- 1Engelhardt Institute of Molecular Biology, Russian Academy of Sciences, Moscow, Russia
- 2Federal Research Center for Bast Fiber Crops, Torzhok, Russia
- 3All-Russian Horticultural Institute for Breeding, Agrotechnology and Nursery, Moscow, Russia
- 4Moscow Institute of Physics and Technology, Moscow, Russia
1 Introduction
Flax (Linum usitatissimum L.) is valued and cultivated worldwide for its dual-purpose use as both a seed and fiber crop. It is one of the oldest domesticated and most widely used crops (Kvavadze et al., 2009). Flax genome has a diploid chromosome set 2x=2n=30 and a size ~450 Mb (Dvorianinova et al., 2022; You et al., 2023). Flax seeds are rich in omega-3 fatty acids, digestible proteins, dietary fiber, and lignans (Goyal et al., 2014). Consumption of linseed oil has beneficial effects on human health, reducing the risks of many disorders and promoting immunity (Mali et al., 2019; Saini et al., 2021; Al-Madhagy et al., 2023). In addition, flax seed oil is used for technical purposes and also serves as a highly nutritious feed for livestock (Xu et al., 2022; Yadav et al., 2024). Flax fiber goes into the production of eco-friendly textiles with high absorption capacity and composite materials (Asyraf et al., 2022; More, 2022).
Different flax varieties are grown for different purposes and vary considerably in their characteristics. Today, traditional breeding is being aided by biotechnology and molecular genetics to select individuals with the desired traits more quickly and efficiently due to high-quality genome assemblies and their annotations. Since it is the study of transcriptomes in various tissues and organs that allows us to establish the associations between a valuable characteristic and its causative region in the genome (Dmitriev et al., 2020; Guo et al., 2020).
To date, there is a great deal of disparate data on gene expression in different flax organs and tissues, under different growth conditions and at different stages of ontogeny. Much work was devoted to the study of the involvement of specific genes in important agronomic traits, resistance to biotic and abiotic stressors, the regulation of organogenesis in flax plants and other characteristics.
Agriculturally valuable traits include those related to yield and to the quality of oil and fiber. The yield and the quality of oil were the subject of great interest of many studies (Xie et al., 2019; Miart et al., 2021; Gao et al., 2022; Jiang et al., 2022; Wang et al., 2022b; Dvorianinova et al., 2023b; Pushkova et al., 2024). Since the valuable product obtained from flax is fiber, the characteristics of phloem fibers were actively studied (Roach and Deyholos, 2007; Zhang and Deyholos, 2016; Gorshkov et al., 2017; Gorshkova et al., 2018; Gorshkov et al., 2019; Galinousky et al., 2020; Mokshina et al., 2020; Petrova et al., 2021; Guo et al., 2022; Mokshina et al., 2022; Yu et al., 2022; Bao et al., 2023; Gorshkova et al., 2023; Liu et al., 2023; Ibragimova and Mokshina, 2024). In addition, transcriptome analysis largely allowed the identification of genes associated with flax plant height (Guo et al., 2021), the length of the growing season, the time of flowering, and the duration of ripening (Gao et al., 2022; House et al., 2022).
Data on flax gene expression and co-expression under suboptimal environmental conditions allowed researches to assess the association of genes with resistance to pathogen infection (Galindo-González and Deyholos, 2016; Dmitriev et al., 2017; Wu et al., 2019b; Boba et al., 2021; He et al., 2022) and abiotic stressors (Yu et al., 2014; Dmitriev et al., 2016; Dash et al., 2017; Wu et al., 2018; Krasnov et al., 2019; Wu et al., 2019a; Huang et al., 2021; Wang et al., 2021; Soto-Cerda et al., 2022; Wang et al., 2022a; Danaeipour et al., 2023; Kostyn et al., 2023; Qiu et al., 2023; Wang et al., 2023; Zhang et al., 2024).
The regulation of organogenesis in flax plays an important role in understanding the development of valuable flax traits (Saha et al., 2021; Yuan et al., 2021; Qi et al., 2023; Zhao et al., 2023). The comparative study of expression profiles of linseed and fiber flax varieties identified genes associated with flax plant type, flax oil odor, and paleohistorical data (Sveinsson et al., 2014; Povkhova et al., 2021; Yang et al., 2022).
Several synthesis articles with annotations for genome assemblies of flax varieties were published: linseed CDC Bethune (Wang et al., 2012) and fiber flax YY5 (Sa et al., 2021). However, the CDC Bethune genome contains some errors because it was assembled only from Illumina reads, which did not allow researchers to resolve its complexity (Sa et al., 2021; Dvorianinova et al., 2023a). The YY5 genome was annotated with transcriptome data of a different variety for only five samples of mature flax plants: leaf, stem, root, flower, and fruit.
In the NCBI database, the reference genome of L. usitatissimum is currently represented by a high-quality assembly of line 3896 (https://www.ncbi.nlm.nih.gov/datasets/genome/GCA_030674075.2/, accessed on 12 October 2024) obtained by us earlier (Dvorianinova et al., 2023a). Line 3896 belongs to the group of linseed flax and is characterized by resistance to Fusarium wilt (Rozhmina and Loshakova, 2016; Dmitriev et al., 2017) and edaphic stressor (low acidity) (Rozhmina et al., 2020), high seed yield and oil content (our observations). In the present study, we complement previous studies of line 3896 with a transcriptome map and genome annotation, which were necessary to make further progress in the field of flax genome research. Our annotation was obtained with the use of RNA-Seq data, whose positive effect on the annotation result was previously shown (Salzberg, 2019; Gabriel et al., 2024). The study of flax genome organization and gene expression will allow the development of methods to obtain improved varieties with desired traits with high efficiency. The results of the study are of use for the identification of genes and polymorphisms responsible for valuable traits and development of modern breeding technologies: genome editing, marker-assisted and genomic selection.
2 Materials and methods
2.1 Plant material
Seeds of linseed line 3896 were provided by the Institute for Flax (Torzhok, Russia). To obtain transcriptome data, we collected a set of organs (Table 1; Figure 1) of line 3896 plants at different stages of vegetation under optimal growth conditions described in the next subsection.
