more effective molecular markers and QTLs associated with PM, DM, and FW to be used in selecting germplasms and accelerating resistance breeding; (3) analyze more differently expressed DNA, RNA, miRNAs, lncRNAs, or metabolic related to PM, DM, or FW, respectively, through omics or multiomics and bioinformatics tools would provide considerable experimental information for mechanistic investigations and understand the regulatory network for cucumber diseases, such as transcriptomics, proteomics, metabolomics, epigenomics, and interactomics. Additionally, the data-driven interface through a user-friendly web interface would also be helpful for the mechanism of cucumber diseases, such as plant regulomics (Ran et al., 2020); (4) improve the efficiency and stability of genetic transformation in cucumber. There are now effective methods for gene functional verification that use biotechnology, such as transgenes, RNAi, Transcription Activator-like Effector Nucleases (TALENs), and CRISPR-Cas; (5) develop persistent and safe preventive measures, including chemical, biological, and physical controls. For example, maintaining an optimization of blue light in the growth light before nighttime UV is important for the management of PW in cucumber (Palma et al., 2021). A balance between effective defense and crops yield should be established through these preventive measures. The plant immunity engineering toolbox that integrates genetics, technology, and engineering is required for enhancing disease resistance in crops in the future and the molecular mechanisms of cucumber resistance to PM, DM, and FW need to be further studied.
Author Contributions
YH, MW, and YY drafted the manuscript. CY, SC, and YS modified the manuscript. LM, HW, XZ, and LW designed the project and gave suggestions on the revision of the manuscript. All the authors approved the final version of the manuscript.
Funding
This study was supported by “Pioneer” and “Leading Goose” R&D Program of Zhejiang (No. 2022C02051), the Zhejiang Natural Science Foundation of China (Grant No. LY19C150008), Opening Project Fund of Key Laboratory of Biology and Genetic Resources of Rubber Tree, Ministry of Agriculture and Rural Affairs, PR China/State Key Laboratory Breeding Base of Cultivation and Physiology for Tropical Crops/Danzhou Investigation and Experiment Station of Tropical Crops, Ministry of Agriculture and Rural Affairs, PR China (No. RRI-KLOF202102), the Natural Science Foundation of Zhejiang province (Grant Nos. LY21C150002 and LQY19C150001), the National Natural Science Foundation of China (Grant Nos. 31872105, 31972221, 32002048, 31801862, and 32172595), the National College Students Innovation and Entrepreneurship Training Program in 2019 and 2021 (Nos. 202110341043 and 201910341005), and the Student Scientific research training program of Zhejiang Agriculture and Forestry University (Nos. 2021KX0196 and 2021KX019), Ministry of Agriculture, and the National Key Research and Development Program of China (Nos. 2018YFD1000800 and 2019YFD1000300).
Conflict of Interest
The authors declare that the research was conducted in the absence of any commercial or financial relationships that could be construed as a potential conflict of interest.
Publisher’s Note
All claims expressed in this article are solely those of the authors and do not necessarily represent those of their affiliated organizations, or those of the publisher, the editors and the reviewers. Any product that may be evaluated in this article, or claim that may be made by its manufacturer, is not guaranteed or endorsed by the publisher.
Supplementary Material
The Supplementary Material for this article can be found online at: https://www.frontiersin.org/articles/10.3389/fpls.2022.862486/full#supplementary-material
References
Barnes, W. C., and Epps, W. M. (1954). An unreported type of resistance to cucumber downy mildew. Plant Dis. Rep. 38:620.