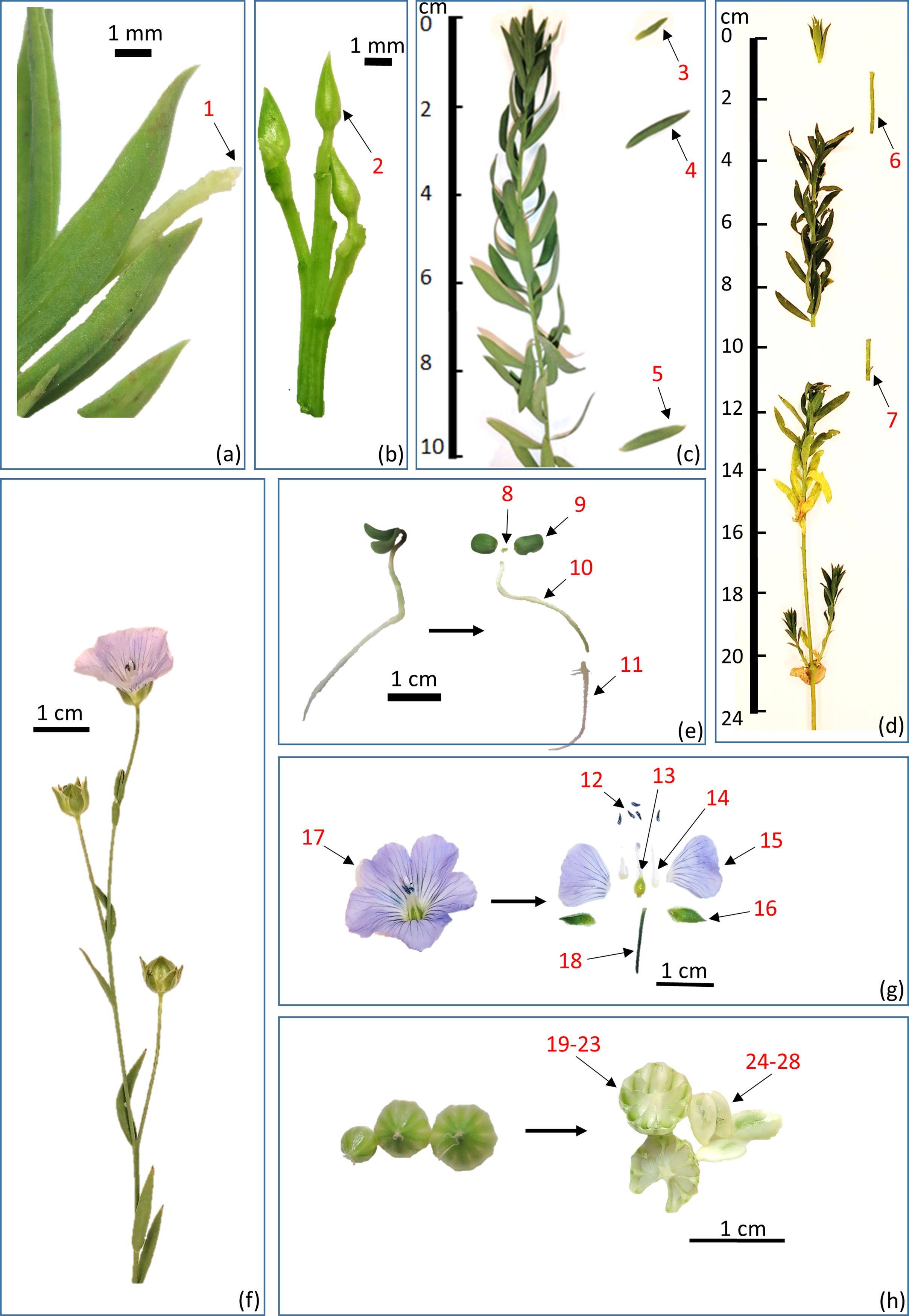
Figure 1. Flax organs/tissues studied by transcriptome analysis: (a-d) Apical parts of stem; (e) Seedling; (f) Mature plant with a flower and capsules; (g) Whole flower and its parts; (h) Capsules and seeds. 1 – SAM (shoot apical meristem); 2 – FAM (floral apical meristem); 3 – Leaf lamina of young leaf; 4 – Leaf lamina of intermediate leaf; 5 – Leaf lamina of mature leaf; 6 – Stem fragment 1-3 cm from the top; 7 – Stem fragment 9-10 cm from the top; 8 – SAM of seedling; 9 – Cotyledons of seedling; 10 – Hypocotyl of seedling; 11 – Root of seedling; 12 – Anthers of mature flower; 13 – Carpel of mature flower; 14 – Stamen filaments of mature flower; 15 – Petals of mature flower; 16 – Sepals of mature flower; 17 – Flower without pedicel; 18 – Pedicel; 19-23 – Capsule; 24-28 – Seeds. Numbers in Figure 1 corresponds to those in Table 1.
2.2 Flax cultivation
Flax seeds were sterilized in 1% sodium hypochlorite and 96% ethanol, after which the seeds were washed twice with water and planted in 0.05% fungicide Maxim (Syngenta, Gaillon, France) solution in Petri dishes for 7 days. Seedlings were planted in the soil and continued to grow under greenhouse conditions at 20°C and ~50-70% relative humidity with regular watering.
For transcriptome analysis, a set of different organs/tissues at different development stages was collected. The plant parts and ontogenetic stages used in the study are listed in Table 1. Each of the samples was collected in two biological replicates. Moreover, each sample was a pool of organs from 2-10 different plants (except capsules and seeds), which is necessary to level out differences between samples and be able to capture trends common to the species (Takele Assefa et al., 2020). The flowers were marked with the date of the day it opened (day of flowering). Seeds from the same capsules were pooled. Capsules were not pooled. The age of the plants at the time of each collection and the collection conditions are shown in Table 1. Samples were collected in the middle of the day between 12 and 15 h under similar conditions to smooth the influence of circadian rhythms on gene expression profiles. Samples were collected in liquid nitrogen and stored at -70°C.
2.3 RNA isolation
Samples #1-6 and #8-18 were grinded using a TissueLyser II homogenizer (Qiagen, Hilden, Germany) with the addition of 3 ceramic beads for two minutes. The harder samples (#7 and #19-28) were homogenized using a disposable pestle inserted in a DeWALT DCD701D2 cordless drill/driver (DeWALT, Towson, MD, USA) at 1200-1500 rpm in 1.5 ml tubes in liquid nitrogen to a fine powder, without allowing the sample to thaw. RNA isolation from samples #1-18 was performed using the Quick-RNA Miniprep Kit (Zymo Research, Irvine, CA, USA). RNA isolation from capsule and seed samples (#19-28) was performed by CTAB with modifications described previously (Pushkova et al., 2024). After that, total RNA was additionally cleaned using the CleanRNA Standard kit (Evrogen, Moscow, Russia) according to the manufacturer’s protocol with a DNAase I treatment step from the RNase-Free DNase Set (Qiagen). The quality of isolated RNA was checked by horizontal electrophoresis in 2% agarose gel and using a 2100 Bioanalyzer (Agilent Technologies, Santa Clara, CA, USA), and concentrations were measured using a Qubit 4 fluorometer (Thermo Fisher Scientific, Waltham, MA, USA).
2.4 cDNA library preparation and sequencing on the Illumina platform
The cDNA libraries were prepared using the QIAseq Stranded mRNA Select Kit (Qiagen) according to the manufacturer’s protocol. The quality of the obtained cDNA libraries (agreement of the length of the obtained libraries with the expected one and the absence of adapter dimers) was assessed on a Qsep1-Plus capillary electrophoresis system (BiOptic, New Taipei City, Taiwan), and the concentration was evaluated on a Qubit 4 fluorometer (Thermo Fisher Scientific). The cDNA libraries (all samples from Table 1) were mixed equimolarly and sequenced on a NextSeq 2000 instrument (Illumina, San Diego, CA, USA) using the NextSeq 2000 P3 Reagents (100 Cycles) kit (Illumina) in 51 + 51 nucleotide format.
2.5 Genome assembly annotation
RNA-Seq reads were trimmed with fastp 0.23.4 using default parameters (Chen et al., 2018). Structural annotation for the flax line 3896 genome assembly (https://www.ncbi.nlm.nih.gov/datasets/genome/GCA_030674075.2/, accessed on 12 October 2024) (Dvorianinova et al., 2023a) was performed using BRAKER3 3.0.8 (Gabriel et al., 2024). This process utilized our RNA-Seq transcriptome data in combination with known Viridiplantae sequences from OrthoDB protein database for gene prediction (Kuznetsov et al., 2023). Functional annotation of the predicted genes was carried out using a local version of InterProScan 5.69-101.0 to assign functional domains and predict gene functions (Jones et al., 2014). The completeness of the annotation was assessed using BUSCO 5.7.1 in protein mode (the eudicots_odb10 dataset). Default parameters of program settings were used in the data analysis.
2.6 Analysis of transcriptome data
Gene expression analysis was performed with PPline (Krasnov et al., 2015) with default parameters of program settings and included the following steps:
● Alignment of the trimmed RNA-Seq reads to the annotated in the present study reference genome of flax line 3896 using STAR 2.7.2b (Dobin et al., 2013).
● Quantification of gene expression: read overlaps with annotated genomic features were counted using featureCounts 1.6.0 (Liao et al., 2014).
● Calculation of the normalized gene expression values, in counts per million (CPM), using edgeR.
● Sample normalization using TMM to account for differences in library sizes and composition between samples.
2.7 Gene enrichment analysis
Custom Gene Ontology (GO) annotation for line 3896 was constructed using EggNOG-mapper (–tax_scope 33090) (emapper 2.1.12, eggNOG DB version: 5.0.2) for the longest proteins in the constructed gene annotation (Huerta-Cepas et al., 2019; Cantalapiedra et al., 2021). Differential gene expression analysis was conducted for a balanced subset of flax samples: mature leaf blades from the shoot at 10 cm from the top at 30 days after germination (DAG), stem fragments 1-3 cm from the top at 30 DAG, roots of seedlings at 5 DAG, hypocotyls of seedlings at 5 DAG, mature flower carpels before pollination at 56 DAG, capsule without seeds at 14 days after flowering (DAF), seeds without capsule at 14 DAF, shoot apical meristems (SAM) of seedlings at 5 DAG. Gene expression in each tissue was compared to that in the other tissues from the subset of flax samples. Genes with FDR (QLF) < 0.05 and logFC > 1.5 were selected for gene enrichment analysis. Gene enrichment was performed using the constructed annotation, the selected gene lists, and the topGO 2.54.0 package (Alexa and Rahnenfuhrer, 2023).
3 Preliminary data analysis
3.1 RNA-seq data characteristics
This article presents data of transcriptome analysis of 28 organs/tissues of flax line 3896: 7 samples of vegetative organs of actively growing individuals, 4 samples of seedlings, 7 samples of various parts of generative organs at flowering stage, and 10 samples of fruits and seeds at different stages of maturation (Table 1; Figure 1). We set out to cover all growth stages of flax and all key events in the development of valuable flax traits. From 5.4 to 20.5 million raw reads (51 + 51 bp) were obtained for each sample on the Illumina platform (two biological replicates were sequenced for each sample). The raw data were deposited in the NCBI Sequence Read Archive (SRA) under the BioProject accession number PRJNA1172129.
After trimming, the reads were mapped to the genome assembly of line 3896, and on average ~95% of the reads for each sample were mapped (on average ~90% were uniquely mapped), confirming the high quality of the transcriptome data.
3.2 Genome annotation
We collected extensive data on gene expression in 28 organs/tissues of flax line 3896 at different development stages. Using the obtained transcriptome data and the Viridiplantae protein sequence database, we annotated the line 3896 genome assembly with BRAKER3: 39,055 genes and 45,154 transcripts were predicted, and 37,787 of these genes were annotated using InterProScan (Supplementary Data Sheet S1). The high completeness of annotation was achieved according to BUSCO (Benchmarking Universal Single-Copy Orthologs) – 95.6% (eudicots). Notably, 22.2% of all BUSCO were complete and single-copy and 73.4% were complete and duplicated. Such a high percentage of duplicated BUSCO was expected for an ancient tetraploid (Bolsheva et al., 2017).
3.3 Transcriptome map
Utilizing the obtained annotation of line 3896, we performed an analysis of our transcriptome data, which resulted in the identification of genes exhibiting tissue-specific and development stage-specific expression patterns within flax organs/tissues. This analysis led to the generation of a comprehensive transcriptome map for line 3896. To present the data in a convenient format for further analysis, we used PPline and RTrans (https://github.com/gskrasnov/RTrans, accessed on 17 October 2024). It was applied to evaluate the expression levels of the identified genes as read counts per million reads (CPM). Our transcriptome map is summarized in Supplementary Table S1 and presented as a heatmap (Supplementary Figure S1).
3.4 Gene pathway enrichment analysis
During the gene pathway enrichment analysis, the following was found out. For leaves collected at a distance of 10 cm from the apex, compared to the other organs/tissues, the GO terms were represented by the processes of photosynthesis, carbohydrate metabolism, plastid organization, electron transport chain, pigment synthesis, and transmembrane transport. These processes are characteristic of the main photosynthetic organ of the plant in the active phase (Müller and Munné-Bosch, 2021; Leister, 2023). In the stem fragment, located 1-3 cm from the top, the processes of vascular tissue histogenesis, vascular and phloem transport, stem morphogenesis, and response to auxin synthesized in the apical meristem predominated. This is logical, since we were dealing with an axial organ whose main function is the transport of metabolites, and the incision was made close to the site of differentiation (Yoshida et al., 2009; Kułak et al., 2023). It was determined that the major pathways in the seedling root included the processes of water and solute transport, root hair formation and growth, response to chemical and mechanical stimuli, and metabolism of auxin, other hormones, and secondary metabolites, which are the main processes occurring in the roots of vascular plants (Vissenberg et al., 2020; Li et al., 2021; Castillo-Jiménez et al., 2023). GO analysis of the hypocotyl transcriptome revealed differential expression of genes related to pathways of amino acid biosynthesis, as well as active regulation of biosynthetic processes, brassinosteroid metabolism, and cell growth. These findings indicate the presence of active development processes in the axial organs of seedlings (Favero et al., 2021). GO analysis for flax pistil demonstrated the representation of genes that are associated with the formation and development of generative structures, pollination, and pollen tube growth. Furthermore, it demonstrated representation of genes associated with active ion metabolism, which is necessary for directed pollen tube growth (Zhou et al., 2022). GO analysis for a capsule (14 DAF) indicated that the GO terms associated with the formation of secondary cell wall, synthesis of its components, and lignification prevailed. These processes enable the preparation of dry fruits for opening and seed dispersal (Seymour et al., 2013). Additionally, catabolism of organic compounds used for cell wall construction and seed maturation is active in ripening capsules. The differential expression pattern of flax seeds (14 DAF) was dominated by processes related to seed and fruit development and maturation, lipid storage and fatty acid synthesis, as well as abscisic acid (ABA) metabolism and regulation. Oil accumulation corresponds to the primary function of the seed as a reproductive organ, and ABA regulates its maturation (Sano and Marion-Poll, 2021; Dvorianinova et al., 2023b). The shoot apical meristem displayed a hallmark pattern indicative of actively dividing cells. The process of SAM is characterized by the macromolecule biosynthesis, ribosome assembly, translation, RNA processing, DNA reparation, organelle formation, nucleosome assembly, and chromatin remodeling (Xue et al., 2020; Burian, 2021). Thus, the results of the gene enrichment analysis of the subset of flax samples in general looked logical and confirmed the adequacy of the obtained data. The results are presented in detail in Supplementary Table S2.