Bartholomew, E. S., Black, K., Feng, Z., Wan, L., Nan, S., Xiao, Z., et al. (2019). Comprehensive analysis of the chitinase gene family in cucumber (Cucumis sativus L.): from gene identification and evolution to expression in response to Fusarium oxysporum. Int. J. Mol. Sci. 20:5309. doi: 10.3390/ijms20215309
Berg, J. A., Appiano, M., Bijsterbosch, G., Richard, G. F. V., Henk, J. S., and Yuling, B. (2017). Functional characterization of cucumber (Cucumis sativus L.) Clade V MLO genes. BMC Plant Biol. 17:80. doi: 10.1186/s12870-017-1029-z
Berg, J. A., Hermans, F. W., Beenders, F., Lou, L., Vriezen, W. H., Visser, R. G., et al. (2020). Analysis of QTL DM4. 1 for downy mildew resistance in cucumber reveals multiple subQTL: a novel RLK as candidate gene for the most important subQTL. Front. Plant Sci. 11:1601. doi: 10.3389/fpls.2020.569876
Block, C. C., and Reitsma, K. R. (2005). Powdery mildew resistance in the US national plant germplasm system cucumber collection. HortScience 40, 416–420. doi: 10.21273/hortsci.40.2.416
Burkhardt, A., and Day, B. (2016). Transcriptome and small RNAome dynamics during a resistant and susceptible interaction between cucumber and downy mildew. Plant Genome 9:plantgenome2015.08.0069. doi: 10.3835/plantgenome2015.08.0069
Call, A. D., Criswell, A. D., Wehner, T. C., Ando, K., and Grumet, R. (2012a). Resistance of cucumber cultivars to a new strain of cucurbit downy mildew. HortScience 47, 171–178. doi: 10.21273/hortsci.47.2.171
Call, A. D., Criswell, A. D., Wehner, T. C., Klosinska, U., and Kozik, E. U. (2012b). Screening cucumber for resistance to downy mildew caused by Pseudoperonospora cubensis (Berk. and Curt.) Rostov. Crop Sci. 52, 577–592. doi: 10.2135/cropsci2011.06.0296
Chen, Q., Yu, G., Wang, X., Meng, X., and Lv, C. (2021). Genetics and resistance mechanism of the cucumber (Cucumis sativus L.) against powdery mildew. J. Plant Growth Regul. 40, 147–153. doi: 10.1007/s00344-020-10075-7
Dong, J., Wang, Y., Xian, Q., Chen, X., and Xu, J. (2020). Transcriptome analysis reveals ethylene-mediated defense responses to Fusarium oxysporum f. sp. cucumerinum infection in Cucumis sativus L. BMC Plant Biol. 20:334. doi: 10.1186/s12870-020-02537-7
Dong, J., Xu, J., Xu, X., Xu, Q., and Chen, X. (2019). Inheritance and quantitative trait locus mapping of Fusarium wilt resistance in cucumber. Front. Plant Sci. 10:1425. doi: 10.3389/fpls.2019.01425
Doruchowski, R. W., and Lakowska-Ryk, E. (1992). “Inheritance of resistance to downy mildew (Pseudoperonospora cubensis Berk & Curt) in Cucumis sativus,” in Proceedings of the 5th EUCARPIA Cucurbitaceae Symposiyum, 66–69. doi: 10.1007/BF00035865
Du, N., Shi, L., Yuan, Y., Li, B., Shu, S., Sun, J., et al. (2016). Proteomic analysis reveals the positive roles of the plant-growth-promoting rhizobacterium NSY50 in the response of cucumber roots to Fusarium oxysporum f. sp. cucumerinum inoculation. Front. Plant Sci. 7:1859. doi: 10.3389/fpls.2016.01859
Fukino, N., Yoshioka, Y., Sugiyama, M., Sakata, Y., and Matsumoto, S. (2013). Identification and validation of powdery mildew (Podosphaera xanthii)-resistant loci in recombinant inbred lines of cucumber (Cucumis sativus L.). Mol. Breed. 32, 267–277. doi: 10.1007/s11032-013-9867-3