4 Conclusions
Flax is of great industrial and nutritional value and is therefore actively studied at the molecular-genetic level. To date, there are several flax genome assemblies, some with annotations, and many scattered gene expression data for different varieties. In this study, we used Illumina sequencing to obtain comprehensive transcriptome data for flax line 3896, whose genome is currently a reference for the species Linum usitatissimum L. in the NCBI database. Gene expression profiles were analyzed in 28 various flax organs/tissues at different stages of ontogenesis. With these data we were able to annotate the genome of line 3896 and generate a high-quality transcriptome map. The transcriptome map will allow the identification of genes that have a high expression level in a particular organ/tissue. Such genes may play a key role in the biological processes taking place in that organ/tissue. In addition, data on gene expression profiles during plant development can help to determine the most important time points at which the processes of interest occur. The transcriptome map also allows the determination of gene functions based not only on homology analysis, but also taking into account gene expression patterns in different organs/tissues. Thus, the transcriptome map and annotation presented in this work allow reaching a new level in the molecular-genetic studies of flax, the search for key genes responsible for the valuable traits, the development of new approaches in flax breeding and the creation of improved varieties.
Data availability statement
The datasets presented in this study can be found in online repositories. The names of the repository/repositories and accession number(s) can be found below: https://www.ncbi.nlm.nih.gov/, PRJNA1172129.
Author contributions
DZ: Conceptualization, Investigation, Writing – original draft, Writing – review & editing. AA: Investigation, Writing – original draft, Writing – review & editing. TR: Investigation, Writing – review & editing. AZ: Investigation, Writing – review & editing. NB: Investigation, Writing – review & editing. ES: Investigation, Writing – review & editing. ED: Investigation, Writing – review & editing. EB: Investigation, Writing – review & editing. EP: Investigation, Writing – review & editing. NM: Conceptualization, Investigation, Writing – review & editing. AD: Conceptualization, Investigation, Writing – review & editing.
Funding
The author(s) declare that financial support was received for the research and/or publication of this article. This work was financially supported by the Russian Science Foundation, grant number 24-64-00033, https://rscf.ru/project/24-64-00033/.
Acknowledgments
This work was performed using the equipment of the EIMB RAS “Genome” center (http://www.eimb.ru/ru1/ckp/ccu_genome_ce.php).
Conflict of interest
The authors declare that the research was conducted in the absence of any commercial or financial relationships that could be construed as a potential conflict of interest.
Generative AI statement
The author(s) declare that no Generative AI was used in the creation of this manuscript.
Publisher’s note
All claims expressed in this article are solely those of the authors and do not necessarily represent those of their affiliated organizations, or those of the publisher, the editors and the reviewers. Any product that may be evaluated in this article, or claim that may be made by its manufacturer, is not guaranteed or endorsed by the publisher.
Supplementary material
The Supplementary Material for this article can be found online at: https://www.frontiersin.org/articles/10.3389/fpls.2025.1520832/full#supplementary-material
Supplementary Figure S1 | Expression heatmap based on log2(CPM/avg.CPM) of annotated genes in 28 organs/tissues of flax line 3896.
Supplementary Table S1 | Expression levels of annotated genes in 28 organs/tissues of flax line 3896 as read counts per million reads (CPM).
Supplementary Table S2 | Gene enrichment analysis for a balanced subset of flax samples: mature leaf blades from the shoot at 10 cm from the top at 30 DAG, stem fragments 1-3 cm from the top at 30 DAG, roots of seedlings at 5 DAG, hypocotyls of seedlings at 5 DAG, mature flower carpels before pollination at 56 DAG, capsule without seeds at 14 DAF, seeds without capsule at 14 DAF, SAM (shoot apical meristem) of seedlings at 5 DAG.
Supplementary Data Sheet S1 | Annotation of flax line 3896 genome assembly.
References
Alexa, A. and Rahnenfuhrer, J. (2023). topGO: Enrichment Analysis for Gene Ontology (2.54.0). doi: 10.18129/B9.bioc.topGO
Al-Madhagy, S., Ashmawy, N. S., Mamdouh, A., Eldahshan, O. A., and Farag, M. A. (2023). A comprehensive review of the health benefits of flaxseed oil in relation to its chemical composition and comparison with other omega-3-rich oils. Eur. J. Med. Res. 28, 240. doi: 10.1186/s40001-023-01203-6
Asyraf, M. R. M., Ishak, M. R., Norrrahim, M. N. F., Amir, A. L., Nurazzi, N. M., Ilyas, R. A., et al. (2022). Potential of flax fiber reinforced biopolymer composites for cross-arm application in transmission tower: a review. Fibers Polymers 23, 853–877. doi: 10.1007/s12221-022-4383-x
Bao, Y., Zou, Y., Tian, R., Huang, X., Liu, L., Wang, B., et al. (2023). Transcriptome analysis of fiber development under high-temperature stress in flax (Linum usitatissimum L.). Ind. Crops Prod. 195, 116019. doi: 10.1016/j.indcrop.2022.116019
Boba, A., Kostyn, K., Kozak, B., Zalewski, I., Szopa, J., and Kulma, A. (2021). Transcriptomic profiling of susceptible and resistant flax seedlings after Fusarium oxysporum lini infection. PloS One 16, e0246052. doi: 10.1371/journal.pone.0246052
Bolsheva, N. L., Melnikova, N. V., Kirov, I. V., Speranskaya, A. S., Krinitsina, A. A., Dmitriev, A. A., et al. (2017). Evolution of blue-flowered species of genus Linum based on high-throughput sequencing of ribosomal RNA genes. BMC Evolutionary Biol. 17, 253. doi: 10.1186/s12862-017-1105-x
Burian, A. (2021). Does shoot apical meristem function as the germline in safeguarding against excess of mutations? Front. Plant Sci. 12. doi: 10.3389/fpls.2021.707740
Cantalapiedra, C. P., Hernández-Plaza, A., Letunic, I., Bork, P., and Huerta-Cepas, J. (2021). eggNOG-mapper v2: functional annotation, orthology assignments, and domain prediction at the metagenomic scale. Mol. Biol. Evol. 38, 5825–5829. doi: 10.1093/molbev/msab293
Castillo-Jiménez, A., Garay-Arroyo, A., de la Paz Sánchez, M., García-Ponce, B., Martínez-García, J. C., and Álvarez-Buylla, E. R. (2023). Extended discrete gene regulatory network model for the Arabidopsis thaliana root-hair cell fate. bioRxiv. doi: 10.1101/2023.11.15.567304
Chen, S., Zhou, Y., Chen, Y., and Gu, J. (2018). fastp: an ultra-fast all-in-one FASTQ preprocessor. Bioinformatics 34, i884–i890. doi: 10.1093/bioinformatics/bty560
Danaeipour, Z., Garoosi, G., Tohidfar, M., Bakhtiari Zadeh, M. R., and Mirjalili, M. H. (2023). Data mining of flax transcriptome profile under stress aluminum toxicity and zinc deficiency. MGJ 18, 21–32.
Dash, P. K., Rai, R., Mahato, A. K., Gaikwad, K., and Singh, N. K. (2017). Transcriptome landscape at different developmental stages of a drought tolerant cultivar of flax (Linum usitatissimum). Front. Chem. 5. doi: 10.3389/fchem.2017.00082
Dmitriev, A. A., Krasnov, G. S., Rozhmina, T. A., Novakovskiy, R. O., Snezhkina, A. V., Fedorova, M. S., et al. (2017). Differential gene expression in response to Fusarium oxysporum infection in resistant and susceptible genotypes of flax (Linum usitatissimum L.). BMC Plant Biol. 17, 253. doi: 10.1186/s12870-017-1192-2
Dmitriev, A. A., Kudryavtseva, A. V., Krasnov, G. S., Koroban, N. V., Speranskaya, A. S., Krinitsina, A. A., et al. (2016). Gene expression profiling of flax (Linum usitatissimum L.) under edaphic stress. BMC Plant Biol. 16, 237. doi: 10.1186/s12870-016-0927-9
Dmitriev, A. A., Novakovskiy, R. O., Pushkova, E. N., Rozhmina, T. A., Zhuchenko, A. A., Bolsheva, N. L., et al. (2020). Transcriptomes of different tissues of flax (Linum usitatissimum L.) cultivars with diverse characteristics. Front. Genet. 11. doi: 10.3389/fgene.2020.565146
Dobin, A., Davis, C. A., Schlesinger, F., Drenkow, J., Zaleski, C., Jha, S., et al. (2013). STAR: ultrafast universal RNA-seq aligner. Bioinformatics 29, 15–21. doi: 10.1093/bioinformatics/bts635
Dvorianinova, E. M., Bolsheva, N. L., Pushkova, E. N., Rozhmina, T. A., Zhuchenko, A. A., Novakovskiy, R. O., et al. (2022). Isolating Linum usitatissimum L. nuclear DNA enabled assembling high-quality genome. Int. J. Mol. Sci. 23, 13244. doi: 10.3390/ijms232113244
Dvorianinova, E., Pushkova, E., Bolsheva, N., Rozhmina, T., Zhernova, D., Sigova, E., et al. (2023a). Improving genome assembly of flax line 3896 with high-precision Illumina reads. Russian J. Genet. 59, S237–S240. doi: 10.1134/S102279542314003X
Dvorianinova, E. M., Zinovieva, O. L., Pushkova, E. N., Zhernova, D. A., Rozhmina, T. A., Povkhova, L. V., et al. (2023b). Key FAD2, FAD3, and SAD genes involved in the fatty acid synthesis in flax identified based on genomic and transcriptomic data. Int. J. Mol. Sci. 24, 14885. doi: 10.3390/ijms241914885
Favero, D. S., Lambolez, A., and Sugimoto, K. (2021). Molecular pathways regulating elongation of aerial plant organs: a focus on light, the circadian clock, and temperature. Plant J. 105, 392–420. doi: 10.1111/tpj.14996
Gabriel, L., Brůna, T., Hoff, K. J., Ebel, M., Lomsadze, A., Borodovsky, M., et al. (2024). BRAKER3: Fully automated genome annotation using RNA-seq and protein evidence with GeneMark-ETP, AUGUSTUS, and TSEBRA. Genome Res. 34, 769–777. doi: 10.1101/gr.278090.123
Galindo-González, L. and Deyholos, M. K. (2016). RNA-seq transcriptome response of flax (Linum usitatissimum L.) to the pathogenic fungus Fusarium oxysporum f. sp. lini. Front. Plant Sci. 7. doi: 10.3389/fpls.2016.01766
Galinousky, D., Padvitski, T., Mokshina, N., Gorshkov, O., Khotyleva, L., Gorshkova, T., et al. (2020). Expression of cellulose synthase-like genes in two phenotypically distinct flax (Linum usitatissimum L.) subspecies. Genet. Resour. Crop Evol. 67, 1821–1837. doi: 10.1007/s10722-020-00943-2
Gao, P., Qiu, S., Ma, X., Parkin, I. A., Xiang, D., and Datla, R. (2022). Spatiotemporal transcriptomic atlas of developing embryos and vegetative tissues in flax. Plants 11, 2031. doi: 10.3390/plants11152031
Gorshkov, O., Chernova, T., Mokshina, N., Gogoleva, N., Suslov, D., Tkachenko, A., et al. (2019). Intrusive growth of phloem fibers in flax stem: integrated analysis of miRNA and mRNA expression profiles. Plants 8, 47. doi: 10.3390/plants8020047
Gorshkov, O., Mokshina, N., Gorshkov, V., Chemikosova, S., Gogolev, Y., and Gorshkova, T. (2017). Transcriptome portrait of cellulose-enriched flax fibres at advanced stage of specialization. Plant Mol. Biol. 93, 431–449. doi: 10.1007/s11103-016-0571-7
Gorshkova, T., Chernova, T., Mokshina, N., Gorshkov, V., Kozlova, L., and Gorshkov, O. (2018). Transcriptome analysis of intrusively growing flax fibers isolated by laser microdissection. Sci. Rep. 8, 14570. doi: 10.1038/s41598-018-32869-2
Gorshkova, T., Mokshina, N., Mitsuda, N., Gorshkov, O., and You, F. M. (2023). “Key stages of flax bast fiber development through the prism of transcriptomics,” in The flax genome. Ed. Fofana, B. (Springer, Cham), 149–198.