Gao, X., Guo, P., Wang, Z., Chen, C., and Ren, Z. (2021). Transcriptome profiling reveals response genes for downy mildew resistance in cucumber. Planta 253:112. doi: 10.1007/s00425-021-03603-6
He, X., Li, Y., Pandey, S., Yandell, B. S., Pathak, M., and Weng, Y. (2013). QTL mapping of powdery mildew resistance in WI 2757 cucumber (Cucumis sativus L.). Theor. Appl. Genet. 126, 2149–2161. doi: 10.1007/s00122-013-2125-6
Innark, P., Panyanitikoon, H., Khanobdee, C., Samipak, S., and Jantasuriyarat, C. (2020). QTL identification for downy mildew resistance in cucumber using genetic linkage map based on SSR markers. J. Genet. Genomics 99:81. doi: 10.1007/s12041-020-01242-6
Jaber, E. H. A., Srour, A. Y., Zambounis, A. G., Vakalounakis, D. J., and Doulis, A. G. (2020). Identification of SCAR markers linked to the Foc gene governing resistance to Fusarium oxysporum f. sp. cucumerinum in cucumber cv. SMR-18. Eur. J. Plant Pathol. 157, 845–855. doi: 10.1007/s10658-020-02045-2
Jenkins, J. M. Jr. (1942). Downy mildew resistance in cucumbers. J. Hered. 33, 35–38. doi: 10.1093/oxfordjournals.jhered.a105122
Kelly, J. D., and Vallejo, V. (2006). “QTL analysis of multigenic disease resistance in plant breeding,” in Multigenic and Induced Systemic Resistance in Plants, eds S. Tuzan and E. Bent (Boston, MA: Springer), 21–48. doi: 10.1007/0-387-23266-4_3
Kooistra, E. (1968). Powdery mildew resistance in cucumber. Euphytica 17, 236–244. doi: 10.1007/BF00021216
Lai, W., Zhu, C., Hu, Z., Liu, S., Wu, H., and Zhou, Y. (2021). Identification and transcriptional analysis of Zinc Finger-Homeodomain (ZF-HD) family genes in cucumber. Biochem. Genet. 59, 884–901. doi: 10.1007/s10528-021-10036-z
Li, J. W., Liu, J., Zhang, H., and Xie, C. H. (2011). Identification and transcriptional profiling of differentially expressed genes associated with resistance to Pseudoperonospora cubensis in cucumber. Plant Cell Rep. 30, 345–357. doi: 10.1007/s00299-010-0959-9
Li, L., He, H., Zou, Z., and Li, Y. (2018). QTL analysis for downy mildew resistance in cucumber inbred line PI 197088. Plant Dis. 102, 1240–1245. doi: 10.1094/PDIS-04-17-0491-RE
Liu, J., Tian, H., Wang, Y., and Guo, A. (2010). Overexpression of a novel antifungal protein gene GNK2-1 results in elevated resistance of transgenic cucumber to Fusarium oxysporum. Chin. Bull. Bot. 45:411. doi: 10.3969/j.issn.1674-3466.2010.04.003
Liu, L., Cai, R., Yuan, X., He, H., and Pan, J. (2008). QTL molecular marker location of powdery mildew resistance in cucumber (Cucumis sativus L.). Sci. China Life Sci. 51, 1003–1008. doi: 10.1007/s11427-008-0110-0
Liu, P., Miao, H., Lu, H., Cui, J., Tian, G., Wehner, T. C., et al. (2017). Molecular mapping and candidate gene analysis for resistance to powdery mildew in Cucumis sativus stem. Genet. Mol. Res. 16:gmr16039680. doi: 10.4238/gmr16039680
Liu, X., Gu, X., Lu, H., Liu, P., Miao, H., Bai, Y., et al. (2021). Identification of novel loci and candidate genes for resistance to powdery mildew in a resequenced cucumber germplasm. Genes 12:584. doi: 10.3390/genes12040584