Goyal, A., Sharma, V., Upadhyay, N., Gill, S., and Sihag, M. (2014). Flax and flaxseed oil: an ancient medicine & modern functional food. J. Food Sci. Technol. 51, 1633–1653. doi: 10.1007/s13197-013-1247-9
Guo, D., Jiang, H., Yan, W., Yang, L., Ye, J., Wang, Y., et al. (2020). Resequencing 200 flax cultivated accessions identifies candidate genes related to seed size and weight and reveals signatures of artificial selection. Front. Plant Sci. 10. doi: 10.3389/fpls.2019.01682
Guo, D., Jiang, H., Ye, J., Zhang, A., Wang, Y., Gao, Y., et al. (2021). Transcriptome combined with population level validation reveals genomic loci controlling plant height in flax (Linum usitatissimum L.). Ind. Crops Prod. 172, 113998. doi: 10.1016/j.indcrop.2021.113998
Guo, Y., Wen, L., Chen, J., Pan, G., Wu, Z., Li, Z., et al. (2022). Comparative transcriptomic analysis identifies key cellulose synthase genes (CESA) and cellulose synthase-like genes (CSL) in fast growth period of flax stem (Linum usitatissimum l.). J. Natural Fibers 19, 10431–10446. doi: 10.1080/15440478.2021.1993510
He, R., Chang, Y., and Wang, J. (2022). Identification of genes responsible for stress resistance in Fusarium oxysporum-inoculated flax seedlings using weighted gene co-expression network analysis. Eur. J. Plant Pathol. 163, 513–528. doi: 10.1007/s10658-022-02497-8
House, M. A., Young, L. W., Robinson, S. J., and Booker, H. M. (2022). Transcriptomic analysis of early flowering signals in ‘Royal’Flax. Plants 11, 860. doi: 10.3390/plants11070860
Huang, W.-G., Jiang, W.-D., Yao, Y.-B., Song, X.-X., Liu, Y., Chen, S., et al. (2021). Transcriptome profiling of flax (Linum usttatissimum L.) response to low potassium stress. Acta Agronomica Sin. 47, 1070–1081. doi: 10.3724/SP.J.1006.2021.04133
Huerta-Cepas, J., Szklarczyk, D., Heller, D., Hernández-Plaza, A., Forslund, S. K., Cook, H., et al. (2019). eggNOG 5.0: a hierarchical, functionally and phylogenetically annotated orthology resource based on 5090 organisms and 2502 viruses. Nucleic Acids Res. 47, D309–D314. doi: 10.1093/nar/gky1085
Ibragimova, N. and Mokshina, N. (2024). Callose metabolism in flax fibers during gravity response: analysis of gene expression. Russian J. Plant Physiol. 71, 57. doi: 10.1134/S1021443724604567
Jiang, H., Guo, D., Liu, Y., Zhu, L., Xie, F., and Xie, L. (2022). RNA-Seq combined with population-level analysis reveals important candidate genes related to seed size in flax (Linum usitatissimum L.). Front. Plant Sci. 13. doi: 10.3389/fpls.2022.1015399
Jones, P., Binns, D., Chang, H.-Y., Fraser, M., Li, W., McAnulla, C., et al. (2014). InterProScan 5: genome-scale protein function classification. Bioinformatics 30, 1236–1240. doi: 10.1093/bioinformatics/btu031
Kostyn, K., Boba, A., Kozak, B., Sztafrowski, D., Widuła, J., Szopa, J., et al. (2023). Transcriptome profiling of flax plants exposed to a low-frequency alternating electromagnetic field. Front. Genet. 14. doi: 10.3389/fgene.2023.1205469
Krasnov, G. S., Dmitriev, A. A., Kudryavtseva, A. V., Shargunov, A. V., Karpov, D. S., Uroshlev, L. A., et al. (2015). PPLine: an automated pipeline for SNP, SAP, and splice variant detection in the context of proteogenomics. J. Proteome Res. 14, 3729–3737. doi: 10.1021/acs.jproteome.5b00490
Krasnov, G. S., Dmitriev, A. A., Zyablitsin, A. V., Rozhmina, T. A., Zhuchenko, A. A., Kezimana, P., et al. (2019). Aluminum responsive genes in flax (Linum usitatissimum L.). BioMed. Res. Int. 2019, 5023125. doi: 10.1155/2019/5023125
Kułak, K., Wojciechowska, N., Samelak-Czajka, A., Jackowiak, P., and Bagniewska-Zadworna, A. (2023). How to explore what is hidden? A review of techniques for vascular tissue expression profile analysis. Plant Methods 19, 129. doi: 10.1186/s13007-023-01109-8
Kuznetsov, D., Tegenfeldt, F., Manni, M., Seppey, M., Berkeley, M., Kriventseva, E. V., et al. (2023). OrthoDB v11: annotation of orthologs in the widest sampling of organismal diversity. Nucleic Acids Res. 51, D445–D451. doi: 10.1093/nar/gkac998
Kvavadze, E., Bar-Yosef, O., Belfer-Cohen, A., Boaretto, E., Jakeli, N., Matskevich, Z., et al. (2009). 30,000-year-old wild flax fibers. Science 325, 1359–1359. doi: 10.1126/science.1175404
Leister, D. (2023). Enhancing the light reactions of photosynthesis: Strategies, controversies, and perspectives. Mol. Plant 16, 4–22. doi: 10.1016/j.molp.2022.08.005
Li, H., Testerink, C., and Zhang, Y. (2021). How roots and shoots communicate through stressful times. Trends Plant Sci. 26, 940–952. doi: 10.1016/j.tplants.2021.03.005
Liao, Y., Smyth, G. K., and Shi, W. (2014). featureCounts: an efficient general purpose program for assigning sequence reads to genomic features. Bioinformatics 30, 923–930. doi: 10.1093/bioinformatics/btt656
Liu, D., Yuan, H., Yao, Y., Cheng, L., Tang, L., Kang, Q., et al. (2023). Genome-wide identification and expression pattern analysis of the F5H gene family in flax (Linum usitatissimum L.). Agronomy 13, 1108. doi: 10.3390/agronomy13041108
Mali, A. V., Padhye, S. B., Anant, S., Hegde, M. V., and Kadam, S. S. (2019). Anticancer and antimetastatic potential of enterolactone: Clinical, preclinical and mechanistic perspectives. Eur. J. Pharmacol. 852, 107–124. doi: 10.1016/j.ejphar.2019.02.022
Miart, F., Fontaine, J.-X., Mongelard, G., Wattier, C., Lequart, M., Bouton, S., et al. (2021). Integument-specific transcriptional regulation in the mid-stage of flax seed development influences the release of mucilage and the seed oil content. Cells 10, 2677. doi: 10.3390/cells10102677
Mokshina, N., Gorshkov, O., Galinousky, D., and Gorshkova, T. (2020). Genes with bast fiber-specific expression in flax plants-Molecular keys for targeted fiber crop improvement. Ind. Crops Prod. 152, 112549. doi: 10.1016/j.indcrop.2020.112549
Mokshina, N., Mikshina, P., and Gorshkova, T. (2022). Expression of cellulose synthase genes during the gravistimulation of flax (Linum usitatissimum) and poplar (Populus alba× tremula) plants. Russian J. Bioorg. Chem. 48, 529–542. doi: 10.1134/S106816202203013X
More, A. P. (2022). Flax fiber–based polymer composites: a review. Advanced Composites Hybrid Mater. 5, 1–20. doi: 10.1007/s42114-021-00246-9
Müller, M. and Munné-Bosch, S. (2021). Hormonal impact on photosynthesis and photoprotection in plants. Plant Physiol. 185, 1500–1522. doi: 10.1093/plphys/kiaa119
Petrova, N., Nazipova, A., Gorshkov, O., Mokshina, N., Patova, O., and Gorshkova, T. (2021). Gene expression patterns for proteins with lectin domains in flax stem tissues are related to deposition of distinct cell wall types. Front. Plant Sci. 12. doi: 10.3389/fpls.2021.634594
Povkhova, L. V., Melnikova, N. V., Rozhmina, T. A., Novakovskiy, R. O., Pushkova, E. N., Dvorianinova, E. M., et al. (2021). Genes associated with the flax plant type (oil or fiber) identified based on genome and transcriptome sequencing data. Plants 10, 2616. doi: 10.3390/plants10122616
Pushkova, E., Dvorianinova, E., Povkhova, L., Rozhmina, T., Novakovskiy, R., Sigova, E., et al. (2024). Expression profiles of genes involved in lignan synthesis in developing flax seeds. Russian J. Genet. 60, 987–991. doi: 10.1134/S1022795424700443
Qi, F., Wang, F., Xiaoyang, C., Wang, Z., Lin, Y., Peng, Z., et al. (2023). Gene expression analysis of different organs and identification of AP2 transcription factors in flax (Linum usitatissimum L.). Plants 12, 3260. doi: 10.3390/plants12183260
Qiu, C., Qiu, H., Peng, D., Chen, J., Wang, Y., Stybayev, G., et al. (2023). The mechanisms underlying physiological and molecular responses to waterlogging in flax. J. Natural Fibers 20, 2198275. doi: 10.1080/15440478.2023.2198275
Roach, M. J. and Deyholos, M. K. (2007). Microarray analysis of flax (Linum usitatissimum L.) stems identifies transcripts enriched in fibre-bearing phloem tissues. Mol. Genet. Genomics 278, 149–165. doi: 10.1007/s00438-007-0241-1
Rozhmina, T. and Loshakova, N. (2016). New sources of effective resistance genes to fusarium wilt in flax (Linum usitatissimum L.) depending on temperature. Agric. Biol. 51, 310–317. doi: 10.15389/agrobiology.2016.3.310eng
Rozhmina, T., Zhuchenko, A., Melnikova, N., and Smirnova, A. (2020). Resistance of flax gene pool samples to edaphic stress caused by low acidity. Agric. Sci. Euro North East 21, 133–140. doi: 10.30766/2072-9081.2020.21.2.133-140
Sa, R., Yi, L., Siqin, B., An, M., Bao, H., Song, X., et al. (2021). Chromosome-level genome assembly and annotation of the fiber flax (Linum usitatissimum) genome. Front. Genet. 12. doi: 10.3389/fgene.2021.735690
Saha, D., Shaw, A. K., Datta, S., and Mitra, J. (2021). Evolution and functional diversity of abiotic stress-responsive NAC transcription factor genes in Linum usitatissimum L. Environ. Exp. Bot. 188, 104512. doi: 10.1016/j.envexpbot.2021.104512
Saini, R. K., Prasad, P., Sreedhar, R. V., Akhilender Naidu, K., Shang, X., and Keum, Y.-S. (2021). Omega-3 polyunsaturated fatty acids (PUFAs): Emerging plant and microbial sources, oxidative stability, bioavailability, and health benefits — A review. Antioxidants 10, 1627. doi: 10.3390/antiox10101627
Salzberg, S. L. (2019). Next-generation genome annotation: we still struggle to get it right. Genome Biol. 20, 92. doi: 10.1186/s13059-019-1715-2
Sano, N. and Marion-Poll, A. (2021). ABA metabolism and homeostasis in seed dormancy and germination. Int. J. Mol. Sci. 22, 5069. doi: 10.3390/ijms22105069
Seymour, G. B., Østergaard, L., Chapman, N. H., Knapp, S., and Martin, C. (2013). Fruit development and ripening. Annu. Rev. Plant Biol. 64, 219–241. doi: 10.1146/annurev-arplant-050312-120057
Soto-Cerda, B. J., Larama, G., Gajardo, H., Inostroza-Blancheteau, C., Cloutier, S., Fofana, B., et al. (2022). Integrating multi-locus genome-wide association studies with transcriptomic data to identify genetic loci underlying adult root trait responses to drought stress in flax (Linum usitatissimum L.). Environ. Exp. Bot. 202, 105019. doi: 10.1016/j.envexpbot.2022.105019
Sveinsson, S., McDill, J., Wong, G. K., Li, J., Li, X., Deyholos, M. K., et al. (2014). Phylogenetic pinpointing of a paleopolyploidy event within the flax genus (Linum) using transcriptomics. Ann. Bot. 113, 753–761. doi: 10.1093/aob/mct306
Takele Assefa, A., Vandesompele, J., and Thas, O. (2020). On the utility of RNA sample pooling to optimize cost and statistical power in RNA sequencing experiments. BMC Genomics 21, 312. doi: 10.1186/s12864-020-6721-y
Vissenberg, K., Claeijs, N., Balcerowicz, D., and Schoenaers, S. (2020). Hormonal regulation of root hair growth and responses to the environment in Arabidopsis. J. Exp. Bot. 71, 2412–2427. doi: 10.1093/jxb/eraa048
Wang, Z., Hobson, N., Galindo, L., Zhu, S., Shi, D., McDill, J., et al. (2012). The genome of flax (Linum usitatissimum) assembled de novo from short shotgun sequence reads. Plant J. 72, 461–473. doi: 10.1111/j.1365-313X.2012.05093.x
Wang, N., Lin, Y., Qi, F., Xiaoyang, C., Peng, Z., Yu, Y., et al. (2022a). Comprehensive analysis of differentially expressed genes and epigenetic modification-related expression variation induced by saline stress at seedling stage in fiber and oil flax, Linum usitatissimum L. Plants 11, 2053. doi: 10.3390/plants11152053
Wang, N., Qi, F., Wang, F., Lin, Y., Xiaoyang, C., Peng, Z., et al. (2023). Evaluation of differentially expressed genes in leaves vs. roots subjected to drought stress in flax (Linum usitatissimum L.). Int. J. Mol. Sci. 24, 12019. doi: 10.3390/ijms241512019