Mahmood, I., Imadi, S. R., Shazadi, K., Gul, A., and Hakeem, K. R. (2016). “Effects of pesticides on environment,” in Plant, Soil and Microbes, eds K. Hakeem, M. Akhtar, and S. Abdullah (Cham: Springer), 253–269. doi: 10.1007/978-3-319-27455-3_13
Mao, A., Zhang, Z., and Zhang, L. (2008). Analysis on the inheritance of resistance to fusarium wilt race 4 and cucumber scab and their linkage in cucumber WLS2757. Zhongguo Nong Ye Ke Xue 41, 3382–3388. doi: 10.3864/j.issn.0578-1752.2008.10.062
Meng, X., Yu, Y., Zhao, J., Cui, N., Song, T., Yang, Y., et al. (2018). The two translationally controlled tumor protein genes, CsTCTP1 and CsTCTP2, are negative modulators in the Cucumis sativus defense response to Sphaerotheca fuliginea. Front. Plant Sci. 9:544. doi: 10.3389/fpls.2018.00544
Morishita, M., Sugiyama, K., Saito, T., and Sakata, Y. (2003). Powdery mildew resistance in cucumber. Jpn. Agric. Res. Q. 37, 7–14. doi: 10.6090/jarq.37.7
Netzer, D., Niego, S., and Galun, E. (1977). A dominant gene conferring resistance to Fusarium wilt in cucumber. Phytopathology 67, 525–527. doi: 10.1094/Phyto-67-525
Nie, J., He, H., Peng, J., Yang, X., Bie, B., Zhao, J., et al. (2015a). Identification and fine mapping of pm5. 1: a recessive gene for powdery mildew resistance in cucumber (Cucumis sativus L.). Mol. Breed. 35:7. doi: 10.1007/s11032-015-0206-8
Nie, J., Wang, Y., He, H., Guo, C., Zhu, W., Pan, J., et al. (2015b). Loss-of-function mutations in CsMLO1 confer durable powdery mildew resistance in cucumber (Cucumis sativus L.). Front. Plant Sci. 6:1155. doi: 10.3389/fpls.2015.01155
Nie, J., Wang, H., Zhang, W., Teng, X., Yu, C., Cai, R., et al. (2021). Characterization of lncRNAs and mRNAs involved in powdery mildew resistance in cucumber. Phytopathology® 111, 1613–1624. doi: 10.1094/PHYTO-11-20-0521-R
Palma, C. F. F., Castro-Alves, V., Morales, L. O., Rosenqvist, E., Ottosen, C. O., and Strid, Å (2021). Spectral composition of light affects sensitivity to UV-B and photoinhibition in cucumber. Front. Plant Sci. 11:610011. doi: 10.3389/fpls.2020.610011
Ran, X., Zhao, F., Wang, Y., Liu, J., Zhuang, Y., Ye, L., et al. (2020). Plant regulomics: a data-driven interface for retrieving upstream regulators from plant multi-omics data. Plant J. 101, 237–248. doi: 10.1111/tpj.14526
Ruiter, W. D., Hofstede, R., Vries, J. D., and Heuvel, H. (2008). “Combining QTLs for resistance to CYSDV and powdery mildew in a single cucumber line. In Cucurbitaceae 2008,” in Proceedings of the IXth EUCARPIA Meeting on Genetics and Breeding of Cucurbitaceae, Avignon, France, 21-24 May 2008, (Paris: Institut National de la Recherche Agronomique (INRA)), 181–188.
Sakata, Y., Kubo, N., Morishita, M., Kitadani, E., Sugiyama, M., and Hirai, M. (2006). QTL analysis of powdery mildew resistance in cucumber (Cucumis sativus L.). Theor. Appl. Genet. 112, 243–250. doi: 10.1007/s00122-005-0121-1
Schouten, H. J., Krauskopf, J., Visser, R. G., and Bai, Y. (2014). Identification of candidate genes required for susceptibility to powdery or downy mildew in cucumber. Euphytica 200, 475–486. doi: 10.1007/s10681-014-1216-z
Shen, L., Xu, Q., Qi, X., and Chen, X. (2011). Genetic model analysis of cucumber powdery mildew resistance. J. Jiangsu Agric. 27, 361–365.