Wang, W., Wang, L., Wang, L., Tan, M., Ogutu, C. O., Yin, Z., et al. (2021). Transcriptome analysis and molecular mechanism of linseed (Linum usitatissimum L.) drought tolerance under repeated drought using single-molecule long-read sequencing. BMC Genomics 22, 109. doi: 10.1186/s12864-021-07416-5
Wang, S., Xu, J., Wang, Z., Li, Z., Yi, L., Yao, L., et al. (2022b). Gene screening for fatty acid synthesis of flax based on transcriptome sequencing. Food Sci. Technol. 42, e93721. doi: 10.1590/fst.93721
Wu, W., Nemri, A., Blackman, L. M., Catanzariti, A.-M., Sperschneider, J., Lawrence, G. J., et al. (2019b). Flax rust infection transcriptomics reveals a transcriptional profile that may be indicative for rust Avr genes. PloS One 14, e0226106. doi: 10.1371/journal.pone.0226106
Wu, J., Zhao, Q., Sun, D., Wu, G., Zhang, L., Yuan, H., et al. (2018). Transcriptome analysis of flax (Linum usitatissimum L.) undergoing osmotic stress. Ind. Crops Prod. 116, 215–223. doi: 10.1016/j.indcrop.2018.02.035
Wu, J., Zhao, Q., Wu, G., Yuan, H., Ma, Y., Lin, H., et al. (2019a). Comprehensive analysis of differentially expressed unigenes under NaCl stress in flax (Linum usitatissimum L.) using RNA-Seq. Int. J. Mol. Sci. 20, 369. doi: 10.3390/ijms20020369
Xie, D., Dai, Z., Yang, Z., Tang, Q., Deng, C., Xu, Y., et al. (2019). Combined genome-wide association analysis and transcriptome sequencing to identify candidate genes for flax seed fatty acid metabolism. Plant Sci. 286, 98–107. doi: 10.1016/j.plantsci.2019.06.004
Xu, L., Wei, Z., Guo, B., Bai, R., Liu, J., Li, Y., et al. (2022). Flaxseed meal and its application in animal husbandry: A review. Agriculture 12, 2027. doi: 10.3390/agriculture12122027
Xue, Z., Liu, L., and Zhang, C. (2020). Regulation of shoot apical meristem and axillary meristem development in plants. Int. J. Mol. Sci. 21, 2917. doi: 10.3390/ijms21082917
Yadav, S., Sharma, K., and Singh, D. (2024). “Modification methods of linseed oil and its applications in different product development,” in Linseed. Eds. Langyan, S. and Kumar., A. (Elsevier, Amsterdam), 219–225.
Yang, J.-W., Li, H.-K., Lin, Y.-F., Liu, W.-Y., and Wei, C.-Q. (2022). Transcriptome analysis of two flaxseed cultivars grown in Xinjiang and mining of differentially expressed genes related to flaxseed oil aroma. Food Sci. 43, 70–76. doi: 10.7506/spkx1002-6630-20201120-213
Yoshida, S., Iwamoto, K., Demura, T., and Fukuda, H. (2009). Comprehensive analysis of the regulatory roles of auxin in early transdifferentiation into xylem cells. Plant Mol. Biol. 70, 457–469. doi: 10.1007/s11103-009-9485-y
You, F. M., Moumen, I., Khan, N., and Cloutier, S. (2023). “Reference genome sequence of flax,” in The flax genome. Eds. You, F. M. and Fofana, B. (Springer, Cham), 1–17.
Yu, Y., Huang, W., Chen, H., Wu, G., Yuan, H., Song, X., et al. (2014). Identification of differentially expressed genes in flax (Linum usitatissimum L.) under saline–alkaline stress by digital gene expression. Gene 549, 113–122. doi: 10.1016/j.gene.2014.07.053
Yu, Y., Yuan, H., Zhao, D., Yao, Y., Zhou, F., Cheng, L., et al. (2022). Transcriptome analysis of two flax varieties at different developmental stages reveals significant differential expression of genes related to stem fiber development. J. Natural Fibers 19, 1428–1442. doi: 10.1080/15440478.2020.1776662
Yuan, H., Guo, W., Zhao, L., Yu, Y., Chen, S., Tao, L., et al. (2021). Genome-wide identification and expression analysis of the WRKY transcription factor family in flax (Linum usitatissimum L.). BMC Genomics 22, 375. doi: 10.1186/s12864-021-07697-w
Zhang, N. and Deyholos, M. K. (2016). RNASeq analysis of the shoot apex of flax (Linum usitatissimum) to identify phloem fiber specification genes. Front. Plant Sci. 7. doi: 10.3389/fpls.2016.00950
Zhang, F., Liu, Y., Ma, J., Su, S., Chen, L., Cheng, Y., et al. (2024). Analyzing the diversity of MYB family response strategies to drought stress in different flax varieties based on transcriptome data. Plants 13, 710. doi: 10.3390/plants13050710
Zhao, L.-R., Li, W., Wang, L.-M., Qi, Y.-N., Li, W.-J., Xie, Y.-P., et al. (2023). Identification and relative expression pattern of PLA1 gene family in flax. Acta Agronomica Sin. 49, 2949–2965. doi: 10.3724/SP.J.1006.2023.24224
Keywords: flax, Linum usitatissimum, line 3896, linseed, transcriptome map, reference genome, genome annotation
Citation: Zhernova DA, Arkhipov AA, Rozhmina TA, Zhuchenko AA, Bolsheva NL, Sigova EA, Dvorianinova EM, Borkhert EV, Pushkova EN, Melnikova NV and Dmitriev AA (2025) Transcriptome map and genome annotation of flax line 3896. Front. Plant Sci. 16:1520832. doi: 10.3389/fpls.2025.1520832
Received: 31 October 2024; Accepted: 25 March 2025;
Published: 16 May 2025.
Edited by:
Changmian Ji, Chinese Academy of Tropical Agricultural Sciences, ChinaReviewed by:
Li Xinrui, Sichuan Agricultural University, ChinaJiban Mitra, Indian Council of Agricultural Research, India
Copyright © 2025 Zhernova, Arkhipov, Rozhmina, Zhuchenko, Bolsheva, Sigova, Dvorianinova, Borkhert, Pushkova, Melnikova and Dmitriev. This is an open-access article distributed under the terms of the Creative Commons Attribution License (CC BY). The use, distribution or reproduction in other forums is permitted, provided the original author(s) and the copyright owner(s) are credited and that the original publication in this journal is cited, in accordance with accepted academic practice. No use, distribution or reproduction is permitted which does not comply with these terms.
*Correspondence: Daiana A. Zhernova, emhlcm5vdmEuZEB5YS5ydQ==; Alexey A. Dmitriev, YWxleF8yNDVAbWFpbC5ydQ==