Sun, C., Song, X., Zheng, J., Li, X., Feng, Z., and Yan, L. (2021). Comparative proteomic profiles of resistant/susceptible cucumber leaves in response to downy mildew infection. Hortic. Plant J. 7, 327–340. doi: 10.1016/j.hpj.2021.01.008
Szczechura, W., Staniaszek, M., Klosinska, U., and Kozik, E. U. (2015). Molecular analysis of new sources of resistance to Pseudoperonospora cubensis (Berk. et Curt.) Rostovzev in cucumber. Russ. J. Genet. 51, 974–979. doi: 10.7868/s0016675815090118
Tan, M. (2021). Cucumber Powdery Mildew Resistance Key Genes Identification Based on Genome-Wide Association Analysis. Yangzhou: Yangzhou University. doi: 10.27441/d.cnki.gyzdu.2021.001588
Toshimitsu, Y., and Noguchi, T. (1975). “Inheritance of resistance to Fusarium wilt in cucumber,” in Proceedings of the Meeting of the Japanese Horticultural Society, 150–151.
Vakalounakis, D. J. (1993). Inheritance and genetic linkage of fusarium wilt {Fusarium oxysporum f. sp. cucumerinum race 1) and scab {Cladosporium cucumerinum) resistance genes in cucumber (Cucumis sativus). Ann. Appl. Biol. 123, 359–365. doi: 10.1111/j.1744-7348.1993.tb04098.x
Vakalounakis, D. J. (1995). Inheritance and linkage of resistance in cucumber line SMR-18 to races 1 and 2 of Fusarium oxysporum f. sp. cucumerinum. Plant Pathol. 44, 169–172.
Vakalounakis, D. J., and Fragkiadakis, G. A. (2003). Plant Pathobreeding with Emphasis in Tomato and Cucurbits, ed. D. J. Vakalounakis (Heraklio).
Vakalounakis, D. J., and Lamprou, K. (2018). The Foc gene governs resistance to race 3 of Fusarium oxysporum f. sp. cucumerinum in the cucumber cv. SMR-18. Eur. J. Plant Pathol. 152, 653–656. doi: 10.1007/s10658-018-1508-6
Wang, X., Chen, Q., Huang, J., Meng, X., Cui, N., Yu, Y., et al. (2021). Nucleotide-binding leucine-rich repeat genes CsRSF1 and CsRSF2are positive modulators in the Cucumis sativusdefense response to Sphaerotheca fuliginea. Int. J. Mol. Sci. 22:3986. doi: 10.3390/ijms22083986
Wang, Y. (2005). Molecular Markers of Fusarium Wilt Resistance-Related Genes in Cucumber (Cucumis sativus L.). Yangling: Northwest A & F University.
Wang, Y., Bo, K., Gu, X., Pan, J., Li, Y., Chen, J., et al. (2020). Molecularly tagged genes and quantitative trait loci in cucumber with recommendations for QTL nomenclature. Hort. Res. 7:3. doi: 10.1038/s41438-019-0226-3
Wang, Y., Tan, J., Wu, Z., VandenLangenberg, K., Wehner, T. C., Wen, C., et al. (2019). STAYGREEN, STAY HEALTHY: a loss-of-susceptibility mutation in the STAYGREEN gene provides durable, broad-spectrum disease resistances for over 50 years of US cucumber production. New Phytol. 221, 415–430. doi: 10.1111/nph.15353
Wang, Y., VandenLangenberg, K., Wehner, T. C., Kraan, P. A., Suelmann, J., Zheng, X., et al. (2016). QTL mapping for downy mildew resistance in cucumber inbred line WI7120 (PI 330628). Theor. Appl. Genet. 129, 1493–1505. doi: 10.1007/s00122-016-2719-x
Wang, Y., VandenLangenberg, K., Wen, C., Wehner, T. C., and Weng, Y. (2018). QTL mapping of downy and powdery mildew resistances in PI 197088 cucumber with genotyping-by-sequencing in RIL population. Theor. Appl. Genet. 131, 597–611. doi: 10.1007/s00122-017-3022-1
Wehner, T. C., and Shetty, N. V. (1997). Downy mildew resistance of the cucumber germplasm collection in North Carolina field tests. HortScience 32:450. doi: 10.2135/cropsci1997.0011183X003700040050x
Win, K. T., Vegas, J., Zhang, C., Song, K., and Lee, S. (2017). QTL mapping for downy mildew resistance in cucumber via bulked segregant analysis using next-generation sequencing and conventional methods. Theor. Appl. Genet. 130, 199–211. doi: 10.1007/s00122-016-2806-z
Wolter, M., Hollricher, K., Salamini, F., and Schulze-Lefert, P. (1993). The mlo resistance alleles to powdery mildew infection in barley trigger a developmentally controlled defence mimic phenotype. Mol Gen Genet. 239, 122–128. doi: 10.1007/BF00281610
Xiao, S., Ellwood, S., Calis, O., Patrick, E., Li, T., Coleman, M., et al. (2001). Broad spectrum mildew resistance in Arabidopsis thaliana mediated by RPW8. Science 291, 118–120. doi: 10.1126/science.291.5501.118
Xu, J., Wang, K., Xian, Q., Zhang, N., Dong, J., and Chen, X. (2021). Identification of susceptibility genes for Fusarium oxysporum in cucumber via comparative proteomic analysis. Genes 12:1781. doi: 10.3390/genes12111781
Xu, Q., Shi, Y., Yu, T., Xu, X., Yan, Y., Qi, X., et al. (2016). Whole-genome resequencing of a cucumber chromosome segment substitution line and its recurrent parent to identify candidate genes governing powdery mildew resistance. PLoS One 11:e0164469. doi: 10.1371/journal.pone.0164469
Xu, Q., Xu, X., Shi, Y., Qi, X., and Chen, X. (2017). Elucidation of the molecular responses of a cucumber segment substitution line carrying Pm5. 1 and its recurrent parent triggered by powdery mildew by comparative transcriptome profiling. BMC Genom. 18:21. doi: 10.1186/s12864-016-3438-z
Xu, X., Liu, X., Yan, Y., Wang, W., Gebretsadik, K., Qi, X., et al. (2019). Comparative proteomic analysis of cucumber powdery mildew resistance between a single-segment substitution line and its recurrent parent. Hort. Res. 6:115. doi: 10.1038/s41438-019-0198-3
Xu, X., Yu, T., Xu, R., Shi, Y., Lin, X., Xu, Q., et al. (2016). Fine mapping of a dominantly inherited powdery mildew resistance major-effect QTL, Pm1. 1, in cucumber identifies a 41.1 kb region containing two tandemly arrayed cysteine-rich receptor-like protein kinase genes. Theor. Appl. Genet. 129, 507–516. doi: 10.1007/s00122-015-2644-4
Xu, X., Zhong, C., Tan, M., Song, Y., Qi, X., Xu, Q., et al. (2020). Identification of MicroRNAs and their targets that respond to powdery mildew infection in cucumber by small RNA and degradome sequencing. Front. Genet. 11:246. doi: 10.3389/fgene.2020.00246
Yan, S., Ning, K., Wang, Z., Liu, X., Zhong, Y., Ding, L., et al. (2020). CsIVP functions in vasculature development and downy mildew resistance in cucumber. PLoS Biol. 18:e3000671. doi: 10.1371/journal.pbio.3000671
Yoshioka, Y., Sakata, Y., Sugiyama, M., and Fukino, N. (2014). Identification of quantitative trait loci for downy mildew resistance in cucumber (Cucumis sativus L.). Euphytica 198, 265–276. doi: 10.1007/s10681-014-1102-8
Zhang, C., Anarjan, M. B., Win, K. T., Begum, S., and Lee, S. (2021). QTL-seq analysis of powdery mildew resistance in a Korean cucumber inbred line. Theor. Appl. Genet. 134, 435–451. doi: 10.1007/s00122-020-03705-x
Zhang, D., Meng, K., Hao, Y., Fan, H., Cui, N., Wang, S., et al. (2016). Comparative proteomic analysis of cucumber roots infected by Fusarium oxysporum f. sp. cucumerium Owen. Physiol. Mol. Plant Pathol. 96, 77–84. doi: 10.1016/j.pmpp.2016.09.002
Zhang, K., Wang, X., Zhu, W., Qin, X., Xu, J., Cheng, C., et al. (2018). Complete resistance to powdery mildew and partial resistance to downy mildew in a Cucumis hystrix introgression line of cucumber were controlled by a co-localized locus. Theor. Appl. Genet. 131, 2229–2243. doi: 10.1007/s00122-018-3150-2
Zhang, P., Zhu, Y., and Zhou, S. (2021). Comparative analysis of powdery mildew resistant and susceptible cultivated cucumber (Cucumis sativus L.) varieties to reveal the metabolic responses to Sphaerotheca fuliginea infection. BMC Plant Biol. 21:24. doi: 10.1186/s12870-020-02797-3
Zhang, S., Liu, M., Miao, H., Zhang, S., Yang, Y., Xie, B., et al. (2013). Chromosomal mapping and QTL analysis of resistance to downy mildew in Cucumis sativus. Plant Dis. 97, 245–251. doi: 10.1094/PDIS-11-11-0941-RE
Zhang, S., Miao, H., Yang, Y., Xie, B., Wang, Y., and Gu, X. (2014). A major quantitative trait locus conferring resistance to Fusarium wilt was detected in cucumber by using recombinant inbred lines. Mol. Breed. 34, 1805–1815. doi: 10.1007/s11032-014-0140-1
Zhang, Z., Mao, L., Chen, H., Bu, F., Li, G., and Sun, J. (2015). Genome-wide mapping of structural variations reveals a copy number variant that determines reproductive morphology in cucumber. Plant Cell 27, 1595–1604. doi: 10.1105/tpc.114.135848
Zheng, L., Zhang, M., Zhuo, Z., Wang, Y., Gao, X., and Li, Y. (2021). Transcriptome profiling analysis reveals distinct resistance response of cucumber leaves infected with powdery mildew. Plant Biol. 23, 327–340. doi: 10.1111/plb.13213
Zhi, P., and Chang, C. (2021). Exploiting epigenetic variations for crop disease resistance improvement. Front. Plant Sci. 12:953. doi: 10.3389/fpls.2021.692328
Zhong, C. (2020). Identification and Functional Analysis of miRNAs Against Powdery Mildew in Cucumber. Yangzhou: Yangzhou University. doi: 10.27441/d.cnki.gyzdu.2020.001914
Zhou, H., Dong, C., Zhang, H., Ren, Y., Guo, S., and Yang, W. (2015). SSR molecular markers and location of the gene Foc-4 linked to the resistance to Fusarium wilt of cucumber. Mol. Plant Breed. 13, 1980–1986. doi: 10.13271/j.mpb.013.001980
Keywords: cucumber, powdery mildew, downy mildew, Fusarium wilt, genetic mechanism
Citation: He Y, Wei M, Yan Y, Yu C, Cheng S, Sun Y, Zhu X, Wei L, Wang H and Miao L (2022) Research Advances in Genetic Mechanisms of Major Cucumber Diseases Resistance. Front. Plant Sci. 13:862486. doi: 10.3389/fpls.2022.862486
Received: 26 January 2022; Accepted: 22 February 2022;
Published: 19 May 2022.
Edited by:
Pei Xu, China Jiliang University, ChinaReviewed by:
Tianshu Sun, University of Cambridge, United KingdomChanglong Wen, Beijing Vegetable Research Center, China
Copyright © 2022 He, Wei, Yan, Yu, Cheng, Sun, Zhu, Wei, Wang and Miao. This is an open-access article distributed under the terms of the Creative Commons Attribution License (CC BY). The use, distribution or reproduction in other forums is permitted, provided the original author(s) and the copyright owner(s) are credited and that the original publication in this journal is cited, in accordance with accepted academic practice. No use, distribution or reproduction is permitted which does not comply with these terms.
*Correspondence: Xiangtao Zhu, enh0ODIwMkAxNjMuY29t; Lingling Wei, d2xsQHphZnUuZWR1LmNu; Huasen Wang, d2FuZ2hzQHphZnUuZWR1LmNu; Li Miao, aGFwcHltbWxAMTYzLmNvbQ==
†These authors have contributed equally to this work